Do not forget to give thiamine to your septic shock patient!
Chemical properties and biochemical functions of thiamine
Thiamine was the first B vitamin to be identified and is also referred to as vitamin B1. It is a water-soluble vitamin that is an indispensable constituent of cellular metabolism (1). A lack of this vitamin can, therefore, be potentially life-threatening (2). Thiamine is present in the human body as free thiamine and as various phosphorylated forms: thiamine monophosphate, thiamine diphosphate, also known as thiamine pyrophosphate (TPP) (3,4), which is the most important and active form of this vitamin, and thiamine triphosphate. Thiamin pyrophosphate is an intracellular compound, which is why it can be considered as the best marker of thiamine nutritional status (1,4). It acts in concomitance with magnesium to expedite various mitochondrial oxidative decarboxylation reactions (Figure 1). Thiamin pyrophosphate is necessary as a cofactor for branched-chain ketoacid dehydrogenase complex essential for the metabolism of the branched-chain amino acids and for two critical complexes required for the mitochondrial synthesis of adenosine triphosphate (ATP): pyruvate and 2-oxoglutarate dehydrogenase (α-ketoglutarate) complexes. Specifically, TPP is a catalyst in the reactions of pyruvate to acetyl-Coenzyme A and α-ketoglutarate to succinyl-Coenzyme A in the Krebs cycle (Figure 1). Also, TPP serves as a coenzyme for transketolase, a cytosolic enzyme implicated in the pentose phosphate pathway, that functions in maintaining cell redox status through the production of NADPH (reduced nicotinamide adenine dinucleotide phosphate) and glutathione (5,6) (Figure 1). Thus, thiamine is an essential source of energy generation from glucose, via the conversion of pyruvate from glucose into acetyl-Coenzyme A for access to the tricarboxylic acid cycle (6), and lack of thiamine leads to alterations in intermediate metabolism that end-up in lactic acidosis (4).
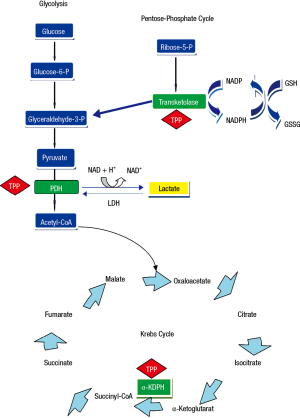
Thiamine absorption
Thiamine is found mainly in raw foods, but its content is relatively low (7). Thiamine is absorbed in the jejunum by two processes. At low thiamine level in the small intestines, an active transport that is likely carrier-mediated is responsible for absorption. When the thiamine concentration is high, absorption takes place by passive diffusion (7). Phosphorylation occurs in the jejunal mucosa to produce TPP. Maximum absorption of thiamine by the small intestines is 5 mg. Thiamine is conveyed by portal blood to the liver and transferred into red blood cells by a diffusion manner (7). The body cannot produce thiamine and can only store up to 30 mg of it with 80% as TPP and the remainder as thiamine monophosphate (1). About 50% of the body stores are found in the skeletal muscles with the rest in the heart, liver, kidney, and tissue of the nervous system, including the brain (3). Thiamine has a quick turnover rate, and therefore, it is difficult to maintain its store without continuous supplementation from food or other resources.
Thiamine elimination
Thiamine, in the surplus of storage quantity and tissue demand, is systemically eliminated by renal excretion (1,3). Under conditions of thiamine shortage, this vitamin falls off rapidly from all tissues excepting the brain. The diminution of TPP in red blood cells is approximately similar to the decline of this coenzyme in other tissues (1). In parallel to the thiamine deficiency development, urinary thiamine excretion drops nearby zero to maintain endogenous stocks. The half-life of thiamine is 9–18 days.
Associated thiamine insufficiency syndromes
Thiamine shortage can develop within two weeks of insufficient status (3). Lack of thiamine manifests in several particular signs and symptoms that are related to the impairments in the biochemistry of thiamine that result in an alteration in the oxidative phosphorylation, augmented levels of intermediate metabolites (pyruvate), and declined transketolase activity in red blood cells, liver, heart, and other organs (1,7).
Thiamine shortage displays as wet or dry beriberi. Wet beriberi or cardiac beriberi alters cardiac function and typically manifests as peripheral dilation with high-output cardiac failure, although variations can occur, including biventricular low-output failure with salt sodium and water retention mimicking the signs and symptoms of heart failure (6). A life-threatening form of thiamine deficiency is termed Shoshin beriberi. Although rare, it is a fulminant form of wet beriberi that can develop very quickly provoking acute cardiovascular collapse, severe lactic acidosis, and if no treatment is available death occurs within hours (8). However, recovery is usually fairly rapid and complete following intra-venous thiamine injection (8,9).
Thiamine deficiency with nervous system involvement is called dry beriberi (10). The neuropathic beriberi is characterized by symmetric alterations of sensory, motor, and reflex functions that typically affects more the lower extremities than the upper extremities and is often painful. Neuropathic beriberi can be permanent even following thiamine repletion (11,12). Another presentation of neurologic involvement is Wernicke-Korsakoff syndrome that classically presents with the clinical triad of global confusion, ataxia, and oculomotor abnormalities, in which nystagmus is the most common abnormality (10,11). However, all these symptoms do not need to be present to make the diagnosis of Wernicke-Korsakoff syndrome (10).
Thiamine deficiency in the critically ill patients
Predisposition to thiamine shortage can come from many situations that are associated with nutritional compromise and co-morbid disease. As thiamine is closely linked to carbohydrate metabolism, increased metabolic demand, parenteral or enteral nutrition, diuretics, hemodiafiltration could all deplete thiamine levels further. Several studies have found the presence of thiamine deficiency in critically ill patients (13-17). In some of these studies, thiamine deficiency was associated with poor outcome (13,14), whereas in others, this relationship was not observed (16,17). The discrepancy between these studies may be explained by different study designs, different patient population, and different methods used to measure blood thiamine levels (erythrocyte transketolase or direct measurement).
Thiamine deficiency is also prevalent in septic shock patients, with rates ranging from 20% to 70% depending on the cutoff value used to define the presence of thiamine deficiency (17-19). Lack of thiamine reduces the flux of pyruvate to the Krebs cycle, thus increasing lactate production by altering the aerobic metabolism (Figure 1). In a prospective, observational study, Donnino et al. (18) investigated the relationship between thiamine levels and lactic acidosis in 30 septic shock patients, and found no correlation between these two variables. However, after excluding patients with abnormal liver function tests, the authors observed a significant negative correlation between thiamine concentrations and lactic acidosis (r=−0.53, P=0.01), implying a potential association between thiamine levels and lactic acidosis in septic shock patients with normal liver function (18). Thus, the possibility that by decreasing the activity of pyruvate dehydrogenase complex, thiamine deficiency can contribute to increased production of lactic acid in critically ill septic patients exists.
Recently, the same authors, in a two-center, randomized, double-blind trial, evaluated if the administration of thiamine in patients with septic shock would result in a reduction in lactate levels (19). Adult patients with the classic criteria of septic shock associated with lactate levels >3 mmol/L were included in this study. Exclusion criteria included patients with liver dysfunction, alcohol abuse, and current thiamine supplementation. Eighty-eight patients were randomized to thiamine or placebo in a 1:1 ratio. Patients were given 200 mg thiamine or matching placebo twice daily for seven days or until hospital discharge. Importantly, placebo was the same in appearance to thiamine and patients, physicians, and the research team all were stayed blinded during the study period. Plasma thiamine and lactate levels were determined at enrollment just before the administration of thiamine and at 6, 12 and 24 hours after that. In this interesting study, thiamine levels were directly measured in plasma via Liquid Chromatography technique, and thiamine deficiency was defined as plasma level of 7 nmol/L or less. The primary outcome was the absolute difference in lactate levels 24 hours after the first administration dose of either thiamine or placebo, even though the sample size was calculated based on the relative rather than the absolute changes in lactate levels. Baseline characteristics were well equilibrated between thiamine and placebo groups (19).
The authors were not able to observe a difference in the primary outcome of lactate concentrations at 24 hours between thiamine and placebo groups [median: 2.5 (1.5–3.4) vs. 2.6 (1.6–5.1) mmol/L, P=0.4; respectively]. The decrease in lactate levels from baseline to 24 hours was higher with significantly lower lactate concentrations in the thiamine group at 24 hours (P=0.048). However, all the pair-wise tests that were performed were without adjustment for multiple comparisons (four comparisons), with the consequence of family-wise error inflation. Thus, this analysis should be considered as exploratory. Moreover, no significant differences in the proportion and time to shock reversal, SOFA score at 24 hours, mortality rates, and ICU and length of stay were found between the thiamine and placebo groups (19). These findings are not a surprise since we do not expect that the administration of thiamine will have outstanding effects in sepsis with a myriad of biochemical abnormalities and multiple underlying pathophysiologic mechanisms.
Among septic shock patients with thiamine deficiency (n=28), the authors found that patients in the thiamine group (n=15) had lower lactate concentrations at 24 hours compared with the placebo group [median: 2.1 (1.4–2.5) vs. 3.1 (1.9–8.3) mmol/L, P=0.03; respectively]. Also, only 7% of patients in the thiamine group had lactate concentration ≥4 mmol/L compared to 38% in the placebo group (P=0.07). The in-hospital mortality was higher in the placebo group, but it was not statistically significant (46% vs. 13%, P=0.1). However, the authors observed a statistically significant difference in time to death in favor of thiamine group (Log-rank test, P=0.047) (19). All these results suggest that the administration of thiamine is advantageous in septic shock patients with severe thiamine deficiency (thiamine level ≤7 nmol/L). Even though the authors a priori planned to analyze the sub-group of patients with thiamine deficiency, these findings should be taken with precautions for several reasons. First, in this sub-group, patients were no longer randomly allocated to receive thiamine or placebo dose resulting in a lack of comparability of the two groups at baseline, and therefore, the effects of thiamine on outcomes are influenced by the potential issues of confounding. Seconds, the authors did not provide any information about the management of septic shock patients. Any differences between the two groups in the way that patients were resuscitated (fluid management, transfusion, antibiotics, etc.) could have a substantial impact on the results. Third, it is not shown whether the administration of thiamine has led to a significant increase in plasma thiamine levels at 24 hours. Fourth, the sample size for the thiamine deficiency group was minuscule preventing any conclusion. Fifth, among the 88 patients included in this study, only 8 (9%) patients were enrolled in the non-coordinating center (19), which means that this is a mainly monocenter study precluding then the generalizability of the results.
Increased lactate levels in septic shock may result from different mechanisms. Inadequacy between oxygen delivery and oxygen demand by the tissues, impairment of tissue oxygen extraction due to the sepsis-induced alterations in the microcirculation, and mitochondrial dysfunction all lead to the activation of anaerobic metabolism, and pyruvate is shunted toward lactate production (20). Other non-hypoxic mechanisms such as accelerated aerobic glycolysis induced by sepsis-associated inflammation (20), increased Na+-K+-ATPase activity through β2 stimulation (21), sepsis-induced inhibition of pyruvate dehydrogenase complex (22), impaired lactate clearance (23), and may be thiamine deficiency (18) may contribute to hyperlactatemia in septic shock patients. However, the source of lactic acidosis in septic shock patients is not usually identified by ICU physicians at the bedside. Also, in practice, measurements of plasma thiamine levels are not widely available laboratory tests and take several days to perform and, therefore, will not aid in decision making in septic shock. Nevertheless, septic shock can have manifestations similar to Shoshin beriberi and as adverse effects of thiamine administration are uncommon at both low or high dose and the overall safety profile (10), we think that septic shock patients should be given thiamine parenterally without waiting for the results of thiamine level. The European Society for Clinical Nutrition and Metabolism guidelines for parenteral nutrition in intensive care recommended thiamine supplementation of 100 to 300 mg/day during the first three days in the ICU for all patients with suspected thiamine deficiency (24). However, a dosage upwards to 500 mg may be necessary for patients with septic shock. Because anaphylaxis has been reported in rare instances (10), guidelines in the United Kingdom have recommended that thiamine should be administered over 15- to 30-minute interval in a mixture of saline solution or dextrose, with the intention of averting potential adverse reactions.
In conclusion, the authors should be congratulated for this fascinating and original study that shed the light on the possible beneficial effects of thiamine treatment in septic shock patients.
Acknowledgements
None.
Footnote
Provenance: This is an invited article commissioned by the Section Editor Zhongheng Zhang (Department of Critical Care Medicine, Jinhua Municipal Central Hospital, Jinhua Hospital of Zhejiang University, Jinhua, China).
Conflicts of Interest: The authors have no conflicts of interest to declare.
References
- Wooley JA. Characteristics of thiamin and its relevance to the management of heart failure. Nutr Clin Pract 2008;23:487-93. [Crossref] [PubMed]
- Donnino M. Gastrointestinal beriberi: a previously unrecognized syndrome. Ann Intern Med 2004;141:898-9. [Crossref] [PubMed]
- Sica DA. Loop diuretic therapy, thiamine balance, and heart failure. Congest Heart Fail 2007;13:244-7. [Crossref] [PubMed]
- Manzanares W, Hardy G. Thiamine supplementation in the critically ill. Curr Opin Clin Nutr Metab Care 2011;14:610-7. [Crossref] [PubMed]
- Gangolf M, Czerniecki J, Radermecker M, et al. Thiamine status in humans and content of phosphorylated thiamine derivatives in biopsies and cultured cells. PLoS One 2010;5:e13616. [Crossref] [PubMed]
- Depeint F, Bruce WR, Shangari N, et al. Mitochondrial function and toxicity: role of the B vitamin family on mitochondrial energy metabolism. Chem Biol Interact 2006;163:94-112. [Crossref] [PubMed]
- Gubler CJ. Thiamin. In: Machlin LJ. editor. Handbook of Vitamins. New York, NY: Marcel Dekker, 1991:233-81.
- Dabar G, Harmouche C, Habr B, et al. Shoshin Beriberi in Critically-Ill patients: case series. Nutr J 2015;14:51. [Crossref] [PubMed]
- Smithline HA. Thiamine for the treatment of acute decompensated heart failure. Am J Emerg Med 2007;25:124-6. [Crossref] [PubMed]
- Donnino MW, Vega J, Miller J, et al. Myths and misconceptions of Wernicke's encephalopathy: what every emergency physician should know. Ann Emerg Med 2007;50:715-21. [Crossref] [PubMed]
- Munir A, Hussain SA, Sondhi D, et al. Wernicke's encephalopathy in a non-alcoholic man: case report and brief review. Mt Sinai J Med 2001;68:216-8. [PubMed]
- Francini-Pesenti F, Brocadello F, Famengo S, et al. Wernicke's encephalopathy during parenteral nutrition. JPEN J Parenter Enteral Nutr 2007;31:69-71. [Crossref] [PubMed]
- Cruickshank AM, Telfer AB, Shenkin A. Thiamine deficiency in the critically ill. Intensive Care Med 1988;14:384-7. [Crossref] [PubMed]
- Lima LF, Leite HP, Taddei JA. Low blood thiamine concentrations in children upon admission to the intensive care unit: risk factors and prognostic significance. Am J Clin Nutr 2011;93:57-61. [Crossref] [PubMed]
- Seear M, Lockitch G, Jacobson B, et al. Thiamine, riboflavin, and pyridoxine deficiencies in a population of critically ill children. J Pediatr 1992;121:533-8. [Crossref] [PubMed]
- Corcoran TB, O'Neill MA, Webb SA, et al. Prevalence of vitamin deficiencies on admission: relationship to hospital mortality in critically ill patients. Anaesth Intensive Care 2009;37:254-60. [PubMed]
- Costa NA, Gut AL, de Souza Dorna M, et al. Serum thiamine concentration and oxidative stress as predictors of mortality in patients with septic shock. J Crit Care 2014;29:249-52. [Crossref] [PubMed]
- Donnino MW, Carney E, Cocchi MN, et al. Thiamine deficiency in critically ill patients with sepsis. J Crit Care 2010;25:576-81. [Crossref] [PubMed]
- Donnino MW, Andersen LW, Chase M, et al. Randomized, Double-Blind, Placebo-Controlled Trial of Thiamine as a Metabolic Resuscitator in Septic Shock: A Pilot Study. Crit Care Med 2016;44:360-7. [Crossref] [PubMed]
- Suetrong B, Walley KR. Lactic Acidosis in Sepsis: It's Not All Anaerobic: Implications for Diagnosis and Management. Chest 2016;149:252-61. [Crossref] [PubMed]
- Levy B, Gibot S, Franck P, et al. Relation between muscle Na+K+ ATPase activity and raised lactate concentrations in septic shock: a prospective study. Lancet 2005;365:871-5. Erratum in: Lancet 2005;366:122. [Crossref] [PubMed]
- Vary TC, Siegel JH, Nakatani T, et al. Effect of sepsis on activity of pyruvate dehydrogenase complex in skeletal muscle and liver. Am J Physiol 1986;250:E634-40. [PubMed]
- Levraut J, Ciebiera JP, Chave S, et al. Mild hyperlactatemia in stable septic patients is due to impaired lactate clearance rather than overproduction. Am J Respir Crit Care Med 1998;157:1021-6. [Crossref] [PubMed]
- Singer P, Berger MM, Van den Berghe G, et al. ESPEN Guidelines on Parenteral Nutrition: intensive care. Clin Nutr 2009;28:387-400. [Crossref] [PubMed]