Pneumonitis in advanced non-small cell lung cancer: no interaction between immune checkpoint inhibition and radiation therapy
Highlight box
Key findings
• In this population-based retrospective cohort study, there was no interaction between immune checkpoint inhibition and radiation therapy in causing pneumonitis.
What is known and what is new?
• Radiation therapy and immune checkpoint inhibition are common causes of pneumonitis in patients with non-small cell lung cancer.
• The risk of pneumonitis for patients treated with both therapies is no more than could be attributed by each therapy alone (i.e., no synergy between radiation therapy and immune checkpoint inhibition).
What is the implication, and what should change now?
• These data support the safety of treating patients with radiation therapy and immune checkpoint inhibition in routine clinical practice.
• Immunotherapy-radiation combinations can be explored to improve patient outcomes without exposing patients to excess toxicity.
Introduction
Pneumonitis is a common complication of treatment for advanced non-small cell lung cancer (NSCLC) (1-13). Often labeled by its presumed precipitant, pneumonitis can be caused by radiation (radiation pneumonitis), immunotherapy (immune-related pneumonitis), and other systemic agents (14). Radiation and immune-related pneumonitis have been studied independently, but little information has emerged describing the interactions between radiation therapy (RT) and immune checkpoint inhibition (ICI).
Studying the interactions between ICI and RT has become increasingly important as more patients are treated with immunotherapy since the first approvals for NSCLC in 2015 (15,16). With prolonged survivals owing to improvements in systemic therapies such as ICI, the incorporation of local therapies such as RT may become increasingly relevant to control symptoms or address gross disease (17). Patients may be treated with palliative RT to a thoracic mass and then start treatment with ICI to manage systemic disease, or they might be treated with definitive RT to a locally advanced mass and then start adjuvant ICI (15). Additionally, there is greater utilization of RT to oligometastatic patients before initiating ICI or after a robust response to ICI (18-23). Understanding how RT and ICI interact is imperative to safely escalate treatment.
While there is growing interest in combining RT and ICI to leverage immune-mediated effects of RT against tumor cells, there is an equal and opposite concern about the overlapping toxicities of these two critical modalities. Local tissue damage from radiation can prime an immune response against self-antigens prior to ICI initiation or restimulate immune-mediated toxicity after ICI treatment (24,25). This biological interplay between RT and ICI has been explored, but a definitive, clinically relevant interaction has not been established (26). Simply observing higher than expected rates of pneumonitis in patients treated with RT and ICI does not imply a true synergistic effect (13,27). An interaction between RT and ICI in causing pneumonitis requires that the risk of pneumonitis from both therapies is higher than the contribution of each therapy alone (28).
To address this clinical hypothesis, we performed a retrospective cohort study of patients with NSCLC treated with RT and ICI. We present this article in accordance with the STROBE reporting checklist (available at https://jtd.amegroups.com/article/view/10.21037/jtd-22-1649/rc).
Methods
Study design and population
A retrospective cohort study was performed comparing patients receiving RT, ICI, RT and ICI, and neither RT nor ICI, using the Surveillance, Epidemiology, and End Results-Medicare database (29). All patients over the age of 65 diagnosed from 2013–2017 with American Joint Committee on Cancer 7th edition (AJCC) stages IIIB–IV NSCLC were included. Patients were excluded if they were not enrolled in Medicare parts A and B during the 12 months before their lung cancer diagnosis or were enrolled in an health maintenance organization (HMO) within 12 months after diagnosis. These exclusions were made to ensure that all treatments and toxicities would be evaluable in the dataset.
Each patient’s index date was their first diagnosis with NSCLC (Figure S1). An exposure assessment window was defined as the 12 months after the index date; first exposures to RT and ICI were only measured during this period. A double-exposure assessment window was defined as the 3 months after the first exposure to either RT or ICI. If a second exposure (e.g., ICI after RT) occurred within this window, then the patient was allocated to the RT + ICI group. The control arm was assembled by randomly matching all patients not treated with RT or ICI to a treated patient diagnosed in the same three-month period (e.g., January–March 2013).
An outcome assessment window (OAW) was defined as the 6 months after the first RT or ICI exposure (for RT and ICI groups), or after the second exposure (for the RT + ICI group), to evaluate for cases of pneumonitis. The control patients were assigned an OAW to be the same as that of their respective match. All patients who had pneumonitis before the OAW were removed from the analysis.
Measures
A single Healthcare Common Procedure Coding System (HCPCS) code in the outpatient or carrier data defined a patient as exposed to that therapy (Table S1). RT claims with a primary diagnosis code of 1983, 1984, C7931, or C7932 (secondary brain metastases) associated with RT claims were excluded to enrich for thoracic RT.
Development of pneumonitis was determined by implementing a validated algorithm for identifying pneumonitis in inpatient and outpatient claims data (30). This algorithm was demonstrated to have a positive predictive value of 86.9% for identifying pneumonitis. The definition included a list of 11 ICD-10-CM codes, which were then converted to ICD-9-CM with lookup tables (Table S2).
Relevant covariates were measured prior to diagnosis and at the time of diagnosis. AJCC Stage, presence of brain metastases, age, squamous histology, and sex were determined at the time of diagnosis. History of chronic obstructive pulmonary disease (COPD) was determined by evaluating ICD codes in outpatient and inpatient claims prior to lung cancer diagnosis (Table S3).
Sensitivity analyses were performed to test whether adjusting key assumptions altered the interaction estimate. The exposure and outcome assessment windows were extended and shortened, thus adjusting the available time to identify treatments codes and pneumonitis diagnoses. Another sensitivity analysis analyzed exposure to RT and ICI as a time-varying covariate (31). To test if treatment sequencing impacted the interaction between RT and ICI, two analyses were performed only including those treated with RT before ICI in the RT + ICI group and vice versa. Additional sensitivity analyses were performed applying more stringent criteria for defining exposure to RT, to enrich for patients receiving thoracic RT: 15 fractions of conventional RT, 5 fractions of stereotactic body radiation therapy, or just one claim for intensity modulatory radiation therapy or proton therapy were required. Analogously, one sub-analysis required exposure to nivolumab and pembrolizumab (PD1 inhibitors) to be counted as exposed to ICI (removing exposure to PDL1 and CTLA4 inhibitors). Other sensitivity analyses were performed altering the inclusion criteria (stages IIIA–IIIC or only stage IV NSCLC), implementing three distinct algorithms for identifying pneumonitis (including only measuring pneumonitis cases that resulted in steroid prescription), and only analyzing inpatient pneumonitis diagnoses (30).
Statistical analysis
Baseline characteristics of patients in each treatment group (control, RT, ICI, RT + ICI) were compared with proportions and Chi-squared tests for categorical variables and medians and Wilcoxon rank-sum tests for continuous variables. Pneumonitis-free survival was computed using the Kaplan-Meier method, censoring patients at the end of the outcome assessment window or death. Hazard ratios were computed using Cox regression in unadjusted and adjusted models. Adjusted models included age at diagnosis, sex, AJCC stage, histology (squamous vs. non-squamous), history of COPD, and presence of brain metastases at diagnosis. These covariates were determined a priori, from multidisciplinary discussion of potential confounders that would impact treatment allocation and development of pneumonitis.
To accomplish the primary aim of the study, evaluating for the presence of an interaction between RT and ICI, the relative excess risk due to interaction (RERI) was quantified. The RERI was chosen as the primary outcome a priori because it quantifies whether the risks of pneumonitis are additively higher in the RT + ICI group than could be attributed to each individual treatment alone (32). The RERI is considered a more biologically relevant measure of interaction than the conventionally used interaction coefficient from a statistical model (33). RERI was calculated using the coefficients from Cox regression as previously described (34). Ninety-five percent confidence intervals (95% CI) of RERI were constructed using bootstrapping. The primary endpoint would be met if the lower bound of the 95% CI were greater than 0, and a one-tailed t-test was used to calculate P values (35). With an alpha cut-off of 0.05, 80% power, a baseline risk of pneumonitis of 1%, 40% of patients in the control group, 40% of patients in the RT group, 15% of patients in the ICI group, and 5% of patients in the RT + ICI group, 5,600 patients are needed to detect a risk ratio of 2.5, where the risk of pneumonitis is 2 times higher for those who were exposed to RT and ICI alone relative to controls.
All analyses were conducted using SAS 9.4 (SAS Institute, Cary, NC, USA). The study was conducted in accordance with the Declaration of Helsinki (as revised in 2013). This study was approved by the Rutgers Biomedical and Health Sciences Institutional Review Board (Pro2020002649) and individual consent for this retrospective analysis was waived.
Results
A total of 42,915 patients with AJCC Stage IIIB-IV NSCLC were identified (Figure 1). Excluded from the analysis were 3,625 (8.4%) patients who were not enrolled in Medicare Parts A and B in the 12 months prior to diagnosis and 14,879 (34.7%) patients who were enrolled in an HMO within12 months of their diagnosis. Of the remaining 24,411 patients, 99 (0.4%) patients were removed because of a pneumonitis diagnosis before lung cancer diagnosis, and 55 (0.2%) patients were removed because of treatment with RT or ICI before confirmed lung cancer diagnoses. In total, 24,257 patients were analyzed: 7,553 patients were treated with RT, 1,332 patients were treated with ICI, and 550 patients were treated with RT + ICI. Of the remaining 14,882 patients who were not exposed to RT or ICI, 5,537 (37.2%) were excluded after matching because they died or developed pneumonitis before the assigned outcome assessment window, leaving 9,345 patients to be analyzed as controls.
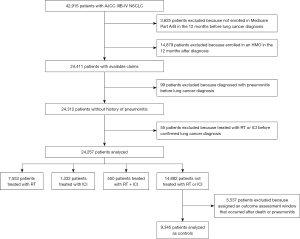
Most patients included in the analysis were ages 65–76 years (54.1%), male (51.8%), and White (83.5%). The baseline characteristics of this patient population are summarized in Table 1. Notably, 12.6% of patients in the control group were over 85 years-old, compared to 6.6%, 7.5%, and 3.3% in the RT, ICI, and RT + ICI groups, respectively (P<0.01). There were also more female and Black patients in the controls than any of the treatment groups (P<0.01). Expectedly, most patients who were exposed to ICI were diagnosed in 2015 or later (P<0.01). While the RT group had more patients with squamous histology and AJCC stage 3B disease than the other groups, the ICI and RT + ICI groups had higher frequencies of patients with a history of COPD (P<0.01). Also, the RT and RT + ICI group had higher frequencies of patients with brain metastases at the time of lung cancer diagnosis (P<0.01).
Table 1
Variables | Patient, n (%) | P value | |||
---|---|---|---|---|---|
RT n=7,553 (40.2%) | ICI n=1,332 (7.1%) | RT + ICI n=550 (2.9%) | Control n=9,345 (49.8%) | ||
Age (years) | <0.01 | ||||
66–75 | 4,528 (60.0) | 752 (56.5) | 342 (62.2) | 4,539 (48.6) | |
76–85 | 2,526 (33.4) | 480 (36.0) | 190 (34.6) | 3,632 (38.9) | |
86+ | 499 (6.6) | 100 (7.5) | 18 (3.3) | 1,174 (12.6) | |
Sex | <0.01 | ||||
Male | 4,011 (53.1) | 682 (51.2) | 300 (54.6) | 4,742 (50.7) | |
Female | 3,542 (46.9) | 650 (48.8) | 250 (45.4) | 4,603 (49.3) | |
Race | <0.01 | ||||
White | 6,412 (84.9) | 1,140 (85.6) | 482 (87.6) | 7,637 (81.7) | |
Black | 689 (9.1) | 94 (7.1) | 28 (5.1) | 910 (9.7) | |
AI | ** | ** | ** | 54 (0.6) | |
API | 417 (5.5) | 88 (6.6) | 38 (6.9) | 731 (7.82) | |
Unknown | ** | ** | ** | 13 (0.1) | |
Year of diagnosis | <0.01 | ||||
2013 | 1,736 (23.0) | 0 (0.0) | ** | 2,256 (24.1) | |
2014 | 1,709 (22.6) | 21 (1.6) | ** | 2,255 (24.1) | |
2015 | 1,620 (21.5) | 232 (17.4) | 70 (12.7) | 1,863 (19.9) | |
2016 | 1,376 (18.2) | 393 (29.5) | 174 (31.6) | 1,617 (17.3) | |
2017 | 1112 (14.7) | 686 (51.5) | 305 (55.5) | 1,354 (14.5) | |
Histology | <0.01 | ||||
Squamous | 2,336 (30.9) | 304 (22.8) | 136 (24.7) | 1,902 (20.4) | |
Non-squamous | 5,217 (69.1) | 1,028 (77.2) | 414 (75.3) | 7,443 (79.7) | |
AJCC Stage | <0.01 | ||||
3B | 1,487 (19.7) | 85 (6.4) | 32 (5.8) | 682 (7.3) | |
4 | 6,066 (80.3) | 1,247 (93.6) | 518 (94.2) | 8,663 (92.7) | |
Comorbidities | |||||
COPD | 2,515 (33.3) | 555 (41.7) | 220 (40.0) | 3,142 (33.6) | <0.01 |
Brain metastases | 1,738 (23.0) | 212 (15.9) | 119 (21.6) | 1,494 (16.0) | <0.01 |
**, suppressed cells to preserve anonymity in line with Centers for Medicare and Medicaid Services Policy. RT, radiation therapy; ICI, immune checkpoint inhibition; AI, American Indian/Alaskan Native; API, Asian or Pacific Islander; AJCC, American Joint Commission on Cancer; COPD, chronic obstructive pulmonary disease; brain metastases, identification of brain metastases at lung cancer diagnosis.
There were significant differences in the type of ICI utilized in the RT + ICI and ICI groups (P<0.01). There were more patients treated with PDL1 inhibitors (atezolizumab, durvalumab) in the RT + ICI group (5.3%) than in the ICI group (3.0%). Treatment with CTLA4 inhibition (ipilimumab) was rare in this cohort; there were <11 patients in the ICI group (<1.0%) treated with ipilimumab compared to no patients in the RT + ICI group.
Pneumonitis events, frequencies, and hazard ratios are presented in Table 2. There were 28 (0.3%), 296 (3.9%), 33 (2.5%), and 19 (3.5%) diagnoses of pneumonitis in the control, RT, ICI, and RT + ICI groups, respectively (P<0.01). Relative to controls, these events corresponded to hazard ratios of 11.5 (95% CI: 7.9–17.0), 6.2 (95% CI: 3.8–10.3), and 10.7 (95% CI: 6.0–19.2) in the RT, ICI, and RT-ICI groups, respectively. A Kaplan-Meier curve depicting freedom from pneumonitis is presented in Figure 2; there was a median follow up of 139 days (right censored at 180 days). After adjusting for age at diagnosis, sex, histology (squamous vs. non-squamous), AJCC stage, brain metastases at diagnosis, and a history of COPD, the hazard ratio estimates remained consistent. There was no evidence of interaction between RT and ICI in the unadjusted (RERI =−6.1 (95% CI: −13.1 to −0.6), P=0.89) and adjusted analyses (RERI =−4.0 (95% CI: −10.7 to 1.5), P=0.91). While there was no evidence of interaction, the adjusted hazard ratios of pneumonitis for RT + ICI relative to RT and ICI were 1.1 (95% CI: 0.7–1.8, P=0.64) and 1.7 (95% CI: 1.0–3.0, P=0.06), respectively.
Table 2
Treatment | Events/Total | Frequencies (95% CI) |
Hazard ratio (95% CI) | RERI (95% CI), P value* | |||
---|---|---|---|---|---|---|---|
Unadjusted | Adjusted | Unadjusted | Adjusted | ||||
Control | 28/9,345 | 0.3% (0.2, 0.4) | Reference | Reference | – | – | |
RT | 296/7,553 | 3.9% (3.5, 4.4) | 11.5 (7.9, 17.0) | 9.5 (6.4, 14.0) | – | – | |
ICI | 33/1,332 | 2.5% (1.8, 3.5) | 6.2 (3.8, 10.3) | 6.2 (3.7, 10.2) | – | – | |
RT + ICI | 19/550 | 3.5% (2.2, 5.4) | 10.7 (6.0, 19.2) | 10.6 (5.9, 19.0) | −6.1 (−13.1, −0.6), P=0.89 | −4.0 (−10.7, 1.5), P=0.91 |
Adjusted models control for age at diagnosis, sex, histology, AJCC stage, brain metastases at diagnosis, and history of chronic obstructive pulmonary disease. *, hypothesis testing for RERI compared estimate to a null hypothesis of RERI ≤0. RT, radiation therapy; ICI, immune checkpoint inhibition; RERI, relative excess risk due to interaction; 95% CI, 95% confidence interval; AJCC, American Joint Commission on Cancer.
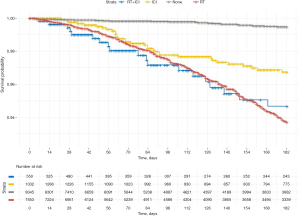
Multiple sensitivity analyses were performed to demonstrate the robustness of the estimate of no interaction between RT and ICI in causing pneumonitis (Table S4). When adjusting the exposure, double-exposure, and outcome assessment windows, and analyzing RT and ICI as time-varying covariates there was still no evidence of interaction. Similarly, when adjusting the definitions of RT and ICI exposure, definition of pneumonitis, and inclusion criteria (AJCC stage), there was no evidence of interaction. Of note, when analyzing RT and ICI as time-varying covariates, the RERI approached 0, indicating pure additivity of the hazards of pneumonitis from RT and ICI, i.e., no synergy or antagonism.
Discussion
This study is the first to our knowledge to examine rigorously the presence of an interaction between RT and ICI in causing pneumonitis in patients with advanced NSCLC. We found no evidence of an interaction between RT and ICI in our primary analysis and various sensitivity analyses. While clinicians may observe a higher risk of pneumonitis in patients receiving RT and ICI, concurrently or in series, our analysis suggests that these risks are no more than could be expected from the contribution of each therapy alone.
Understanding if RT and ICI interact in causing pneumonitis is of major clinical relevance. First, many patients with metastatic disease will receive RT to the primary tumor in the weeks surrounding initiation of ICI. Because most of the survival benefits in Stage IV disease are secondary to ICI initiation and maintenance, a synergism between RT and ICI in causing pneumonitis would discourage utilization of RT. Similarly, in the setting of locally advanced disease, where patients are treated with definitive chemoradiation, a synergism between RT and ICI, would reduce the real-world efficacy of this approach especially in patients at higher risk of pneumonitis at baseline (36). The lack of synergism between RT and ICI in our study suggests that the risks of initiating RT and ICI treatment can be considered independently.
The theoretical risks of combining RT and ICI are founded in a reasonable biological rationale. RT and ICI are both known to cause pneumonitis and their mechanisms could plausibly be synergistic. RT may result in an in situ vaccination effect, which could be leveraged to mount an anti-tumor immune response, but also deleterious chronic inflammation (24,37-40). When combined with ICI, a disinhibited immune system may cause increased cytokine and cell-dependent toxicity, thus promoting pneumonitis (41-43). However, our study provided no evidence of synergy, potentially indicating that this pre-clinical evidence is not translatable to clinical practice.
Beyond the biological plausibility of the risks of pneumonitis, investigators have raised concerns about combining RT and ICI after pivotal clinical trials. The PACIFIC trial was one of the first studies to discuss this effect. The investigators found an increased risk of any-grade pneumonitis in patients receiving consolidative ICI after definitive RT vs. the placebo arm (33.9% vs. 24.8%) (13). These results are not at odds with the findings from our study. In the sub-analysis of patients with Stage III disease (Table S4), we found an insignificantly higher risk of pneumonitis in the RT + ICI group than the RT group and no evidence of interaction (RERI =2.2, P=0.50). Our results in the context of the PACIFIC trial support that risks of pneumonitis are higher in patients receiving both RT and ICI, but these higher risks are not supra-additive (synergistic). That being said, it might be premature to make claims about the locally advanced NSCLC population, because the PACIFIC regimen was not widely used before the end of the study period. A future investigator may want to repeat this analysis once more patients with Stage III disease are treated with RT and ICI.
Another study of clinical trial data submitted to the Federal Drug Administration some evidence of increased risks of pneumonitis in patients receiving RT within 90 days of ICI (27). They found a 6.8% risk of any grade pneumonitis in patients receiving RT within 90 days of ICI compared to 3.6% risk in patients receiving RT >90 days after ICI. While this study did not measure interaction explicitly, these results were consistent with our primary analysis. We found that there was an insignificantly higher risk of pneumonitis in patients receiving RT and ICI compared to ICI alone (HR =1.7, 95% CI: 1.0–3.0). Like the PACIFIC trial findings, higher risk of pneumonitis is not unexpected, and does not imply synergy.
Limitations
Our study is not without limitations. First, this study was a retrospective analysis of routinely collected administrative data. Without the benefits of randomization, confounding by indication may exist, limiting the interpretability of our interaction estimates. There are likely meaningful differences between patients allocated to each treatment group. While we did control for important measured covariates in multivariable Cox regression, residual confounding could exist.
Another weakness of using administrative data is the limited information about the dose, fractionation, and treatment site of RT. It is possible that a higher proportion of patients in the RT group received definitive doses of radiation to the primary tumor than patients in the RT + ICI group. Higher definitive doses in the RT group could have led to inflated estimates of the hazards of pneumonitis and bias the estimate of interaction towards the null. Although this effect was explored in a sensitivity analysis by applying a stricter definition of RT, residual confounding may exist.
Similarly, assessing pneumonitis in claims data has the risk of misclassification. To mitigate this risk, we used a validated definition of pneumonitis for our primary analysis that had a reported positive predictive value of 86.9% and conducted the analysis using three other validated definitions (30). Because these algorithms do not necessarily identify only higher grade, clinically relevant pneumonitis, we also conducted a sensitivity analysis only analyzing diagnosis codes from hospital admissions. Although, we found differing estimates of the risks of pneumonitis in each algorithm used, we consistently detected no evidence of interaction.
While our analysis found no interaction between RT and ICI in causing pneumonitis, this null finding may be the result of the treatment patterns and patient characteristics between 2012-2018. Since 2018, radiation and immunotherapy have been applied in other clinical contexts and use has been expanding to more patients who were untreated during our study period. Also, our analysis only included patients ages 65 or greater; the Medicare-eligible population might not receive the most aggressive RT and ICI treatment. Thus, the generalizability of our study may be limited, and synergism between RT and ICI may need to be examined again after updates to the SEER-Medicare database.
Finally, our primary analysis was hinged on establishing discrete exposure, double exposure, and outcome assessment windows. These windows were established a priori based on clinical judgment but could have impacted the results. We mitigated this risk by conducting numerous sensitivity analyses adjusting these windows, and the estimate of interaction did not significantly change. Similarly, a model of time-varying exposure—which allows patients to move between strata upon RT or ICI exposure—showed no evidence of interaction.
Conclusions
In this retrospective analysis of data of Medicare beneficiaries diagnosed with AJCC IIIB-IV NSCLC, there was no evidence of interaction between RT and ICI in causing pneumonitis. At most, it appears that pneumonitis risk from RT and ICI is additive, but not synergistic. The risk of pneumonitis in patients treated with RT and ICI is not greater than could be expected from the contribution of each therapy alone. These results support the safety of combining RT and ICI in patients with advanced NSCLC.
Acknowledgments
Funding: This study was supported by the Radiological Society of North American Research and Education Foundation Medical Student Grant, award number RMS2126 (SSN). Research reported in this publication was supported by the National Center for Advancing Translational Sciences (NCATS), a component of the National Institutes of Health (NIH) under award number UL1TR003017 (SSN). The content is solely the responsibility of the authors and does not necessarily represent the official views of the National Institutes of Health. This study was also supported in part by the Chancellor’s Global Health Scholarship (SSN).
Footnote
Reporting Checklist: The authors have completed the STROBE reporting checklist. Available at https://jtd.amegroups.com/article/view/10.21037/jtd-22-1649/rc
Peer Review File: Available at https://jtd.amegroups.com/article/view/10.21037/jtd-22-1649/prf
Conflicts of Interest: All authors have completed the ICMJE uniform disclosure form (available at https://jtd.amegroups.com/article/view/10.21037/jtd-22-1649/coif). SKJ reports grants and contracts from Merck & Co, and consulting fees from Merck & Co and IMX Medical. JM reports grants and contracts Bristol Myers-Squibb, Celldex, Daiichi Sankyo, and Beyond Spring. SSN reports RSNA R&E Foundation Medical Student Grant, Chancellor’s Global Health Scholarship, the National Center for Advancing Translational Sciences Fellowship UL1, Consulting fees from Hibiscus BioTechnology, and Novocure travel grant. The other authors have no conflicts of interest to declare.
Ethical Statement: The authors are accountable for all aspects of the work in ensuring that questions related to the accuracy or integrity of any part of the work are appropriately investigated and resolved. The study was conducted in accordance with the Declaration of Helsinki (as revised in 2013). This study was approved by the Rutgers Biomedical and Health Sciences Institutional Review Board (Pro2020002649) and individual consent for this retrospective analysis was waived.
Open Access Statement: This is an Open Access article distributed in accordance with the Creative Commons Attribution-NonCommercial-NoDerivs 4.0 International License (CC BY-NC-ND 4.0), which permits the non-commercial replication and distribution of the article with the strict proviso that no changes or edits are made and the original work is properly cited (including links to both the formal publication through the relevant DOI and the license). See: https://creativecommons.org/licenses/by-nc-nd/4.0/.
References
- Suresh K, Voong KR, Shankar B, et al. Pneumonitis in Non-Small Cell Lung Cancer Patients Receiving Immune Checkpoint Immunotherapy: Incidence and Risk Factors. J Thorac Oncol 2018;13:1930-9. [Crossref] [PubMed]
- Tyczynski JE, Potluri R, Kilpatrick R, et al. Incidence and Risk Factors of Pneumonitis in Patients with Non-Small Cell Lung Cancer: An Observational Analysis of Real-World Data. Oncol Ther 2021;9:471-88. [Crossref] [PubMed]
- Fujimoto D, Kato R, Morimoto T, et al. Characteristics and Prognostic Impact of Pneumonitis during Systemic Anti-Cancer Therapy in Patients with Advanced Non-Small-Cell Lung Cancer. PLoS One 2016;11:e0168465. [Crossref] [PubMed]
- Suh CH, Park HS, Kim KW, et al. Pneumonitis in advanced non-small-cell lung cancer patients treated with EGFR tyrosine kinase inhibitor: Meta-analysis of 153 cohorts with 15,713 patients: Meta-analysis of incidence and risk factors of EGFR-TKI pneumonitis in NSCLC. Lung Cancer 2018;123:60-9. [Crossref] [PubMed]
- Brahmer J, Reckamp KL, Baas P, et al. Nivolumab versus Docetaxel in Advanced Squamous-Cell Non-Small-Cell Lung Cancer. N Engl J Med 2015;373:123-35. [Crossref] [PubMed]
- Paz-Ares L, Luft A, Vicente D, et al. Pembrolizumab plus Chemotherapy for Squamous Non-Small-Cell Lung Cancer. N Engl J Med 2018;379:2040-51. [Crossref] [PubMed]
- Hellmann MD, Ciuleanu TE, Pluzanski A, et al. Nivolumab plus Ipilimumab in Lung Cancer with a High Tumor Mutational Burden. N Engl J Med 2018;378:2093-104. [Crossref] [PubMed]
- Hellmann MD, Rizvi NA, Goldman JW, et al. Nivolumab plus ipilimumab as first-line treatment for advanced non-small-cell lung cancer (CheckMate 012): results of an open-label, phase 1, multicohort study. Lancet Oncol 2017;18:31-41. [Crossref] [PubMed]
- Ready N, Hellmann MD, Awad MM, et al. First-Line Nivolumab Plus Ipilimumab in Advanced Non-Small-Cell Lung Cancer (CheckMate 568): Outcomes by Programmed Death Ligand 1 and Tumor Mutational Burden as Biomarkers. J Clin Oncol 2019;37:992-1000. [Crossref] [PubMed]
- Jabbour SK, Lee KH, Frost N, et al. Pembrolizumab Plus Concurrent Chemoradiation Therapy in Patients With Unresectable, Locally Advanced, Stage III Non-Small Cell Lung Cancer: The Phase 2 KEYNOTE-799 Nonrandomized Trial. JAMA Oncol. 2021;7:1-9. Epub ahead of print. [Crossref] [PubMed]
- Jabbour SK, Berman AT, Decker RH, et al. Phase 1 Trial of Pembrolizumab Administered Concurrently With Chemoradiotherapy for Locally Advanced Non-Small Cell Lung Cancer: A Nonrandomized Controlled Trial. JAMA Oncol 2020;6:848-55. [Crossref] [PubMed]
- Durm GA, Jabbour SK, Althouse SK, et al. A phase 2 trial of consolidation pembrolizumab following concurrent chemoradiation for patients with unresectable stage III non-small cell lung cancer: Hoosier Cancer Research Network LUN 14-179. Cancer 2020;126:4353-61. [Crossref] [PubMed]
- Antonia SJ, Villegas A, Daniel D, et al. Durvalumab after Chemoradiotherapy in Stage III Non-Small-Cell Lung Cancer. N Engl J Med 2017;377:1919-29. [Crossref] [PubMed]
- Hwang W, Niemierko A, Willers H, et al. Incidence of Pneumonitis in Metastatic Lung Cancer Patients Receiving Immune Checkpoint Inhibitors With or Without Thoracic Radiation Therapy. International Journal of Radiation Oncology, Biology, Physics 2017;99:S150. [Crossref]
- Ettinger DS, Wood DE, Aisner DL, et al. NCCN Guidelines Insights: Non-Small Cell Lung Cancer, Version 2.2021. J Natl Compr Canc Netw 2021;19:254-66. [Crossref] [PubMed]
- Kehl KL, Hassett MJ, Schrag D. Patterns of care for older patients with stage IV non-small cell lung cancer in the immunotherapy era. Cancer Med 2020;9:2019-29. [Crossref] [PubMed]
- Lu T, Yang X, Huang Y, et al. Trends in the incidence, treatment, and survival of patients with lung cancer in the last four decades. Cancer Manag Res 2019;11:943-53. [Crossref] [PubMed]
- Bauml JM, Mick R, Ciunci C, et al. Pembrolizumab After Completion of Locally Ablative Therapy for Oligometastatic Non-Small Cell Lung Cancer: A Phase 2 Trial. JAMA Oncol 2019;5:1283-90. [Crossref] [PubMed]
- Bestvina CM, Pointer KB, Karrison T, et al. A Phase 1 Trial of Concurrent or Sequential Ipilimumab, Nivolumab, and Stereotactic Body Radiotherapy in Patients With Stage IV NSCLC Study. J Thorac Oncol 2022;17:130-40. [Crossref] [PubMed]
- Theelen WSME, Peulen HMU, Lalezari F, et al. Effect of Pembrolizumab After Stereotactic Body Radiotherapy vs Pembrolizumab Alone on Tumor Response in Patients With Advanced Non-Small Cell Lung Cancer: Results of the PEMBRO-RT Phase 2 Randomized Clinical Trial. JAMA Oncol 2019;5:1276-82. [Crossref] [PubMed]
- Pitroda SP, Chmura SJ, Weichselbaum RR. Integration of radiotherapy and immunotherapy for treatment of oligometastases. Lancet Oncol 2019;20:e434-42. [Crossref] [PubMed]
- Palma D, Tree A, Guckenberger M. Should stereotactic radiotherapy be the preferred treatment for oligometastatic disease? Lancet Oncol 2021;22:1067-8. [Crossref] [PubMed]
- Palma DA, Olson R, Harrow S, et al. Stereotactic ablative radiotherapy versus standard of care palliative treatment in patients with oligometastatic cancers (SABR-COMET): a randomised, phase 2, open-label trial. Lancet 2019;393:2051-8. [Crossref] [PubMed]
- Demaria S, Ng B, Devitt ML, et al. Ionizing radiation inhibition of distant untreated tumors (abscopal effect) is immune mediated. Int J Radiat Oncol Biol Phys 2004;58:862-70. [Crossref] [PubMed]
- Reits EA, Hodge JW, Herberts CA, et al. Radiation modulates the peptide repertoire, enhances MHC class I expression, and induces successful antitumor immunotherapy. J Exp Med 2006;203:1259-71. [Crossref] [PubMed]
- Shaverdian N, Lisberg AE, Bornazyan K, et al. Previous radiotherapy and the clinical activity and toxicity of pembrolizumab in the treatment of non-small-cell lung cancer: a secondary analysis of the KEYNOTE-001 phase 1 trial. Lancet Oncol 2017;18:895-903. [Crossref] [PubMed]
- Anscher MS, Arora S, Weinstock C, et al. Association of Radiation Therapy With Risk of Adverse Events in Patients Receiving Immunotherapy: A Pooled Analysis of Trials in the US Food and Drug Administration Database. JAMA Oncol. 2022;8:232-40. [Crossref] [PubMed]
- Roell KR, Reif DM, Motsinger-Reif AA. An Introduction to Terminology and Methodology of Chemical Synergy-Perspectives from Across Disciplines. Front Pharmacol 2017;8:158. [Crossref] [PubMed]
- Enewold L, Parsons H, Zhao L, et al. Updated Overview of the SEER-Medicare Data: Enhanced Content and Applications. J Natl Cancer Inst Monogr 2020;2020:3-13. [PubMed]
- Neibart SS, Portal DE, Malhotra J, et al. Validation of a claims-based algorithm for identifying non-infectious pneumonitis in patients diagnosed with lung cancer. Pharmacoepidemiol Drug Saf 2021;30:1624-9. [Crossref] [PubMed]
- Therneau TM, Grambsch PM. The cox model. Modeling survival data: extending the Cox model: Springer; 2000. p. 39-77.
- VanderWeele TJ, Knol MJ. A tutorial on interaction. Epidemiologic Methods 2014;3:33-72. [Crossref]
- Rothman KJ. Epidemiology: an introduction: Oxford university press; 2012.
- VanderWeele TJ. Causal interactions in the proportional hazards model. Epidemiology 2011;22:713-7. [Crossref] [PubMed]
- Richardson DB, Kaufman JS. Estimation of the relative excess risk due to interaction and associated confidence bounds. Am J Epidemiol 2009;169:756-60. [Crossref] [PubMed]
- Jang JY, Kim SS, Song SY, et al. Radiation pneumonitis in patients with non-small-cell lung cancer receiving chemoradiotherapy and an immune checkpoint inhibitor: a retrospective study. Radiat Oncol 2021;16:231. [Crossref] [PubMed]
- Stoecklein VM, Osuka A, Ishikawa S, et al. Radiation exposure induces inflammasome pathway activation in immune cells. J Immunol 2015;194:1178-89. [Crossref] [PubMed]
- Zeng J, See AP, Phallen J, et al. Anti-PD-1 blockade and stereotactic radiation produce long-term survival in mice with intracranial gliomas. Int J Radiat Oncol Biol Phys 2013;86:343-9. [Crossref] [PubMed]
- Deng L, Liang H, Burnette B, et al. Irradiation and anti-PD-L1 treatment synergistically promote antitumor immunity in mice. J Clin Invest 2014;124:687-95. [Crossref] [PubMed]
- Demaria S, Kawashima N, Yang AM, et al. Immune-mediated inhibition of metastases after treatment with local radiation and CTLA-4 blockade in a mouse model of breast cancer. Clin Cancer Res 2005;11:728-34. [Crossref] [PubMed]
- Hill RP, Zaidi A, Mahmood J, et al. Investigations into the role of inflammation in normal tissue response to irradiation. Radiother Oncol 2011;101:73-9. [Crossref] [PubMed]
- Xue J, Du S, Lu Y, et al. Abstract 3671: Anti-PD-1 treatment may potentiate the radiation-induced lung injury. Cancer Res 2017;77:3671. [Crossref]
- Myers CJ, Lu B. Decreased Survival After Combining Thoracic Irradiation and an Anti-PD-1 Antibody Correlated With Increased T-cell Infiltration Into Cardiac and Lung Tissues. Int J Radiat Oncol Biol Phys 2017;99:1129-36. [Crossref] [PubMed]