Relationship between PD-L1 expression and molecular aberrances in lung adenocarcinoma with solid components
Highlight box
Key findings
• Our study revealed a clear correlation between the expression level of PD-L1 and driver mutations as well as clinicopathological characteristics in lung adenocarcinoma with solid components (LUAD-SC).
What is known and what is new?
• LUAD-SC has reportedly undermined the therapeutic response to postoperative adjuvant chemotherapy. The therapeutic management of LUAD-SC patients has been improved with molecularly targeted and immune checkpoint inhibitor (ICI) therapies.
• This work is the first large sample study to explore the operational features and molecular aberrations of the expression level of PD-L1 in LUAD-SC.
What is the implication, and what should change now?
• It is important to evaluate the percentage of solid components in both punctured and excised specimens, which may help identify cases with high PD-L1 expression.
Introduction
Lung cancer has the highest mortality rate among patients with malignancies worldwide (1). In 2011, the International Association for the Study of Lung Cancer proposed a novel histological classification, subdividing lung adenocarcinoma (LUAD) into five types (2): solid, micropapillary, acinar, papillary, and lepidic predominant types for non-mucinous adenocarcinoma. Numerous investigations have explored the link between histological type and prognosis in LUAD, revealing that the solid pattern is a poor prognostic factor in LUAD (3,4). Therefore, studying LUAD with solid components (LUAD-SC) in depth is of great clinical value.
In immunotherapies, immune checkpoint inhibitors (ICIs) are particularly interesting. Recent studies have shown promising results for programmed death ligand 1 (PD-L1) inhibitors for the treatment of advanced non-small cell lung cancer (NSCLC), particularly for patients who did not receive targeted therapy. Currently, our understanding of the distribution of PD-L1 in lung carcinoma comes mainly from randomized controlled clinical trials on NSCLC (5,6), and the reported percentage of high PD-L1 expression in cancer cells ranges from 7.5% to 16.3% in previous studies (7-9). Further, when considering the relationship between driver gene variants and PD-L1 expression, many researchers have found that certain molecular variants can affect PD-L1 expression and may influence its predictive power (10-12). However, most previous studies have been limited to single gene alterations, with occasionally conflicting results, and opinions vary. In addition, Ujiie et al. (13) identified an association between high PD-L1 expression and LUAD-SC, suggesting that this subtype may have a superior benefit in immunotherapy and greater opportunities for ICI treatment. The importance of immunotherapy is further highlighted by the fact that solid LUAD responds more poorly to EGFR-targeted agents than other types of drugs (14). However, most studies have focused on exploring NSCLC’s PD-L1 expression, and there are no corresponding large-sample studies in Chinese patients with LUAD-SC (12,15). Furthermore, it remains unknown whether the relationship that exists between PD-L1 expression levels and clinicopathological and molecular profiles in small biopsy specimens is consistent with surgically-resected specimens.
Herein, we explored the influence of PD-L1 expression on LUAD-SC, studying its correlations with clinicopathologic characteristics and driver genes in resected and biopsy specimens, respectively. We present this article in accordance with the REMARK reporting checklist (available at https://jtd.amegroups.com/article/view/10.21037/jtd-22-1095/rc).
Methods
The study was conducted in accordance with the Declaration of Helsinki (as revised in 2013). This study was approved by the Ethics Committee of Fudan University Zhongshan Hospital (approval No. B2021-141) and was performed in accordance with the approved guidelines. The requirement for written informed consent was waived due to the retrospective nature of the study. Using a pathology database, we identified all lung cancer patients at Zhongshan Hospital for whom PD-L1 immunohistochemistry (IHC) testing and gene mutation detection were conducted on the same tissue sample. The data of LUAD-SC patients who were first diagnosed and pathologically confirmed at Zhongshan Hospital, Fudan University from January 2016 to December 2019 were obtained. The medical and pathological records of patients were examined to extract the demographical and pathological data.
This study included 1,186 consecutive patient samples. The clinicopathological characteristics included sex, age, histological subtype, pathological stage, smoking history, visceral pleural invasion, disease-free survival (DFS), lymphovascular invasion, and overall survival (OS). Pathological stage was defined according to the definition of the 8th American Joint Committee on Cancer (AJCC) Staging Manual. The histological subtypes of lung cancer were divided based on the 5th World Health Organization on Lung Cancer.
The solid predominant group was defined as those with a tumor with a solid component of at least 50%, whereas adenocarcinomas with solid components of 5–45% were classified as the solid minor group. Cases with non-adenocarcinoma histology, mucinous adenocarcinomas, targeted therapy or radiotherapy, previous chemotherapy, or inadequate tumor material were excluded. The last follow-up date was January 31, 2021.
PD-L1 expression
An IHC analysis of PD-L1 expression was conducted using PD-L1 monoclonal 28-8 (Abcam, Cambridge, UK) or E1L3N (Cell Signaling Technology, Danvers, USA) antibodies on a typical tumor slide. A section containing adequate tumor tissue was called a representative slide. The assay was carried out using the Dako Automated Link 48 platform. We determined the expression of PD-L1 using the tumor proportion score (TPS), which simply refers to the percentage of viable tumor cells with at least partial membranous staining in the entire viable tumor cell population on the whole slide. Two trained pathologists performed this procedure using light microscopes (Olympus BX43, Japan). The PD-L1 subgroups were as follows: negative (<1%), low (1–49%), and high (≥50%).
Molecular analysis
Examinations for KRAS (exon 2), BRAF (exon 15), EGFR (exons 18, 19, 20, and 21), Her2 (exon 20), and PIK3CA (exons 9, 20) mutation hot-spot regions were performed for 1,186 cases using the ADx-ARMS (Amplification Refractory Mutation System) kit (Amoy Diagnostics, Xiamen, China). ALK, ROS1, and RET translocations were detected in 1,186 cases using a reverse transcription-polymerase chain reaction (RT-PCR) kit (Amoy Diagnostics).
Statistical analysis
Statistical analysis was performed using IBM SPSS Statistics version 25.0 (SPSS, Chicago, IL, USA) and R version 3.6.0 software (R Foundation for Statistical Computing, Vienna, Austria). We adopted the chi-square test or Fisher’s exact test to investigate the relationship between PD-L1 expression and clinical characteristics as well as gene mutations. The differences between groups were tested using the t-test (two groups). Variables with P<0.1 in the univariate analysis were included in the multivariate ordinal logistic regression analyses. Survival analysis was carried out using log-rank tests and presented as Kaplan-Meier curves. P<0.05 was considered statistically significant. All P values were two-sided.
Results
Clinical and molecular features of patients with LUAD-SC
A total of 1,186 patients with LUAD-SC were enrolled in this study, including 1,090 (91.91%) surgical specimens and 96 (8.09%) biopsy specimens. All cases were immunohistochemically detected for the PD-L1 protein. There were 215, 374, and 597 patients in the high, low, and negative PD-L1 subgroups, respectively (Figure 1).
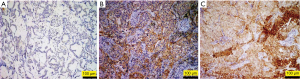
In the excised surgical specimens, there were 612 (56.15%) male patients and 478 (43.85%) female patients; also, 468 (42.94%) patients were aged ≤60 years and 622 (57.06%) patients aged >60 years. Among them, 772 (70.83%) patients had never smoked, and 352 (32.29%) patients were solid predominant. In terms of the AJCC staging, there were 425 (38.99%), 377 (34.59%), 234 (21.47%), and 54 (4.95%) patients in stages I to IV, respectively. Moreover, there were 203 (18.62%) patients with positive vascular invasion and 481 (44.13%) patients had positive pleural invasion.
As for the biopsy specimens, 54 (56.25%) patients were male and 42 (43.75%) were female. There were 38 (39.58%) patients aged ≤60 years and 58 (60.42%) patients aged >60 years. Sixty-nine patients (71.88%) were never smokers. There were 3 (3.13%) patients in stage I, 9 (9.38%) patients in stage II, 8 (8.33%) patients in stage III, and 76 (79.17%) patients in stage IV. Also, 73 (76.04%) patients were solid predominant. The detailed data are presented in Table 1. Compared with their resected counterparts, the biopsy specimens were significantly associated with solid predominant, advanced TNM stage, and high PD-L1 expression. However, there were no significant differences in the demographic variables and smoking history between these two groups.
Table 1
Variables | Resected | Percentage | Biopsy | Percentage | P |
---|---|---|---|---|---|
Gender | |||||
Male | 612 | 56.15% | 54 | 56.25% | 0.984 |
Female | 478 | 43.85% | 42 | 43.75% | |
Age | |||||
≤60 years | 468 | 42.94% | 38 | 39.58% | 0.524 |
>60 years | 622 | 57.06% | 58 | 60.42% | |
Smoking | |||||
Smokers | 318 | 29.17% | 27 | 28.13% | 0.828 |
Non-smokers | 772 | 70.83% | 69 | 71.88% | |
Lymphovascular invasion | |||||
Present | 203 | 18.62% | * | * | |
Absent | 887 | 81.38% | * | * | |
Pleural invasion | |||||
Present | 481 | 44.13% | * | * | |
Absent | 609 | 55.87% | * | * | |
Solid component | |||||
Solid predominant | 352 | 32.29% | 73 | 76.04% | <0.001 |
Solid minor | 738 | 67.71% | 23 | 23.96% | |
TNM stage | |||||
I | 425 | 38.99% | 3 | 3.13% | <0.001 |
II | 377 | 34.59% | 9 | 9.38% | |
III | 234 | 21.47% | 8 | 8.33% | |
IV | 54 | 4.95% | 76 | 79.17% | |
PD-L1 | <0.001 | ||||
High | 184 | 16.88% | 31 | 32.29% | |
Low | 340 | 31.19% | 34 | 35.42% | |
Negative | 566 | 51.93% | 31 | 32.29% | |
Genetic alternation | |||||
EGFR | 547 | 50.18% | 37 | 38.54% | 0.029 |
KRAS | 108 | 9.91% | 10 | 10.42% | 0.873 |
BRAF | 8 | 0.73% | 3 | 3.13% | 0.053 |
Her2 | 27 | 2.48% | 4 | 4.17% | 0.309 |
PIK3CA | 7 | 0.64% | 1 | 1.04% | 0.492 |
ALK | 87 | 7.98% | 11 | 11.46% | 0.236 |
RET | 16 | 1.47% | 2 | 2.08% | 0.652 |
ROS1 | 23 | 2.11% | 2 | 2.08% | 0.986 |
*, not evaluated in the biopsy specimens. LUAD-SC, lung adenocarcinoma with solid component; TNM, tumor-node-metastasis; PD-L1, programmed cell death ligand 1.
All patients were tested for eight driver mutations using the ADx-ARMS kit. As depicted in Table 1, the EGFR mutation rates in the resected versus biopsy specimens were statistically different (P=0.029, 50.18% vs. 38.54%). However, there were no differences in mutation rates for the seven remaining genes between the resected and biopsy specimens.
PD-L1 expression in terms of the clinicopathologic characteristics in LUAD-SC
Among the 1,090 excised surgical specimens, 184 patients (16.88%) had high PD-L1 expression levels (TPS ≥50 %) and 340 patients (31.19%) had low PD-L1 expression (1% ≤ TPS < 50%), leaving 566 patients (51.93%) with negative PD-L1 expression. Univariate analysis showed that high PD-L1 expression was more prevalent in male patients (P=0.011), smokers (P=0.025), patients with blood vessel invasion (P<0.001), the solid-dominant type (P<0.001), and in more serious clinical stages (P<0.001). Elevated PD-L1 expression was more observed in wild-type-gene patients (P<0.001). However, there was no significant relationship with age and pleural invasion. The specific data are presented in Table 2.
Table 2
Variables | TPS ≥50% | 1% ≤ TPS < 50% | TPS <1% | P | Adjusted P |
---|---|---|---|---|---|
Gender, n (%) | 0.011 | 0.451 | |||
Male | 120 (65.22) | 194 (57.06) | 298 (52.65) | ||
Female | 64 (34.78) | 146 (42.94) | 268 (47.35) | ||
Age, n (%) | 0.402 | * | |||
≤60 years | 75 (40.76) | 156 (45.88) | 237 (41.87) | ||
>60 years | 109 (59.24) | 184 (54.12) | 329 (58.13) | ||
Smoking, n (%) | 0.025 | 0.488 | |||
Smokers | 67 (36.41) | 103 (30.29) | 148 (25.15) | ||
Non-smokers | 117 (63.59) | 237 (69.71) | 418 (73.85) | ||
Lymphovascular invasion, n (%) | 0.000 | 0.006 | |||
Present | 50 (27.17) | 72 (21.18) | 81 (14.31) | ||
Absent | 134 (72.83) | 268 (78.82) | 485 (85.69) | ||
Pleural invasion, n (%) | 0.068 | 0.322 | |||
Present | 83 (45.11) | 166 (48.82) | 232 (40.99) | ||
Absent | 101 (54.89) | 174 (51.18) | 334 (59.01) | ||
Solid component, n (%) | <0.001 | <0.001 | |||
Solid predominant | 125 (67.93) | 110 (32.35) | 117 (20.67) | ||
Solid minor | 59 (32.07) | 230 (67.65) | 449 (79.33) | ||
Genetic alternation, n (%) | <0.001 | 0.403 | |||
Mutation | 114 (61.96) | 263 (77.35) | 438 (77.39) | ||
Wild | 70 (38.04) | 77 (22.65) | 128 (22.61) | ||
TNM stage, n (%) | <0.001 | ||||
I | 47 (25.54) | 128 (37.65) | 250 (44.17) | Reference | |
II | 60 (32.61) | 126 (37.06) | 191 (33.75) | 0.058 | |
III | 67 (36.41) | 70 (20.59) | 97 (17.14) | <0.001 | |
IV | 10 (5.43) | 16 (4.71) | 28 (4.95) | 0.085 | |
EGFR, n (%) | <0.001 | * | |||
Mutation | 54 (29.35) | 161 (47.35) | 332 (58.66) | ||
Wild | 130 (70.65) | 179 (52.65) | 234 (41.34) | ||
KRAS, n (%) | 0.005 | * | |||
Mutation | 27 (14.67) | 40 (11.76) | 41 (7.24) | ||
Wild | 157 (85.33) | 300 (88.24) | 525 (92.76) | ||
BRAF, n (%) | 0.032 | * | |||
Mutation | 3 (1.63) | 4 (1.18) | 1 (0.18) | ||
Wild | 181 (98.37) | 336 (98.82) | 565 (99.82) | ||
Her2, n (%) | 0.096 | * | |||
Mutation | 1 (0.54) | 12 (3.53) | 14 (2.47) | ||
Wild | 183 (99.46) | 328 (96.47) | 552 (97.53) | ||
PIK3CA, n (%) | 0.101 | * | |||
Mutation | 0 (0.00) | 5 (1.47) | 2 (0.35) | ||
Wild | 184 (100.00) | 335 (98.53) | 564 (99.65) | ||
ALK, n (%) | 0.185 | * | |||
Rearrangement | 17 (9.24) | 33 (9.71) | 37 (6.54) | ||
Negative | 167 (90.76) | 307 (90.29) | 529 (93.46) | ||
RET, n (%) | 0.355 | * | |||
Rearrangement | 4 (2.17) | 6 (1.76) | 6 (1.06) | ||
Negative | 180 (97.83) | 334 (98.24) | 560 (98.94) | ||
ROS1, n (%) | 0.040 | * | |||
Rearrangement | 8 (4.35) | 8 (2.35) | 7 (1.24) | ||
Negative | 176 (95.65) | 332 (97.65) | 559 (98.76) |
*, not included in the multivariate model. Adjusted P, multivariate ordinal logistic regression analysis. LUAD-SC, lung adenocarcinoma with solid component; PD-L1, programmed cell death ligand 1; TPS, tumor proportion score; TNM, tumor-node-metastasis.
Univariate analysis was also performed for 96 biopsy specimens. The results showed that only the solid-dominant type was associated with high PD-L1 expression (P<0.001), while no other clinicopathological characteristics were found to be correlated with PD-L1 expression (Table 3). Multivariate logistic regression analysis was respectively performed on resected and biopsy specimens with complete clinicopathological information. For excised surgical specimens, the results showed that lymphovascular invasion, solid-dominant type, and TNM stage were independent factors associated with high PD-L1 expression in the analyses taking PD-L1 as the ordinal outcome variable (Table 2). Meanwhile, in the biopsy specimens, the results showed that the solid dominant type was an independent factor associated with high PD-L1 expression (Table 3).
Table 3
Variables | TPS ≥50% | 1% ≤ TPS < 50% | TPS <1% | P | Adjust P |
---|---|---|---|---|---|
Gender, n (%) | 0.186 | * | |||
Male | 17 (54.84) | 23 (67.65) | 14 (45.16) | ||
Female | 14 (45.16) | 11 (32.35) | 17 (54.84) | ||
Age, n (%) | 0.973 | * | |||
≤60 years | 12 (38.71) | 14 (41.18) | 12 (38.71) | ||
>60 years | 19 (61.29) | 20 (58.82) | 19 (61.29) | ||
Smoking, n (%) | 0.225 | * | |||
Smokers | 8 (25.81) | 13 (38.24) | 6 (19.35) | ||
Non-smokers | 23 (74.19) | 21 (61.76) | 25 (80.65) | ||
Solid component, n (%) | <0.001 | <0.001 | |||
Solid predominant | 28 (90.32) | 29 (85.29) | 16 (51.61) | ||
Solid minor | 3 (9.68) | 5 (14.71) | 15 (48.39) | ||
Genetic alternation, n (%) | 0.512 | * | |||
Mutation | 20 (64.52) | 23 (67.65) | 24 (77.42) | ||
Wild | 11 (35.48) | 11 (32.35) | 7 (22.58) | ||
TNM stage, n (%) | 0.928 | * | |||
I | 1 (3.23) | 1 (2.94) | 1 (3.23) | ||
II | 4 (12.90) | 3 (8.82) | 2 (6.45) | ||
III | 4 (12.90) | 2 (5.88) | 2 (6.45) | ||
IV | 22 (70.97) | 28 (82.35) | 26 (83.87) | ||
EGFR, n (%) | 0.014 | * | |||
Mutation | 7 (22.58) | 12 (35.29) | 18 (58.06) | ||
Wild | 24 (77.42) | 22 (64.71) | 13 (41.94) | ||
KRAS, n (%) | 0.767 | * | |||
Mutation | 4 (12.90) | 4 (11.76) | 2 (6.45) | ||
Wild | 27 (87.10) | 30 (88.24) | 29 (93.55) | ||
BRAF, n (%) | 0.997 | * | |||
Mutation | 1 (3.23) | 1 (2.94) | 1 (3.23) | ||
Wild | 30 (96.77) | 33 (97.06) | 30 (96.77) | ||
Her2, n (%) | 0.117 | * | |||
Mutation | 1 (3.23) | 0 (0.00) | 3 (9.68) | ||
Wild | 30 (96.77) | 34 (100.00) | 28 (90.32) | ||
PIK3CA, n (%) | 0.646 | * | |||
Mutation | 1 (3.23) | 0 (0.00) | 0 (0.00) | ||
Wild | 30 (96.77) | 34 (100.00) | 31 (100.00) | ||
ALK, n (%) | 0.524 | * | |||
Rearrangement | 5 (16.13) | 4 (11.76) | 2 (6.45) | ||
Negative | 26 (83.87) | 30 (88.24) | 29 (93.55) | ||
RET, n (%) | 0.327 | * | |||
Rearrangement | 0 (0.00) | 2 (5.88) | 0 (0.00) | ||
Negative | 31 (100.00) | 32 (94.12) | 31 (100.00) | ||
ROS1, n (%) | 0.204 | * | |||
Rearrangement | 2 (6.45) | 0 (0.00) | 0 (0.00) | ||
Negative | 29 (93.55) | 34 (100.00) | 31 (100.00) |
*, not included in the multivariate model. Adjusted P, multivariate ordinal logistic regression analysis. LUAD-SC, lung adenocarcinoma with solid component; PD-L1, programmed cell death ligand 1; TPS, tumor proportion score; TNM, tumor-node-metastasis.
PD-L1 expression in terms of driver aberrances in LUAD-SC
In the 1,090 resected surgical specimens, we identified a relationship between PD-L1 expression and EGFR state (P<0.001), and the EGFR aberrance rate was lower in tumors in the high PD-L1 expression group (TPS ≥50%) than in those in the negative PD-L1 expression group (TPS <1%). The number of EGFR mutations in patients in the high PD-L1 expression group was 54 (29.35%, 54/184), and that in the group with negative PD-L1 was 332 (58.66%, 332/566).
Moreover, there was a link between PD-L1 expression level and KRAS state (P=0.005), and the rate of KRAS mutations was higher in tumors in the high PD-L1 expression group (TPS ≥50%). The number of KRAS mutations in the high and negative PD-L1 expression groups was 27 and 41, respectively (14.67%, 27/184; 7.24%, 41/566).
Compared with their PD-L1 negative counterparts, high PD-L1 expression harbored a significantly higher number of BRAF mutations (1.63% vs. 0.18%, P=0.032) and ROS1 rearrangement (4.35% vs. 1.24%, P=0.040). Furthermore, we found no remarkable correlation between the expression of PD-L1 and the other driver aberrances tested (Her2, PIK3CA, ALK, and RET). The specific relationship between the distribution of PD-L1 expression and driver genes is displayed in Table 2. However, in about 96 biopsy specimens, only EGFR exhibited a significant difference in terms of PD-L1 expression (P=0.014). The specific data are presented in Table 3.
Survival analysis
We did not observe a remarkable association between PD-L1 expression and DFS; however, there existed a link between PD-L1 expression and OS (P=0.037). PD-L1 was identified as a factor for poor OS in patients with LUAD-SC (Figure 2).
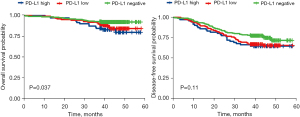
Discussion
NSCLC causes considerable deaths globally (1), in which the solid component in lung cancer is a poor prognostic factor. LUAD-SC reportedly undermines the therapeutic response to postoperative adjuvant chemotherapy (3). Therefore, in clinical practice, patients with LUAD-SC require more attention. At present, the therapeutic management of LUAD patients has been improved with molecularly targeted and ICI therapies.
To our knowledge, this is the first large sample study to explore the operational features and molecular aberrations of the PD-L1 expression level in LUAD-SC. We discovered that different clinical and molecular characteristics are linked to varied PD-L1 expression levels and that PD-L1 is a poor prognostic factor for OS in this subtype. These findings can be used to assess the expression level of PD-L1 and explain the position of PD-L1 against genomic variations.
In resected samples, high PD-L1 expression was detected in 184 of 1,090 patients (16.88%), with high levels in 59 patients (8.0%) in the solid minor group, which was greater than the high expression rate reported in previous studies (7,8). This suggests that the presence of a solid component is a favorable factor for high PD-L1 expression in lung cancer. We also found that PD-L1 expression was higher in male patients, which is consistent with previous reports (16). However, this correlation was not found in the multivariate regression analysis, which showed that vascular invasion, solid predominant subtype, and advanced disease were independent factors of high PD-L1 expression. This indicates that PD-L1 is possibly expressed often in aggressive LUADs. Most current studies have reported similar findings, with markedly elevated PD-L1 expression levels in patients with high-grade adenocarcinomas (17,18). However, in a study by Pan et al. (19) involving 1,550 patients with NSCLC, high PD-L1 expression was found to be only linked to poor tumor differentiation, while no other factors (including sex, smoking state, and histological type) were related to PD-L1 expression. Also, in biopsy specimens, PD-L1 expression was only found to be higher in solid-predominant patients. So, solid component assessment of biopsy specimens has important significance in predicting PD-L1 expression.
In this study, we identified a remarkably higher PD-L1 expression in biopsy specimens than in surgical specimens. A previous study did not report a statistically significant difference in the expression between 51 paired biopsy and resection specimens (20), which may be because the biopsy specimens were mostly from advanced patients and the heterogeneity of tumor tissues might have resulted in the observed differences. We speculate that the expression discrepancy between biopsied and resection specimens could not be caused by the tissue sampling approach.
At present, data on the prognostic effect of PD-L1 expression on NSCLC are contradictory. Numerous researchers have found that high PD-L1 expression levels are correlated with a poor prognosis in patients with lung cancer (17,21,22). Yet, there are also other reports showing that PD-LI expression is not related to prognosis (23). In the current study, OS was remarkably shorter in patients with high PD-L1 expression levels, but we did not observe a notable association between PD-L1 expression and DFS, that is, DFS was not markedly shorter in patients with high PD-L1 expression levels, suggesting the limited prognostic value of PD-L1. Nevertheless, our follow-up period was relatively short and further investigation is required to explore the prognostic role of PD-L1.
Herein, some driver mutations linked with differential expression levels of PD-L1 were identified. Mutations in KRAS and ROS1 were related to high PD-L1 expression levels. In contrast, mutations in the driver gene EGFR were found to be associated with low PD-L1 expression levels. Mutations in BRAF were correlated with high PD-L1 expression, and basic studies have reported that BRAFV600E mutations can cause upregulated PD-L1 expression (24). These results almost agree with those of recent studies, most of which reported a positive correlation between PD-L1 expression and KRAS and a negative link with EGFR, with ROS1 not showing any correlation (12,25-27). In another Korean study, it was found that ROS1 was markedly correlated with high PD-L1 expression in NSCLC (28), which is consistent with our results, and the exact mechanism of interaction between them needs to be further investigated. A Chinese study found that BRAF mutations and ROS1 were correlated with increased PD-L1 expression; however, no statistical significance was observed (15), suggesting the existence of the ethnic differences between the East and West in the relationship between PD-L1 and ROS1 driver aberrances, which has been previously reported in other similar studies (29). Meanwhile, in biopsy samples, only EGFR was found to be associated with PD-L1, which is probably attributable to a more advanced stage.
Several previous studies have shown that EGFR aberrances attenuate the prognostic effect of PD-L1 for ICIs, whereas KRAS mutations enhance the prognostic function of PD-L1 in ICI treatment (12). In addition, EGFR mutations are detrimental to ICI treatment and lead to a failure in prolonging survival (11), whereas HER2 mutations are beneficial for ICI therapy (30). High PD-L1 expression level is related to early resistance to EGFR mutation-targeted therapy and shorter OS (31). Further studies are required to investigate the efficacy of ICI therapy in patients with EGFR isogenic mutant phenotypes. Since most current targeted therapies develop resistance, it is necessary to evaluate various combinations of therapies, including immunotherapy and targeted therapy, to improve patient prognosis.
Herein, we used two kinds of PD-L1 antibodies; several studies have reported that there is a concordance between various PD-L1 antibodies, including the 28-8 and E1L3N antibodies used in this study (32,33). Despite the inconsistency in the PD-L1 antibody used, we found biological associations that were consistent with previous studies, which demonstrates the reliability of our results. However, the current study has some limitations that should be noted. First, we lacked data on the treatment of patients with mutations with ICIs or targeted drugs. Second, our follow-up time was only 3 years; further continuous follow-up is required. Third, the number of patients with ROS1 fusions in our study was small, even though we included a total of 1,186 patients. Therefore, further studies are required to validate our conclusions.
Conclusions
Our study highlights a clear correlation between the PD-L1 expression level and driver mutations as well as clinicopathological characteristics in LUAD-SC. The expression level of PD-L1 was positively related to KRAS, BRAF, and ROS1 mutations and negatively correlated with EGFR mutation. Based on the observed correlations, studies on the underlying mechanism are required to validate the causal relationship between the molecular and immunological phenotypes of cancer. Finally, it is important to evaluate the percentage of solid components in both punctured and excised specimens.
Acknowledgments
Funding: This work was supported by the Foundation for Researchers of Zhongshan Hospital (No. 17).
Footnote
Reporting Checklist: The authors have completed the REMARK reporting checklist. Available at https://jtd.amegroups.com/article/view/10.21037/jtd-22-1095/rc
Data Sharing Statement: Available at https://jtd.amegroups.com/article/view/10.21037/jtd-22-1095/dss
Peer Review File: Available at https://jtd.amegroups.com/article/view/10.21037/jtd-22-1095/prf
Conflicts of Interest: All authors have completed the ICMJE uniform disclosure form (available at https://jtd.amegroups.com/article/view/10.21037/jtd-22-1095/coif). The authors have no conflicts of interest to declare.
Ethical Statement:
Open Access Statement: This is an Open Access article distributed in accordance with the Creative Commons Attribution-NonCommercial-NoDerivs 4.0 International License (CC BY-NC-ND 4.0), which permits the non-commercial replication and distribution of the article with the strict proviso that no changes or edits are made and the original work is properly cited (including links to both the formal publication through the relevant DOI and the license). See: https://creativecommons.org/licenses/by-nc-nd/4.0/.
References
- Siegel RL, Miller KD, Jemal A. Cancer statistics, 2019. CA Cancer J Clin 2019;69:7-34. [Crossref] [PubMed]
- Travis WD, Brambilla E, Noguchi M, et al. International Association for the Study of Lung Cancer/American Thoracic Society/European Respiratory Society: international multidisciplinary classification of lung adenocarcinoma: executive summary. Proc Am Thorac Soc 2011;8:381-5. [Crossref] [PubMed]
- Zhang Y, Li J, Wang R, et al. The prognostic and predictive value of solid subtype in invasive lung adenocarcinoma. Sci Rep 2014;4:7163. [Crossref] [PubMed]
- Jeon HW, Kim YD, Sim SB, et al. Comparison of clinical results between high grade patterns in stage I lung adenocarcinoma. Thorac Cancer 2022;13:2473-9. [Crossref] [PubMed]
- Mok TSK, Wu YL, Kudaba I, et al. Pembrolizumab versus chemotherapy for previously untreated, PD-L1-expressing, locally advanced or metastatic non-small-cell lung cancer (KEYNOTE-042): a randomised, open-label, controlled, phase 3 trial. Lancet 2019;393:1819-30. [Crossref] [PubMed]
- Reck M, Rodríguez-Abreu D, Robinson AG, et al. Pembrolizumab versus Chemotherapy for PD-L1-Positive Non-Small-Cell Lung Cancer. N Engl J Med 2016;375:1823-33. [Crossref] [PubMed]
- Zheng Q, Huang Y, Zeng X, et al. Clinicopathological and molecular characteristics associated with PD-L1 expression in non-small cell lung cancer: a large-scale, multi-center, real-world study in China. J Cancer Res Clin Oncol 2021;147:1547-56. [Crossref] [PubMed]
- Wu J, Sun W, Wang H, et al. The correlation and overlaps between PD-L1 expression and classical genomic aberrations in Chinese lung adenocarcinoma patients: a single center case series. Cancer Biol Med 2019;16:811-21. [Crossref] [PubMed]
- Cruz-Rico G, Avilés-Salas A, Popa-Navarro X, et al. Association of Lung Adenocarcinoma Subtypes According to the IASLC/ATS/ERS Classification and Programmed Cell Death Ligand 1 (PD-L1) Expression in Tumor Cells. Pathol Oncol Res 2021;27:597499. [Crossref] [PubMed]
- Sabari JK, Leonardi GC, Shu CA, et al. PD-L1 expression, tumor mutational burden, and response to immunotherapy in patients with MET exon 14 altered lung cancers. Ann Oncol 2018;29:2085-91. [Crossref] [PubMed]
- Hastings K, Yu HA, Wei W, et al. EGFR mutation subtypes and response to immune checkpoint blockade treatment in non-small-cell lung cancer. Ann Oncol 2019;30:1311-20. [Crossref] [PubMed]
- Schoenfeld AJ, Rizvi H, Bandlamudi C, et al. Clinical and molecular correlates of PD-L1 expression in patients with lung adenocarcinomas. Ann Oncol 2020;31:599-608. [Crossref] [PubMed]
- Ujiie H, Kadota K, Chaft JE, et al. Solid Predominant Histologic Subtype in Resected Stage I Lung Adenocarcinoma Is an Independent Predictor of Early, Extrathoracic, Multisite Recurrence and of Poor Postrecurrence Survival. J Clin Oncol 2015;33:2877-84. [Crossref] [PubMed]
- Yoshida T, Ishii G, Goto K, et al. Solid predominant histology predicts EGFR tyrosine kinase inhibitor response in patients with EGFR mutation-positive lung adenocarcinoma. J Cancer Res Clin Oncol 2013;139:1691-700. [Crossref] [PubMed]
- Li C, Liu J, Xie Z, et al. PD-L1 expression with respect to driver mutations in non-small cell lung cancer in an Asian population: a large study of 1370 cases in China. Ther Adv Med Oncol 2020;12:1758835920965840. [Crossref] [PubMed]
- Chen Q, Fu YY, Yue QN, et al. Distribution of PD-L1 expression and its relationship with clinicopathological variables: an audit from 1071 cases of surgically resected non-small cell lung cancer. Int J Clin Exp Pathol 2019;12:774-86. [PubMed]
- Miyazawa T, Marushima H, Saji H, et al. PD-L1 Expression in Non-Small-Cell Lung Cancer Including Various Adenocarcinoma Subtypes. Ann Thorac Cardiovasc Surg 2019;25:1-9. [Crossref] [PubMed]
- Naso JR, Wang G, Pender A, et al. Intratumoral heterogeneity in programmed death-ligand 1 immunoreactivity is associated with variation in non-small cell lung carcinoma histotype. Histopathology 2020;76:394-403. [Crossref] [PubMed]
- Pan ZK, Ye F, Wu X, et al. Clinicopathological and prognostic significance of programmed cell death ligand1 (PD-L1) expression in patients with non-small cell lung cancer: a meta-analysis. J Thorac Dis 2015;7:462-70. [PubMed]
- Gradecki SE, Grange JS, Stelow EB. Concordance of PD-L1 Expression Between Core Biopsy and Resection Specimens of Non-Small Cell Lung Cancer. Am J Surg Pathol 2018;42:1090-4. [Crossref] [PubMed]
- Zhang Y, Wang L, Li Y, et al. Protein expression of programmed death 1 ligand 1 and ligand 2 independently predict poor prognosis in surgically resected lung adenocarcinoma. Onco Targets Ther 2014;7:567-73. [Crossref] [PubMed]
- Velcheti V, Schalper KA, Carvajal DE, et al. Programmed death ligand-1 expression in non-small cell lung cancer. Lab Invest 2014;94:107-16. [Crossref] [PubMed]
- Lin G, Fan X, Zhu W, et al. Prognostic significance of PD-L1 expression and tumor infiltrating lymphocyte in surgically resectable non-small cell lung cancer. Oncotarget 2017;8:83986-94. [Crossref] [PubMed]
- Siraj AK, Parvathareddy SK, Pratheeshkumar P, et al. PD-L1 Is an Independent Prognostic Marker in Middle Eastern PTC and Its Expression Is Upregulated by BRAFV600E Mutation. Cancers (Basel) 2021;13:555. [Crossref] [PubMed]
- Parra ER, Villalobos P, Zhang J, et al. Immunohistochemical and Image Analysis-Based Study Shows That Several Immune Checkpoints are Co-expressed in Non-Small Cell Lung Carcinoma Tumors. J Thorac Oncol 2018;13:779-91. [Crossref] [PubMed]
- Scheel AH, Ansén S, Schultheis AM, et al. PD-L1 expression in non-small cell lung cancer: Correlations with genetic alterations. Oncoimmunology 2016;5:e1131379. [Crossref] [PubMed]
- Lamberti G, Spurr LF, Li Y, et al. Clinicopathological and genomic correlates of programmed cell death ligand 1 (PD-L1) expression in nonsquamous non-small-cell lung cancer. Ann Oncol 2020;31:807-14. [Crossref] [PubMed]
- Lee J, Park CK, Yoon HK, et al. PD-L1 expression in ROS1-rearranged non-small cell lung cancer: A study using simultaneous genotypic screening of EGFR, ALK, and ROS1. Thorac Cancer 2019;10:103-10. [Crossref] [PubMed]
- Chan AWH, Tong JHM, Kwan JSH, et al. Assessment of programmed cell death ligand-1 expression by 4 diagnostic assays and its clinicopathological correlation in a large cohort of surgical resected non-small cell lung carcinoma. Mod Pathol 2018;31:1381-90. [Crossref] [PubMed]
- Lau SCM, Fares AF, Le LW, et al. Subtypes of EGFR- and HER2-Mutant Metastatic NSCLC Influence Response to Immune Checkpoint Inhibitors. Clin Lung Cancer 2021;22:253-9. [Crossref] [PubMed]
- Liu J, Itchins M, Nagrial A, et al. Relationship between PD-L1 expression and outcome in EGFR-mutant lung cancer patients treated with EGFR tyrosine kinase inhibitors. Lung Cancer 2021;155:28-33. [Crossref] [PubMed]
- Gaule P, Smithy JW, Toki M, et al. A Quantitative Comparison of Antibodies to Programmed Cell Death 1 Ligand 1. JAMA Oncol 2017;3:256-9. [Crossref] [PubMed]
- Tsao MS, Kerr KM, Kockx M, et al. PD-L1 Immunohistochemistry Comparability Study in Real-Life Clinical Samples: Results of Blueprint Phase 2 Project. J Thorac Oncol 2018;13:1302-11. [Crossref] [PubMed]
(English Language Editor: A. Kassem)