A bioinformatics analysis of the susceptibility genes in Stanford type A aortic dissection
Highlight box
Key findings
• Gene ontology analysis demonstrated that many genes in Stanford type A aortic dissection were involved in cell biological functions, cell components, and molecular functions through upregulating and downregulating the levels of expression.
What is known and what is new?
• Genes play an important role in Stanford type A aortic dissection.
• Many lncRNAs with different functions are involved in epigenetic modification, as well as transcription and posttranscriptional regulation of mRNAs, and play important roles in the regulation of gene expression.
What is the implication, and what should change now?
• The studies of biological indicators related to different diseases are of great significance for diagnosis and treatment. Analysis of the biological pathways revealed that the differentially expressed lncRNAs in Stanford type A aortic dissection are enriched in the cytokine receptor interaction pathway, vascular smooth muscle contraction related pathway, the actin cytoskeleton, and sticky spot signal pathway.
Introduction
Acute Stanford type A dissecting aneurysm is a life-threatening cardiovascular disease with high morbidity and mortality (1). Within the first 48 h after the occurrence of acute Stanford type A dissecting aneurysm, the mortality rate reaches 50–68%, and with the passage of time, the average hourly mortality rate increases by 1% (2-5). If treatment is not administered in a timely manner, about 90% of patients will die within 3 months. The pathogenesis of acute aortic dissection is complex, involving many factors (6), and the specific etiological mechanisms remain to be fully elucidated. The rapid development of molecular biology technology and the emergence of biochip technology has provided a powerful means to reveal the pathogenesis of diseases at the gene level, and promote the transition of medicine from the “system, blood vessel, tissue, and cell” level to the “DNA, RNA, protein, and their interaction” level (7).
Aortic dissection is the most dangerous disease in cardiovascular surgery, with a very high mortality rate (8). The disease progresses rapidly after onset, and more than 20% of patients suffer from aortic dissection rupture and died before being admitted for treatment (8). With the changes in the spectrum of cardiovascular diseases and the continuous improvements in diagnostic techniques, the detection rate and incidence of cardiovascular diseases have increased. The annual incidence of aortic dissection in the population as a whole is conservatively estimated to be approximately 5–30 per 100,000 (9). According to the International Registry of Aortic Dissection (IRAD) statistics, without treatment, patients with type A aortic dissection have a mortality rate of approximately 1–2% per hour within 48 h, 70% per week, and 80% per 2 weeks. The 3-month mortality rate is 90%, the 1-year survival rate is less than 10%, and almost all patients will die within 10 years (10). With drug treatment alone, the mortality of type A dissection is close to 20% in 24 h, 30% in 48 h, 40% in 7 days, and 50% in 1 month. With active surgical treatment, the mortality of type A dissection is 10% in 24 h, 13% in 7 days, and 20% in 1 month. Thus, the severity of aortic dissection is much higher than that of cerebral infarction, myocardial infarction, and malignant tumors, and it has become an important medical and social problem that severely affects patient well-being. Recent studies (11-13) have greatly improved the diagnosis of aortic dissection and the diversification of treatment regimens has greatly enhanced treatment efficacy. However, the specific pathogenesis of aortic dissection remains unclear. It is now thought that in addition to some specific causes as such as Marfan’s syndrome, giant cell aortic inflammation, and trauma, the occurrence of aortic dissection may involve a variety of inflammatory cells and inflammatory factors that play a role in the degradation of the extracellular matrix and vascular remodeling (14). In addition, genetic, environmental, hemodynamic, and immune factors may also play an important role (15). In recent years, with the development of molecular biology technology, researchers have begun to explore the nature of human diseases, including aortic diseases, at the gene and protein level (16-18).
Long-term hypertension may lead to vascular remodeling, intimal thickening, extracellular matrix degeneration, loss of elastic fibers, and smooth muscle cell apoptosis, which may contribute to aortic dissection. Previous studies (19-21) have confirmed that metallomatrix proteinases MMP-2 and MMP-9, as well as and its inhibitor tissue inhibitor of metalloproteinase (TIMP), are abnormally expressed in the vascular tissue of acute aortic dissection. It is believed that this is involved in the occurrence of acute aortic dissection and vascular wall tissue remodeling. A study using cDNA microarray technology have detected 3,537 known human genes in patients with acute aortic dissection (22). Of these genes, 1,250 genes were expressed in aortic tissue, and 66 genes were significantly differentially expressed between dissected aorta and normal aorta. The upregulated genes in aortic dissection included genes encoding proteins related to domain infection, extracellular matrix hydrolysis, proliferation, transcription, and translation. Downregulated genes included those encoding extracellular matrix and cytoskeleton proteins (22).
However, the relevant research is still insufficient, and the correlation between these differentially expressed genes is still unclear. This current investigation used Arraystar’s lncRNA chip technology to detect and compare the expression of lncRNAs and mRNAs in Stanford type A aortic dissection tissue and normal aortic tissue. Bioinformatic methods were used to analyze the relationship between differentially expressed lncRNAs/mRNAs, construct the gene regulatory network of aortic dissection, and explore the pathogenesis of aortic dissection. These insights will provide a theoretical basis for the diagnosis and treatment of the aortic dissection. We present the following article in accordance with the STREGA reporting checklist (available at https://jtd.amegroups.com/article/view/10.21037/jtd-23-308/rc).
Methods
Tissue collection
The tissue samples of 5 patients who presented with Stanford type A aortic dissections and the normal ascending aorta tissues from 5 donor heart transplantation patients receiving surgical treatment in Ganzhou People’s Hospital were collected, all the tissues were collected during the surgery. After obtaining the aortic wall specimen (including the sandwich flap), the blood stains were rinsed with cold 0.9% NaCl. 50 mg of aortic wall tissue was weighed and total RNA was extracted using the Trizol method. According to the instructions of the reverse transcription kit, mRNA was reverse transcribed into cDNA using a two-step method to detect gene expression. The study was conducted in accordance with the Declaration of Helsinki (as revised in 2013). The study was approved by institutional ethics board of the Ganzhou People’s Hospital (No. 202100162). Informed consent was taken from all the patients.
Normalization of the initial lncRNA data and filtering the low signal intensity values
The GeneSpring GX V12.0 software package (Agilent Technologies) was used to standardize the original data. The processing of the original signal value of the chip was standardized using the censored mean to increase the credibility of the obtained value. The censored mean is calculated by removing the maximum and minimum values, and then calculating the mean of the remaining values. The mean obtained by anti-number change is the censored mean (TM).
Preliminary analysis of mRNA data
The GeneSpring GXv12.0 software package (Agilent Technologies) was used to standardize raw mRNA data. If at least 5 of the 10 tissue samples were shown as marginal presence (MP) by standardized mRNA values, the gene would be selected for further screening of differential mRNAs in Stanford type A aortic dissection. The lncRNA/mRNA microarray was used to detect the expression of 22,959 mRNAs in more than half of the Stanford type A aortic dissection samples or normal aortic vascular tissues. The consistency and expression differences of these mRNAs in the 10 tissue specimens was further analyzed.
Target disease screening
The DisGeNET (www.disgenet.org/) and Gene Expression Onmibus (GEO; www.ncbi.nlm.nih.gov/geo/) databases were searched using the terms “vascular sandwich” and “ischemic heart disease” to identify diseases related to aortic dissection.
Differential gene screening of two categories (TwoClassDiff)
Differential gene screening of two categories (TwoClassDiff) refers to the analysis of two types of samples in gene chip experiments and real time polymerase chain reaction (RT-PCR) experiments. The first sample type is the experimental group and the other sample type is the control group. In cases where the principles of normal distribution were not satisfied, the significance level and misjudgment rate of the differences between genes were calculated using the small-sample statistical learning theory, so as to screen out the significant difference genes of two kinds of samples.
Gene Ontology (GO) analysis
The GO database is a large open biosystematics website (http://obo.sourceforge.net/) maintained by the GO Institute (http://www.geneontology.org/) and includes a number of sub-databases, such as the fly genome database (FlyBase), the yeast genome database (SGD), and the mouse genome database (MGD). The main objectives of the GO Institute are as follows: (I) to provide and maintain categories of gene ontologies; (II) to link genes with ontology, so as to carry out more effective gene function annotation and information extraction; (III) to provide tools to facilitate the use of genetic information. GO provides 3 ontological categories, namely, molecular functions (MFs), biological processes (BPs), and cell components (CCs). Within each category, a classification structure is provided that describes the relevant information. Each classified term in GO is organized into structures called directed acyclic diagrams (DAGs).
Biological pathway analysis
There are many biological interactions between genes, known as “biological pathways”. There are two main methods to analyze differentially expressed genes and their relationship to biological pathways using microarray experiments.
Statistical analysis
Based on the results of lncRNA/mRNA microarray, t-tests using the unpaired unequal variance (Welch) algorithm were performed to screen out the genes with fold change (FC) ≥2 and P value <0.05 as significantly upregulated genes. All differentially expressed genes were imported into the R package software for GO analysis and pathway analysis.
Results
Screening of lncRNAs in Stanford type A aortic dissection
Figure 1 shows the differentially expressed lncRNAs, including upregulated and downregulated lncRNAs, in Stanford type A aortic dissection.
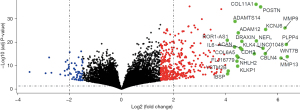
Protein-protein interaction (PPI) network analysis and module analysis
Differentially expressed genes with |log2FC| >2 were analyzed using the STRING online platform, and the Cytoscape (v 3.9.1) software was used to generate the PPI network diagram. The Cytohubba plug-in was then used to screen out the top 10 genes with the highest connectivity using the Medicines Control Council (MCC), Degree, and multinucleated cells (MNC) algorithms. The Centiscape plug-in was then combined with parameters such as Degree, Betweenness, Eigenvector, and Closeness, and so-called P and log2FC values were generated to screen for core genes (Figure 2).
Hierarchical cluster analysis of the differentially expressed lncRNAs
Hierarchical cluster analysis was performed the further study the expression of lncRNAs in Stanford type A aortic dissection and normal blood vessels (Figure 3).
GO functional analysis and Kyoto Encyclopedia of Genes and Genomes (KEGG) pathway enrichment analysis
GO analysis and KEGG pathway enrichment analysis were performed on the key targets. The differentially expressed lncRNAs were enriched in BPs including apoptosis, nitric oxide synthesis, estradiol response, angiogenesis, inflammatory response, oxidative stress, and acute response; CCs including cytoplasm, nucleus, cytoplasmic matrix, extracellular space, protein complex, and platelet α granule lumen; and MFs including protease binding, zinc ion binding, steroid compound binding, steroid hormone receptor activity, heme binding, protein kinase, cytokine, superoxide dismutase, and nitric oxide synthase activities (Figure 4).
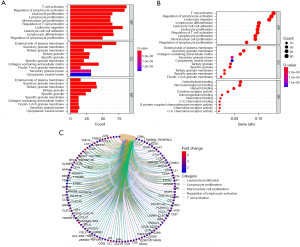
The KEGG pathway analysis demonstrated that the differentially expressed lncRNAs were enriched in the tumor necrosis factor (TNF) signaling pathway, phosphatidylinositol-3 kinase/protein kinase B (PI3K/Akt) signaling pathway, hypoxia-inducible factor-1 (HIF-1) signaling pathway, apoptosis, chemokine pathway, NOD and Toll-like receptor signaling pathway, vascular endodermal growth factor (VEGF) signaling pathway, prolactin signaling pathway, p53 signaling pathway, and adipocytokine signaling pathway (Figure 5).
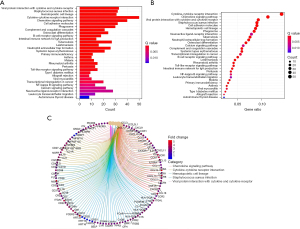
Analysis of the pathological features in the arterial wall
Figure 6A shows that the vessel wall is fully formed, consisting of the adventitia, the middle layer, and the inner layer, with the middle layer being the thickest. The normal blood vessel tissue is densely organized and the middle layer of elastic fiber is rich. The middle layer of a normal blood vessel is generally composed of 40 to 70 layers of elastic membrane, collagen fiber dimension, and smooth muscle fine cells. At the same time, the fine cell ratio is abundant. The blue stain represents the fine nuclei, and the wavy structures are elastic fibers. Figure 6B shows that the elastic fiber dimension of normal blood duct tissue is thick and wavy, and the gap between elastic forces is small (Figure 6).
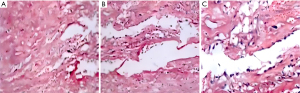
Discussion
Analysis of the lncRNA/mRNA microarray identified genes that were significantly upregulated or downregulated between acute Stanford type A aortic dissection and normal aortic tissue. Gene ontology analysis revealed the associated enriched BPs, CCs, and MFs, while KEGG analysis identified the enriched biological pathways of the differentially expressed genes.
The differential expression of genes may result in significant changes in aortic vessel wall structure, which may subsequently lead to the formation of aortic dissection (23-26). KEGG pathway analysis revealed that the differentially expressed genes of Stanford type A type aortic dissection were involved in 84 signaling pathways. The upregulated mRNAs were involved in 52 signaling pathways, with the top 3 most enriched pathways being the cytokine and cell interaction factor receptor pathway, rheumatoid arthritis signaling pathway, and Staphylococcus aureus infection signaling pathway (27). Differentially downregulated mRNAs genes in Stanford type A aortic dissection were involved in 32 signaling pathways, with the top 3 pathways being the vascular smooth muscle contraction-related pathway, actin cytoskeletal pathway, and adhesion spot signaling pathway. According to the structural characteristics and components of vascular tissue, we believe that one upregulated pathway, being the cytokine and cytokine receptor pathway, and the 3 previously mentioned downregulated pathways may be the most closely related to the pathogenesis of aortic dissection (28). Gene ontology analysis revealed that the differentially upregulated mRNAs in Stanford type A aortic dissection are involved in a variety of biological processes, especially in inflammatory stimulation and immune response (29), suggesting that inflammation is generally involved in the development of aortic dissection. Furthermore, the enriched cell components included the cytoplasm, cell membrane and components of cell membrane, extracellular space, extracellular region, and the junction part of the extracellular region. Finally, the enriched molecular functions included protein linking, signaling molecule sensor activity, chemokine activity, and cytokine receptor binding (30-32). These data indicated that the formation of aortic dissection involves a series of changes in a variety of cytokines, chemokines, and their receptors, leading to gradual changes in cellular ultrastructure that affects the tension resistance of the aortic wall.
The ontology analysis of the differentially downregulated mRNAs in Stanford type A aortic dissection revealed enrichment in a variety of biological processes, especially in cytoskeletal proteins and muscle fiber and myofibrillar contraction. This reflects the changes in the process of muscle contraction, the muscle system and systems in the process of growth and development. Genetic alterations may also lead to muscle system changes which may play a role in the development of aortic dissection. The enriched cell components were mainly the cytoplasm, cell membrane, and peripheral components, and the enrichment score showed that the changes were most significant in the contractile fiber, contractile fiber, and myofibrils. Finally, the molecular connectivity function changes were enriched in protein and cytoskeletal protein link function, and cellular receptor function. The enrichment value score suggested that actin cytoskeleton protein connections and changes in the structure of the muscular system composition may play a significant role in aortic dissection (33). The changes in cell junction components, especially cytoskeletal protein junctions, myofibrils, and actin, lead to gradual changes in cell junction contraction and cytoskeletal components, which may affect the composition structure of the aortic wall, and the tension resistance of the aortic wall.
LncRNAs are non-coding transcripts of about 200 nucleotides in length. They are located in the nucleus or cytoplasm, and rarely participate in the function of protein coding. Indeed, lncRNAs were once considered by-products in the process of transcription with no biological function. However, increasingly, a study has shown that lncRNAs may have complex biological roles, including regulation of gene expression at the epigenetic level, transcriptional level, and post-transcriptional level (34). It is now believed that lncRNAs are widely involved in almost all pathophysiological processes of the body, and is closely related to many clinical diseases (34). In recent years, the role of lncRNAs in circulatory diseases has attracted increasing attention. Some reports have confirmed that lncRNAs are important regulatory molecules involved in the development of cardiovascular diseases. A study has shown that lncRNAs can regulate transcription and translation through transcriptional interference, gene post-transcriptional regulation, translation inhibition, mRNA denaturation, production of endogenous small interfering (si)RNA, interaction with other biomolecules, activation of auxiliary proteins to inactivate target genes, change the protein position of target sites, and formation of large RNA-protein complexes. Enhancer RNAs (eRNAs) identified in this study are generated by bidirectional transcription of RNA polymerase II clustered near the enhancer region, suggesting that they may play an active role in the process of mRNA synthesis (35).
Limitations
This study was based on bioinformatics analysis. Further in vivo and in vitro experimental studies were needed to confirmed the conclusions. Moreover, we did not study the role of necrosis and immune infiltration in Stanford type A aortic dissection. Moreover, weighted correlation network analysis was not conducted in this study.
Conclusions
The studies of biological indicators related to different diseases are of great significance for diagnosis and treatment (36-40). Analysis of the biological pathways revealed that the differentially expressed lncRNAs in Stanford type A aortic dissection are enriched in the cytokine receptor interaction pathway, vascular smooth muscle contraction related pathway, the actin cytoskeleton, and sticky spot signal pathway.
Acknowledgments
Funding: None.
Footnote
Reporting Checklist: The authors have completed the STREGA reporting checklist. Available at https://jtd.amegroups.com/article/view/10.21037/jtd-23-308/rc
Data Sharing Statement: Available at https://jtd.amegroups.com/article/view/10.21037/jtd-23-308/dss
Peer Review File: Available at https://jtd.amegroups.com/article/view/10.21037/jtd-23-308/prf
Conflicts of Interest: All authors have completed the ICMJE uniform disclosure form (available at https://jtd.amegroups.com/article/view/10.21037/jtd-23-308/coif). The authors have no conflicts of interest to declare.
Ethical Statement: The authors are accountable for all aspects of the work in ensuring that questions related to the accuracy or integrity of any part of the work are appropriately investigated and resolved. The study was conducted in accordance with the Declaration of Helsinki (as revised in 2013). The study was approved by institutional ethics board of the Ganzhou People’s Hospital (No. 202100162). Informed consent was taken from all the patients.
Open Access Statement: This is an Open Access article distributed in accordance with the Creative Commons Attribution-NonCommercial-NoDerivs 4.0 International License (CC BY-NC-ND 4.0), which permits the non-commercial replication and distribution of the article with the strict proviso that no changes or edits are made and the original work is properly cited (including links to both the formal publication through the relevant DOI and the license). See: https://creativecommons.org/licenses/by-nc-nd/4.0/.
References
- Zhu Y, Lingala B, Baiocchi M, et al. Type A Aortic Dissection-Experience Over 5 Decades: JACC Historical Breakthroughs in Perspective. J Am Coll Cardiol 2020;76:1703-13. [Crossref] [PubMed]
- Bashir M, Harky A, Howard C, et al. Type A Aortic Dissection in the United Kingdom: The Untold Facts. Semin Thorac Cardiovasc Surg 2019;31:664-7. [Crossref] [PubMed]
- Tadros RO, Tang GHL, Barnes HJ, et al. Optimal Treatment of Uncomplicated Type B Aortic Dissection: JACC Review Topic of the Week. J Am Coll Cardiol 2019;74:1494-504. [Crossref] [PubMed]
- Elefteriades JA, Ziganshin BA. A new 'angle' towards prediction of type A aortic dissection. Eur J Cardiothorac Surg 2021;60:987-8. [Crossref] [PubMed]
- Fukuhara S, Norton EL, Chaudhary N, et al. Type A Aortic Dissection With Cerebral Malperfusion: New Insights. Ann Thorac Surg 2021;112:501-9. [Crossref] [PubMed]
- Yang B, Norton EL, Rosati CM, et al. Managing patients with acute type A aortic dissection and mesenteric malperfusion syndrome: A 20-year experience. J Thorac Cardiovasc Surg 2019;158:675-687.e4. [Crossref] [PubMed]
- Frankel WC, Green SY, Orozco-Sevilla V, et al. Contemporary Surgical Strategies for Acute Type A Aortic Dissection. Semin Thorac Cardiovasc Surg 2020;32:617-29. [Crossref] [PubMed]
- Antoniou A, Bashir M, Harky A, et al. Decoding the volume-outcome relationship in Type A aortic dissection. Gen Thorac Cardiovasc Surg 2019;67:32-6. [Crossref] [PubMed]
- Chivasso P, Mastrogiovanni G, Miele M, et al. Frozen Elephant Trunk Technique in Acute Type A Aortic Dissection: Is It for All? Medicina (Kaunas) 2021;57:894. [Crossref] [PubMed]
- Munir W, Chong JH, Harky A, et al. Type A aortic dissection: involvement of carotid artery and impact on cerebral malperfusion. Asian Cardiovasc Thorac Ann 2021;29:635-42. [Crossref] [PubMed]
- Kreibich M, Rylski B, Czerny M, et al. Type A Aortic Dissection in Patients With Bicuspid Aortic Valve Aortopathy. Ann Thorac Surg 2020;109:94-100. [Crossref] [PubMed]
- Sfeir PM, Issa K, Ayoub CM. Mesenteric Malperfusion Syndromes in Type A Aortic Dissection: Current Management Strategies. J Cardiothorac Vasc Anesth 2021;35:3711-9. [Crossref] [PubMed]
- Saw LJ, Lim-Cooke MS, Woodward B, et al. The surgical management of acute type A aortic dissection: Current options and future trends. J Card Surg 2020;35:2286-96. [Crossref] [PubMed]
- Bashir M, Harky A, Shaw M, et al. Type A aortic dissection in patients over the age of seventy in the UK. J Card Surg 2019;34:1439-44. [Crossref] [PubMed]
- Lansman SL, Goldberg JB, Kai M, et al. Extended Arch Procedures for Acute Type A Aortic Dissection: A Downstream Problem? Semin Thorac Cardiovasc Surg 2019;31:17-20. [Crossref] [PubMed]
- Yadav R, Mughal H, Rimmer L, et al. From ER to OR-Type A aortic dissection delay dilemma. J Card Surg 2021;36:1056-61. [Crossref] [PubMed]
- Runyan B, Hanak CR, Mahendiran S, et al. Type A Aortic Dissection Complicated by Pheochromocytoma. Ann Thorac Surg 2019;107:e13-4. [Crossref] [PubMed]
- Speir AM. Commentary: Regionalization of surgery for type A aortic dissection: What does this really mean? J Thorac Cardiovasc Surg 2021;161:1738-9. [Crossref] [PubMed]
- Poleri I, Dias-Neto M, Rocha-Neves J, et al. Type B Aortic Dissection - A Single Center Series. Rev Port Cir Cardiotorac Vasc 2019;26:131-7. [PubMed]
- Hattori S, Noguchi K, Gunji Y, et al. Acute type A aortic dissection in non-agenarians: to cut or not. Interact Cardiovasc Thorac Surg 2020;31:102-7. [Crossref] [PubMed]
- Fukuhara S, Tang H, Kim KM, et al. Type A Aortic Dissection During COVID-19 Pandemic: Report From Tertiary Aortic Centers in the United States and China. Semin Thorac Cardiovasc Surg 2021;33:303-12. [Crossref] [PubMed]
- Gold AK, Raiten J, Augoustides JG. Mesenteric Malperfusion in Type A Aortic Dissection-The Importance and Clinical Utility of the Penn Classification. J Cardiothorac Vasc Anesth 2021;35:3720-2. [Crossref] [PubMed]
- Di Marco L, Leone A, Murana G, et al. Acute type A aortic dissection: Rationale and outcomes of extensive repair of the arch and distal aorta. Int J Cardiol 2018;267:145-9. [Crossref] [PubMed]
- Wang L, Zhao Y, Zhang W, et al. Retrograde Type A Aortic Dissection after Thoracic Endovascular Aortic Repair: Incidence, Time Trends and Risk Factors. Semin Thorac Cardiovasc Surg 2021;33:639-53. [Crossref] [PubMed]
- Comentale G, D'Andrea C, Pilato E, et al. Type A Aortic Dissection in an 11-month-old Infant With Loeys-Dietz Syndrome. Ann Thorac Surg 2021;112:e287-9. [Crossref] [PubMed]
- Nihira T, Yamada N. Type A aortic dissection associated with tension pneumothorax. Am J Emerg Med 2019;37:1218.e1-3. [Crossref] [PubMed]
- Velayudhan BV, Idhrees AM. Alternatives to the frozen elephant trunk in acute type A aortic dissection. Asian Cardiovasc Thorac Ann 2021;29:623-6. [Crossref] [PubMed]
- Kim WK, Park SJ, Kim HJ, et al. The fate of unrepaired chronic type A aortic dissection. J Thorac Cardiovasc Surg 2019;158:996-1004.e3. [Crossref] [PubMed]
- Leone A, Di Marco L, Murana G, et al. The Decision-Making Process in Acute Type A Aortic Dissection: When to Replace the Aortic Arch. Semin Thorac Cardiovasc Surg 2019;31:691-6. [Crossref] [PubMed]
- Gomibuchi T, Seto T, Naito K, et al. Strategies to improve outcomes for acute type A aortic dissection with cerebral malperfusion. Eur J Cardiothorac Surg 2021;59:666-73. [Crossref] [PubMed]
- Inoue T, Ichihara T, Masuyama S. Stanford Type A Acute Aortic Dissection with Myasthenia Gravis:Report of a Case. Kyobu Geka 2021;74:139-41. [PubMed]
- Bezerra PCLB, Lima RC, Salerno PR, et al. Management of Acute Type A Aortic Dissection at a Public Cardiac Center in the Northeast Region of Brazil. Braz J Cardiovasc Surg 2021;36:150-7. [Crossref] [PubMed]
- Grimm JC, Sultan I. When It Comes to Type A Aortic Dissection: It's All About Malperfusion, Malperfusion, Malperfusion. Ann Thorac Surg 2021;111:60-1. [Crossref] [PubMed]
- Norton EL, Farhat L, Wu X, et al. Managing Malperfusion Syndrome in Acute Type A Aortic Dissection With Previous Cardiac Surgery. Ann Thorac Surg 2021;111:52-60. [Crossref] [PubMed]
- DeFabio DC, DeFabio CJ. Exercise parameters for the chronic type B aortic dissection patient: a literature review and case report. Postgrad Med 2021;133:217-22. [Crossref] [PubMed]
- Chen Y, Wang J, Zhang X, et al. Correlation between apparent diffusion coefficient and pathological characteristics of patients with invasive breast cancer. Ann Transl Med 2021;9:143. [Crossref] [PubMed]
- Qi A, Li Y, Yan S, et al. Effect of postoperative chemotherapy on blood glucose and lipid metabolism in patients with invasive breast cancer. Gland Surg 2021;10:1470-7. [Crossref] [PubMed]
- Qi A, Li Y, Yan S, et al. Effect of anthracycline-based postoperative chemotherapy on blood glucose and lipid profiles in patients with invasive breast cancer. Ann Palliat Med 2021;10:5502-8. [Crossref] [PubMed]
- Qi A, Li Y, Sun H, et al. Incidence and risk factors of sexual dysfunction in young breast cancer survivors. Ann Palliat Med 2021;10:4428-34. [Crossref] [PubMed]
- Teng D, Xia S, Hu S, et al. miR-887-3p Inhibits the Progression of Colorectal Cancer via Downregulating DNMT1 Expression and Regulating P53 Expression. Comput Intell Neurosci 2022;2022:7179733. [Crossref] [PubMed]
(English Language Editor: J. Teoh)