Development of prognostic models to estimate the probability of lung injury one year after COVID-19-related hospitalization—a prospective study
Introduction
The first cases of pneumonia of unknown origin were reported in Wuhan, Hubei, China, in December 2019 (1). In January 2020, a novel coronavirus [severe acute respiratory syndrome coronavirus 2 (SARS-CoV-2)] was isolated in these patients. The disease caused by this virus was named coronavirus disease 2019 (COVID-19) (2). In October 2022, the World Health Organization (WHO) had confirmed near 620 million cases and 6.5 million deaths (3). This disease, associated with high hospitalization rates (4), represents a global threat to public health even in developed countries.
COVID-19 is associated with a wide variety of symptoms, ranging from asymptomatic infection to life-threatening complications such as acute respiratory distress (ARDS), multi-organic failure, and death (1,5-7). Some therapies (8) seem to improve prognosis, and the majority of patients recover from the disease. Other patients, however, are at a higher risk of developing severe pulmonary complications with potential long-term effects (9,10). Such is the case of patients with an advanced age and comorbidities (6). Apart from long-term health complications, patients may also experience a wide variety of symptoms (physical and mental) that may persist and impair their quality of life. These persistent symptoms constitute the so-called post-acute COVID-19 syndrome (11,12). Patients with post-acute COVID-19 syndrome need long-term follow-up. Considering their acquired disability, declined quality of life, and increased use of healthcare resources use, specific health programs should be designed for these patients.
Although the prevalence of this syndrome is not high, massive SARS-COV-2 infection may pose a serious public health problem, due to the intensive use of health resources that this syndrome involves. In the light of the inconsistent evidence currently available, it is necessary to develop tools that identify patients at a higher risk of developing lung injury after SARS-CoV-2 hospitalization. The use of these tools would facilitate the development of specific integral follow-up programs from which these patients would benefit.
The objectives of this study were to identify the persistent pulmonary lesions that long-stay patients developed one year after SARS-COV-2 infection and investigate whether it is possible to estimate the probability to develop persistent respiratory symptoms. We present this article in accordance with the STROBE reporting checklist (available at https://jtd.amegroups.com/article/view/10.21037/jtd-22-1565/rc).
Methods
Study design
This prospective study was conducted in a 1,000-bed tertiary university hospital serving a population of 450,000. The study was conducted in accordance with the Declaration of Helsinki (as revised in 2013). The study was approved by the institutional ethics committee of Santiago-Lugo (Registration No. 2020/305) and informed consent was taken from all the patients.
Following American Thoracic Society and European Respiratory Society (13) recommendations, the regional health system of Galicia (Servicio Gallego de Salud) promoted the creation of multidisciplinary post-COVID-19 units for the follow-up of patients who have been hospitalized for SARS-CoV-2 infection (14). In our center, post-COVID-19 Unit was composed of specialists from Internal Medicine and Pulmonology Departments.
Procedures
Sample collection for the diagnosis of COVID-19 was performed in accordance with WHO protocol (15). Criteria for hospitalization were the presence of pulmonary opacities on thoracic radiography, having a SaO2 <95% and a respiratory rate >25/minute, and/or being a high-risk patient (subjects >60 years with comorbidities). In patients with mobility problems, diagnosis of pneumonia was established by lung ultrasound (16). Diagnosis of ARDS was established in accordance with Berlin definition (17).
All adult patients (≥18 years) hospitalized for SARS-CoV-2 infection were by Internal Medicine Unit. In the initial follow-up visit, complementary studies were performed (including, in all cases, a postero-anterior and lateral chest X-ray) to exclude the presence of subacute processes/lesions related to COVID-19. Patients were referred for pulmonology follow-up in the presence of exertional dyspnea, baseline SaO2 ≤95% (unrelated to a previous known disease), pathological findings on chest X-ray (conducted at 6–8 weeks after discharge and not present prior to COVID-19), pulmonary embolism during hospitalization and/or discharge with home oxygen therapy.
Pulmonology department prepared a medical record and conducted the following studies: detailed physical examination, chest radiograph (2 projections), blood test (including immunoglobulins and alpha 1 antitrypsin), spirometry (with bronchodilator and gas diffusion test) and baseline SaO2/arterial blood gas analysis. If all studies were unremarkable, the patient was discharged. If the patient exhibited abnormalities, depending on the type of finding, a thoracic high-resolution CT scan (HRCT) (CT angiography upon suspicion of pulmonary embolism) was performed. Six-minute walking test (6MWT) and/or a transthoracic echocardiogram were carried out to detect potential right ventricular dysfunction or pulmonary hypertension. All patients with abnormalities were follow-up. Patients younger than 18 years and those who declined to participate were excluded.
Demographic, clinical, radiological, and laboratory data, along with respiratory function test results were collected. Functional status was assessed using post-COVID-19 functional status scale (PCFS) (18). Spirometry and diffusion tests were carried out in accordance with current guidelines (19,20). Variables were expressed as percentages with respect to reference values (21,22). Reference values for 6MWT were obtained from standard guidelines (23). Findings on thoracic HRCT were classified in accordance with current recommendations (24). Diagnosis of a post-COVID-19 fibrotic pulmonary lesion was established based on the presence of a fibrotic lesions on thoracic HRCT with functional impact [forced vital capacity (FVC)% and/or diffusion capacity for carbon monoxide (DLCO)% <80% of the reference value], regardless of the presence or absence of clinical symptoms.
Statistical analysis
Continuous variables were described as median values and interquartile ranges. Categorical values were expressed as absolute and relative frequencies. Differences between baseline values and 1-year values were assessed using Wilcoxon test for continuous variables and the McNemar test for categorical values. Differences between the patients who stayed in a conventional hospitalization ward and those who were admitted to the critical care unit were assessed using the Mann-Whitney U test (continuous variables) and Chi-squared test (categorical variables). Logistic regression was used to estimate the probability of developing complications one year after discharge. Two prognostic models were built: one for persistent respiratory symptoms, and another for post-COVID-19 fibrotic pulmonary lesions. Based on a model containing all potential covariates, the variable with the least significant P value was removed and tested using the likelihood-ratio test until all variables left in the model (at alpha =0.05) contributed significantly to the model. Results are presented as odds ratio (OR) with 95% confidence intervals (CIs). Model performance was assessed in terms of calibration and discrimination. Calibration was assessed using the Brier score (25). The receiver operating characteristics (ROC) curves [and the corresponding area under the ROC curve (AUC)] were calculated to test discrimination. To correct optimism, internal validation was performed for each model using the bootstrap procedure with 1,000 bootstrapped samples (25). Analyses were carried out using pROC (26) and rms (27) software packages and ggplot plots (28), all available on the R free software (29). The analysis conforms to the reporting standards of TRIPOD (30).
Results
Between March 1, 2020, and July 31, 2021, a total of 22,749 patients received a diagnosis of COVID-19, of whom 2,053 needed hospitalization. Figure 1 shows the inclusion flowchart. Patients characteristics are shown in Table 1. Of the 275 patients included, 42 were excluded in the initial pulmonology visit as they only had mild clinical symptoms and complementary studies were unremarkable. Follow-up of the remainder 233 patients was performed. Median age was 66 years [interquartile range (IQR): 56, 74], and 138 (59.2%) were male. Statistically significant differences were observed between the patients who stayed in a conventional hospitalization ward and those who stayed in CCU. The patients who stayed in the CCU were generally male, exhibited more extensive radiological lesions, had a longer mean stay, needed more frequently high-flow nasal cannulae, non-invasive ventilation (NIV), orotracheal intubation (OTI) and/or a tracheostomy. In addition, these cases generally developed ADRS and obtained higher scores on PCFS scale. Finally, CCU patients showed higher average of peak values of inflammatory markers.
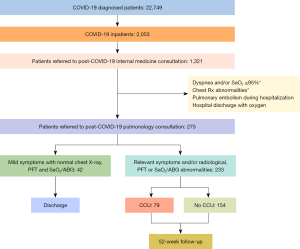
Table 1
Characteristics | Total | Hospitalization ward | CCU | P |
---|---|---|---|---|
Total, n (%) | 233 | 154 (66.1) | 79 (33.9) | |
Men, n (%) | 138 (59.2) | 83 (53.9) | 55 (69.6) | 0.029 |
Age (years), mean (IQR) | 66 (56, 74) | 64 (56, 75) | 68 (56.5, 73) | 0.073 |
Smoking (former or current), n (%) | 119 (51.1) | 77 (50.0) | 42 (53.2) | 0.749 |
Coexisting conditions, n (%) | ||||
Hypertension | 119 (51.1) | 74 (48.1) | 45 (57.0) | 0.25 |
Diabetes | 54 (23.2) | 32 (20.8) | 22 (27.9) | 0.295 |
COPD | 22 (9.4) | 16 (10.4) | 6 (7.6) | 0.649 |
Asthma | 26 (11.2) | 17 (11.0) | 9 (11.4) | 1 |
ILD | 13 (5.6) | 12 (7.8) | 1 (1.3) | 0.065 |
OSA | 53 (22.8) | 30 (19.5) | 23 (29.1) | 0.101 |
Radiological data | <0.001 | |||
Normal | 23 (9.9) | 22 (14.3) | 1 (1.3) | |
One lobe | 4 (1.7) | 4 (2.6) | 0 (0.0) | |
Two lobes | 197 (84.6) | 119 (77.3) | 78 (98.7) | |
Bilateral | 7 (3.0) | 7 (4.6) | 0 (0.0) | |
Analytical parameters, mean (IQR) | ||||
Peak CRP, mg/L‡ | 11.2 [ 5.1, 17.1] | 8.38 [3.6, 14] | 16.73 [11.2, 23.41] | <0.001 |
Peak D-dimer, ng/mL‡ | 1,364 [732.5, 4,002.5] | 981 [594.3, 1,574] | 3,918 [1,835.5, 13,266] | <0.001 |
Peak IL-6 pg/mL‡ | 40.5 [14, 197] | 24 [9, 43] | 281 [93, 1,254.5] | <0.001 |
Peak LDH, IU/L‡ | 574 [422.5, 724] | 545 [403, 668.8] | 669 [487.5, 842.5] | <0.001 |
Mean length of stay (days), mean (IQR) | 13 (7, 24) | 9 (6, 14.8) | 31 (20, 60.5) | <0.001 |
HFNC, n (%) | 56 (68.3) | 3 (1.9) | 53 (67.1) | <0.001 |
NIV, n (%) | 17 (21.5) | 0 (0.0) | 17 (21.5) | 1 |
OTI, n (%) | 54 (68.4) | 0 (0.0) | 54 (68.4) | 1 |
Tracheotomy, n (%) | 18 (22.8) | 0 (0.0) | 18 (22.8) | 1 |
ARDS, n (%) | 131 (56.2) | 53 (34.4) | 78 (98.7) | <0.001 |
Mild | 46 (35.4) | 41 (77.4) | 5 (6.5) | |
Moderate | 28 (21.5) | 9 (17.0) | 19 (24.7) | |
Severe | 56 (43.1) | 3 (5.7) | 53 (68.8) | |
Dyspnea, n (%) | 0.425 | |||
0 | 12 (5.2) | 10 (6.5) | 2 (2.5) | |
1 | 108 (46.4) | 72 (46.8) | 36 (45.6) | |
≥2 | 113 (48.5) | 72 (46.8) | 41 (51.9) | |
PCFS scale, n (%) | <0.001 | |||
0 | 57 (24.5) | 43 (27.9) | 14 (17.7) | |
1 | 65 (27.9) | 38 (24.7) | 27 (34.2) | |
2 | 84 (36.1) | 63 (40.9) | 21 (26.6) | |
≥3 | 27 (11.6) | 10 (6.5) | 17 (21.5) |
‡, maximum concentration during admission. CCU, critical care unit; IQR, interquartile range; COPD, chronic obstructive pulmonary disease; ILD, interstitial lung disease; OSA, obstructive sleep apnea; CRP, C-reactive protein; IL-6, interleukin-6; LDH, lactate dehydrogenase; HFNC, high flow nasal cannulas; NIV, noninvasive ventilation; OTI, orotracheal intubation; ARDS, acute respiratory distress syndrome; PCFS, post-COVID-19 functional status.
Table 2 shows the level of dyspnea, radiological findings, respiratory function test results, and PCFS score in initial visit and at 52 weeks by type of hospitalization unit (conventional hospitalization ward vs. CCU). At the end of follow-up, variables had improved significantly (P<0.001). Radiological findings were not compared, as chest radiography was performed in the initial visit, whereas a HRCT was performed in the final visit. Figure 2A-2C show differences between the two visits in terms of FEV1%, FVC% and DLCO% (median and IQR), and course of the patients between visits. PCFS scores at the initial visit and at week 52 are shown in Figure 2D.
Table 2
Characteristics | Initial | At 52 weeks | P | ||||||||
---|---|---|---|---|---|---|---|---|---|---|---|
Total | Ward | CCU | Total | Ward | CCU | Total | Ward | CCU | |||
Clinical | |||||||||||
Dyspnea (mMRC) , n (%) | <0.001 | <0.001 | 0.004 | ||||||||
0 | 12 (5.2) | 10 (6.5) | 2 (2.5) | 87 (37.3) | 55 (35.7) | 32 (40.5) | |||||
1 | 108 (46.4) | 72 (46.8) | 36 (45.6) | 106 (45.5) | 73 (47.4) | 33 (41.8) | |||||
≥2 | 113 (48.5) | 72 (46.8) | 41 (51.9) | 40 (17.2) | 26 (16.9) | 14 (17.7) | |||||
Radiological findings, n (%) | |||||||||||
Imaging test | X-ray | X-ray | X-ray | HRCT | HRCT | HRCT | |||||
No consolidation | 170 (73.0) | 12 (83.8) | 41 (51.9) | ||||||||
Persistence of pulmonary lesions | 63 (27.0) | 25 (16.2) | 38 (48.1) | ||||||||
Ground glass opacities | 33 (14.2) | 10 (21.4) | 23 (29.1) | ||||||||
Unspecific radiological findings | 24 (10.3) | 10 (21.4) | 14 (17.7) | ||||||||
Predominantly fibrotic | 27 (11.6) | 6 (3.9) | 21 (26.6) | ||||||||
Respiratory function test, mean (IQR) | |||||||||||
FEV1 (%) | 98.5 [80.7, 115] | 100.5 [79, 117.2] | 97 [80, 111.2] | 101 [83.5, 118] | 101.5 [82, 118] | 101 [86.2, 113.2] | <0.001 | 0.039 | 0.002 | ||
FVC (%) | 99.5 [84, 117] | 108 [91, 117] | 97 [80, 111.2] | 104 [ 88.7, 115.2] | 106 [ 89.7, 120.2] | 101 [86.2, 113.2] | <0.001 | 0.044 | 0.002 | ||
DLCO | 69 [59, 82] | 68 [54, 81] | 74 [62.5, 82] | 77 [63, 87.5] | 74 [63, 86] | 81.50 [69, 87.7] | <0.001 | <0.001 | <0.001 | ||
DLCO/AV | 93 [77.5, 103] | 86.50 [71.7, 96.5] | 96 [87.5, 105] | 99 [ 84.5, 111] | 89 [79, 109] | 102.50 [95.5, 113.7] | <0.001 | 0.001 | <0.001 | ||
Functional status | |||||||||||
PCFS scale, n (%) | <0.001 | <0.001 | <0.001 | ||||||||
0 | 57 (24.5) | 43 (27.9) | 14 (17.7) | 115 (49.4) | 73 (47.4) | 42 (53.2) | |||||
1 | 65 (27.9) | 38 (24.7) | 27 (34.2) | 72 (30.9) | 52 (33.8) | 20 (25.3) | |||||
2 | 84 (36.1) | 63 (40.9) | 21 (26.6) | 30 (12.9) | 21 (13.6) | 9 (11.4) | |||||
≥3 | 27 (11.6) | 10 (6.5) | 17 (21.5) | 16 (6.9) | 8 (5.2) | 8 (10.1) |
CCU, critical care unit; mMRC, modified Medical Research Council dyspnea scale; HRCT, high-resolution computed tomography; IQR, interquartile range; FEV1, forced expiratory volume in 1 second; FVC, forced vital capacity; DLCO, diffusion capacity for carbon monoxide; AV, alveolar volume; PCFS, Post-COVID-19 functional status; X-Ray, chest radiography.
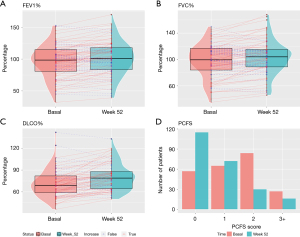
Table 3 detail the demographic and clinical characteristics of patients with respiratory symptoms (dyspnea ≥2 on the mMRC), and post-COVID-19 fibrotic pulmonary lesions (dominantly fibrotic lesions with deterioration of respiratory function) at 52 weeks, according to whether they stayed in a conventional hospitalization ward or in CCU. A total of 179 patients (76.8%) exhibited respiratory symptoms (including dyspnea ≥1 on the mMRC); 46 (25.7%) had a PCFS ≥2; 33 (14.2%) had ground-glass opacities (GGO); 24 (10.3%) had indeterminate radiological lesions; 27 (11.6%) showed fibrotic lesions on HRCT; and 57 (58.2%) had some pulmonary function parameter <80% of the predicted value. Twenty-two patients (9.4%) presented fibrotic lesions on HRCT and impairment of pulmonary function (FVC% and/or DLCO% <80% of its predicted value); therefore, they were considered to have post-COVID-19 fibrotic pulmonary lesions. CCU admission was not associated with a higher risk of developing persistent pulmonary lesions, except for fibrotic lesions on HRCT and post-COVID-19 pulmonary fibrotic lesions (P<0.001) (Table 3).
Table 3
Characteristics | Total | Hospitalization ward | CCU | P |
---|---|---|---|---|
Demographic characteristics | ||||
Total, n (%) | 179 | 120 (67.0) | 59 (33.0) | 0.623 |
Male, n (%) | 98 (54.8) | 60 (50.0) | 38 (64.4) | 0.079 |
Age (years) (mean, IQR) | 66 (55.5, 75) | 64.5 (55.8, 76) | 68 (55.5, 72.5) | 0.813 |
Clinical characteristics, n (%) | ||||
Dyspnea (mMRC ≥2) | 40 (22.3) | 26 (21.7) | 14 (23.7) | 0.815 |
Cough | 64 (35.8) | 39 (32.5) | 25 (42.4) | 0.245 |
Asthenia | 28 (15.6) | 22 (18.3) | 6 (10.2) | 0.192 |
Post-COVID-19 fibrotic pulmonary lesions, n (%) | ||||
Dominantly fibrotic lesions with deterioration of respiratory function | 22 (9.4) | 4 (2.6) | 18 (22.8) | <0.001 |
COVID-19, coronavirus disease 2019; CCU, critical care unit; IQR, interquartile range; mMRC, modified Medical Research Council dyspnea scale.
Following prognostic factors were included in Model 1: PCFS at initial visit (the higher the score, the higher the risk) and previous bronchial asthma. Model 2 for post-COVID-19 fibrotic pulmonary lesions included the following prognostic factors: being female; FVC% at the initial visit (the lower the FVC%, the higher the probability); and CCU stay (Table 4). Models 1 and 2 showed a good and excellent power of discrimination, respectively [AUC 0.857 (95% CI: 0.799, 0.915)] (Figure 3A) and [AUC 0.901 (95% CI: 0.837 0.964)] (Figure 3B), respectively. Their power of discrimination remained the same after adjustment for optimism bias using 1,000 bootstrap samples (AUC =0.844 for Model 1, and AUC =0.884 for Model 2). Calibration of the two models was excellent (Brier score of Model 1=0.118 and Model 2=0.060).
Table 4
Prognostic model | OR | 95% CI | P |
---|---|---|---|
Persistent symptoms | |||
Age | 0.988 | 0.956–1.020 | 0.451 |
Gender (male) | 0.604 | 0.267–1.369 | 0.225 |
PCFS | |||
0 (reference) | — | — | |
1 | 4.349 | 1.881–10.054 | <0.001 |
≥2 | 57.881 | 15.270–219.397 | <0.001 |
Asthma | 6.788 | 1.342–34.342 | 0.019 |
Post-COVID-19 fibrotic pulmonary lesions | |||
Age | 1.01 | 0.968–1.055 | 0.633 |
Gender (male) | 0.286 | 0.083–0.988 | 0.046 |
FVC% | 0.94 | 0.915–0.966 | <0.001 |
CCU | 14.413 | 3.810–54.525 | <0.001 |
SARS-CoV-2, severe acute respiratory syndrome coronavirus 2; OR, odds ratio; 95% CI, 95% confidence interval; PCFS, post-COVID-19 functional status; FVC, forced vital capacity; CCU, critical care unit.
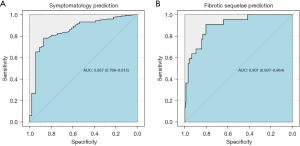
Figure 4 shows clinical scores for both models. The total score indicates the estimated individual risk of having post-COVID lung complications. For instance, a patient with a PCFS score of 1 (34 points) and asthma (49 points), obtains a total score of 83 points, which corresponds to a 95% probability of developing persistent respiratory symptoms. This model has a specificity of 0.94, with a positive predictive value of 0.97. Regarding post-COVID-19 pulmonary fibrotic lesions, being female (13 points); an initial FVC% of 80% (67 points); and no CCU admission (0 points), yields a total score of 80 points, which corresponds to a 10% probability of developing pulmonary fibrotic lesions.
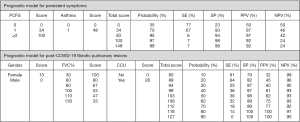
Discussion
The results of this study reveal that a high proportion of patients hospitalized for COVID-19 have a risk of experiencing persistent respiratory symptoms, impaired pulmonary function, changes in pulmonary functional status or fibrotic pulmonary lesions on radiological studies a year after infection. Prognostic factors of persistent respiratory symptoms after COVID-19 include PCFS at the initial visit and history of bronchial asthma. Sex, percentage of FVC with respect to initial visit and CCU stay are prognostic factors of post-COVID-19 fibrotic pulmonary lesions.
As many as 76.8% of patients [179] had dyspnea (≥1 of mMRC), cough or asthenia a year after SARS-CoV-2 infection, which is consistent with the results of other series (31,32). These symptoms are not specific of COVID-19 (31) and, as demonstrated by our results, usually improve over time (33). The reasons why symptoms persist are still unclear. Potentially associated factors include damage to the affected organs, persistent low-grade inflammation, endothelial dysfunction or the presence of viral reservoirs (34) promoted by severe psychological trauma (specially in patients who stayed in the CCU) (35). Thus, post-COVID-19 dyspnea has been associated with hyperventilation syndrome, a form of dysfunctional breathing (36,37). This complication usually occurs in absence of organic respiratory disease and affects patients quality of life. Cough has been suggested to be secondary to activation of vagal sensory nerves (38,39).
PCFS is a recently developed score for assessing functional limitations and status in COVID-19 survivors after hospitalization (18,40). In our study, PCFS improved significantly over time [median 2, (IQR: 1, 2) in the initial visit vs. 0 (IQR: 0, 1) in the final visit; P<0.001). Hence, in the initial visit, 47% of patients [111] had a PCFS ≥2, but only 19.8% maintained this score at 52 weeks [46] (P<0.001). Consistently with our results, the study by Taboada et al. (40) showed that 24.7% of patients obtained a PCFS ≥2 at 6 months. In terms of radiological findings, pulmonary abnormalities were classified as dominantly fibrotic, GGO and with indeterminate course (24). Fibrotic abnormalities are irreversible and have more severe effects. They result from a pathological reconstruction of the alveolar epithelium associated with destruction of normal pulmonary architecture (33,38). In the COMEBAC study, 19.3% of patients had small fibrotic lesions 4 months after hospitalization, with mild deterioration of pulmonary function (38). In our study, only 27 patients (11.6%) had fibrotic lesions. This rate, however, is very likely to be influenced by the timing of measurements, the number of patients that developed ARDS and stayed in CCU in each series and the number of patients who needed OTI. These factors have been associated with a higher risk for fibrotic lesions (38). The percentage of patients with GGO (14.2%) and lesions of indeterminate course (10.3%) in our series was slightly lower than in previous studies. GGO are associated with inflammation of the lung parenchyma and are potentially reversible, although they can be observed 12 months after COVID-19 diagnosis. Indeterminate lesions have a poorly differentiated course profile.
In total, 58.2% of cases had values <80% of the predicted for any pulmonary function parameter. In contrast, the median values of FVC% and FEV1% exceeded that threshold (80%), both at the initial visit and at 1 year. Only the median of baseline DLCO% was <80% of its value of reference both, at the initial visit (65%) and at 52 weeks (77%), although values improved significantly (P<0.001). Consistently, the study by Wu et al. in hospitalized patients who did not need NIV revealed a slight deterioration of DLCO% and FVC% at 3 months [median values (IQR) 77% (67–87%) and 92% (81–99%) of predicted values, respectively]. These parameters improved progressively at 6 months [DLCO 76% (68–90%) and FVC 94% (85–104%)] and at 12 months [DLCO 88% (78–101%) and FVC 98% (89–109%)] (32). In a systematic review of seven articles including 380 patients after hospitalization for SARS-COV-2 infection, the pulmonary function parameter that deteriorated most significantly was DLCO% (41). Our results support the hypothesis that the pulmonary function parameter most severely affected by COVID-19 is DLCO% (38). In this sense, we used the expression “post-COVID-19 fibrotic pulmonary lesions”, which indicates the presence of fibrotic lesions on HRCT with concurrent deterioration of pulmonary function. This concept, which is possibly arbitrary and still poorly defined, defines patients more likely to experience more severe lung injury secondary to SARS-COV-2 infection. The fact that this subgroup of patients only accounts for a small percentage of the total sample (9.4%), along with the fact that patients only experienced a mild deterioration of pulmonary function, suggest that predominantly fibrotic lesions do not cause relevant changes in respiratory function parameters, except for DLCO.
Our prognostic models for the identification of persistent symptoms and patients with a higher risk of developing post-COVID-19 fibrotic pulmonary lesions include five parameters: PCFS score at the initial visit; history of asthma to detect persistent respiratory symptoms; female sex; FVC% at the initial visit and CCU stay. The two models showed a good (AUC 0.857; 95% CI: 0.799–0.915) and excellent (AUC 0.901 95% CI: 0.837–0.964) power of discrimination, respectively. These models can be very useful in clinical practice, as they are easy to use and the variables included are measured in routine laboratory tests. Our models will help physicians determine risk and adopt early follow-up measures. The variables included in the model for persistent respiratory symptoms are associated with high ORs. The same occurs with the variables of the prognostic model for fibrotic pulmonary lesions. Hence, a 1-point increase in baseline FVC% leads to a 6% decrease (OR 0.940) in the probability of developing fibrotic lesions. In addition, CCU stay multiplies by 14 the probability of having these lesions one year after infection. We decided to build two models upon realizing that functional status and history of asthma are enough for the prognosis of persistent respiratory symptoms; in contrast, the prognosis of post-COVID-19 fibrotic pulmonary lesions requires the analysis of a wider variety of variables (factors associated with the deterioration of pulmonary function along with a history of COVID-related CCU stay).
This study has some limitations. Firstly, it is a single-center study without external validation and is based on a small sample of patients. Secondly, the abnormalities observed on HRCT and lung function test cannot be completely attributed to diffuse alveolar damage secondary to infection of the lung parenchyma by SARS-COV-2 and/or subsequent ARDS.
Conclusions
In conclusion, although values prior to SARS-COV-2 infection were not available, the results of this study suggest that a high percentage of patients hospitalized for COVID-19 develop pulmonary lesions though with a low pulmonary function impact. Follow-up these patients is necessary, especially if they have risk factors. Our models identify patients with a high risk of developing persistent respiratory symptoms or post-COVID-19 pulmonary fibrosis with a high accuracy, which could be very useful in clinical practice. Further, longer studies are needed to determine the long-term impact of these complications. External validation of these models is also needed.
Acknowledgments
The authors extend their appreciation to all the study participants.
Funding: None.
Footnote
Reporting Checklist: The authors have completed the STROBE reporting checklist. Available at https://jtd.amegroups.com/article/view/10.21037/jtd-22-1565/rc
Data Sharing Statement: Available at https://jtd.amegroups.com/article/view/10.21037/jtd-22-1565/dss
Peer Review File: Available at https://jtd.amegroups.com/article/view/10.21037/jtd-22-1565/prf
Conflicts of Interest: All authors have completed the ICMJE uniform disclosure form (available at https://jtd.amegroups.com/article/view/10.21037/jtd-22-1565/coif). The authors have no conflicts of interest to declare.
Ethical Statement: The authors are accountable for all aspects of the work in ensuring that questions related to the accuracy or integrity of any part of the work are appropriately investigated and resolved. The study was conducted in accordance with the Declaration of Helsinki (as revised in 2013). The study was approved by the institutional ethics committee of Santiago-Lugo (Registration No. 2020/305) and informed consent was taken from all the patients.
Open Access Statement: This is an Open Access article distributed in accordance with the Creative Commons Attribution-NonCommercial-NoDerivs 4.0 International License (CC BY-NC-ND 4.0), which permits the non-commercial replication and distribution of the article with the strict proviso that no changes or edits are made and the original work is properly cited (including links to both the formal publication through the relevant DOI and the license). See: https://creativecommons.org/licenses/by-nc-nd/4.0/.
References
- Huang C, Wang Y, Li X, et al. Clinical features of patients infected with 2019 novel coronavirus in Wuhan, China. Lancet 2020;395:497-506. [Crossref] [PubMed]
- Lu R, Zhao X, Li J, et al. Genomic characterisation and epidemiology of 2019 novel coronavirus: implications for virus origins and receptor binding. Lancet 2020;395:565-74. [Crossref] [PubMed]
- World Health Organization 2022. Available online: https://covid19.who.int. [Last accessed on 2022 July 10].
- Grasselli G, Pesenti A, Cecconi M. Critical Care Utilization for the COVID-19 Outbreak in Lombardy, Italy: Early Experience and Forecast During an Emergency Response. JAMA 2020;323:1545-6. [Crossref] [PubMed]
- Wang D, Hu B, Hu C, et al. Clinical Characteristics of 138 Hospitalized Patients With 2019 Novel Coronavirus-Infected Pneumonia in Wuhan, China. JAMA 2020;323:1061-9. [Crossref] [PubMed]
- Chen N, Zhou M, Dong X, et al. Epidemiological and clinical characteristics of 99 cases of 2019 novel coronavirus pneumonia in Wuhan, China: a descriptive study. Lancet 2020;395:507-13. [Crossref] [PubMed]
- Guan WJ, Ni ZY, Hu Y, et al. Clinical Characteristics of Coronavirus Disease 2019 in China. N Engl J Med 2020;382:1708-20. [Crossref] [PubMed]
- Chalmers JD, Crichton ML, Goeminne PC, et al. Management of hospitalised adults with coronavirus disease 2019 (COVID-19): a European Respiratory Society living guideline. Eur Respir J 2021;57:2100048. [Crossref] [PubMed]
- Weiss P, Murdoch DR. Clinical course and mortality risk of severe COVID-19. Lancet 2020;395:1014-5. [Crossref] [PubMed]
- Wu C, Chen X, Cai Y, et al. Risk Factors Associated With Acute Respiratory Distress Syndrome and Death in Patients With Coronavirus Disease 2019 Pneumonia in Wuhan, China. JAMA Intern Med 2020;180:934-43. [Crossref] [PubMed]
- Nalbandian A, Sehgal K, Gupta A, et al. Post-acute COVID-19 syndrome. Nat Med 2021;27:601-15. [Crossref] [PubMed]
- Montani D, Savale L, Beurnier A, et al. Multidisciplinary approach for post-acute COVID-19 syndrome: time to break down the walls. Eur Respir J 2021;58:2101090. [Crossref] [PubMed]
- Bai C, Chotirmall SH, Rello J, et al. Updated guidance on the management of COVID-19: from an American Thoracic Society/European Respiratory Society coordinated International Task Force (29 July 2020). Eur Respir Rev 2020;29:200287. [Crossref] [PubMed]
- Unidade multidisciplinar post-COVID. Available online: https://coronavirus.sergas.gal/Contidos/Documents/794/Plan_funcional_Unidad_Multid_PostCovid.pdf
- World Health Organization. Coronavirus disease (COVID-19) technical guidance: laboratory testing for 2019-nCoV in humans. Available online: https://www.who.int/emergencies/diseases/novel-coronavirus-2019/technical-guidance/laboratory-guidance
- Kameda T, Mizuma Y, Taniguchi H, et al. Point-of-care lung ultrasound for the assessment of pneumonia: a narrative review in the COVID-19 era. J Med Ultrason (2001) 2021;48:31-43. [Crossref] [PubMed]
- The ARDS Definition Task Force. Acute Respiratory Distress Syndrome. The Berlin Definition. JAMA 2012;307:2526-33. [PubMed]
- Klok FA, Boon GJAM, Barco S, et al. The Post-COVID-19 Functional Status scale: a tool to measure functional status over time after COVID-19. Eur Respir J 2020;56:2001494. [Crossref] [PubMed]
- Miller MR, Hankinson J, Brusasco V, et al. Standardisation of spirometry. Eur Respir J 2005;26:319-38. [Crossref] [PubMed]
- Wanger J, Clausen JL, Coates A, et al. Standardisation of the measurement of lung volumes. Eur Respir J 2005;26:511-22. [Crossref] [PubMed]
- Roca J, Burgos F, Sunyer J, et al. values for forced spirometry. Group of the European Community Respiratory Health Survey. Eur Respir J 1998;11:1354-62. [Crossref] [PubMed]
- Stocks J, Quanjer PH. Reference values for residual volume, functional residual capacity and total lung capacity. ATS Workshop on Lung Volume Measurements. Official Statement of The European Respiratory Society. Eur Respir J 1995;8:492-506. [Crossref] [PubMed]
- Casanova C, Celli BR, Barria P, et al. The 6-min walk distance in healthy subjects: reference standards from seven countries. Eur Respir J 2011;37:150-6. [Crossref] [PubMed]
- Solomon JJ, Heyman B, Ko JP, et al. CT of Post-Acute Lung Complications of COVID-19. Radiology 2021;301:E383-95. [Crossref] [PubMed]
- Harrell FE. Regression Modeling Strategies: With Applications to Linear Models, Logistic and Ordinal Regression, and Survival Analysis, 2nd edn. New York, NY: Springer International Publishing, 2015.
- Robin X, Turck N, Hainard A, et al. pROC: an open-source package for R and S+ to analyze and compare ROC curves. BMC Bioinformatics 2011;12:77. [Crossref] [PubMed]
- Harrell Jr FE. Regression Modeling Strategies. R package version 6.3-0, 2022. Available online: https://hbiostat.org/doc/rms.pdf
- Wickham H. ggplot2: Elegant graphics for data analysis. Springer-Verlag New York, 2016. ISBN 978-3-319-24277-4.
- R Core Team (2022). R: A language and environment for statistical computing. R Foundation for Statistical Computing, Vienna, Austria. Available online: https://www.R-project.org/
- Moons KG, Altman DG, Reitsma JB, et al. Transparent Reporting of a multivariable prediction model for Individual Prognosis or Diagnosis (TRIPOD): explanation and elaboration. Ann Intern Med 2015;162:W1-73. [Crossref] [PubMed]
- Montani D, Savale L, Noel N, et al. Post-acute COVID-19 syndrome. Eur Respir Rev 2022;31:210185. [Crossref] [PubMed]
- Wu X, Liu X, Zhou Y, et al. 3-month, 6-month, 9-month, and 12-month respiratory outcomes in patients following COVID-19-related hospitalisation: a prospective study. Lancet Respir Med 2021;9:747-54. [Crossref] [PubMed]
- González J, Benítez ID, Carmona P, et al. Pulmonary Function and Radiologic Features in Survivors of Critical COVID-19: A 3-Month Prospective Cohort. Chest 2021;160:187-98. [Crossref] [PubMed]
- Ren X, Wen W, Fan X, et al. COVID-19 immune features revealed by a large-scale single-cell transcriptome atlas. Cell 2021;184:1895-1913.e19. [Crossref] [PubMed]
- Hancox RJ, Morgan J, Dickson N, et al. Rape, asthma and dysfunctional breathing. Eur Respir J 2020;55:1902455. [Crossref] [PubMed]
- George PM, Barratt SL, Condliffe R, et al. Respiratory follow-up of patients with COVID-19 pneumonia. Thorax 2020;75:1009-16. [Crossref] [PubMed]
- Chenivesse C, Similowski T, Bautin N, et al. Severely impaired health-related quality of life in chronic hyperventilation patients: exploratory data. Respir Med 2014;108:517-23. [Crossref] [PubMed]
- Morin L, Savale L, et al. Four-Month Clinical Status of a Cohort of Patients After Hospitalization for COVID-19. JAMA 2021;325:1525-34. [Crossref] [PubMed]
- Song WJ, Hui CKM, Hull JH, et al. Confronting COVID-19-associated cough and the post-COVID syndrome: role of viral neurotropism, neuroinflammation, and neuroimmune responses. Lancet Respir Med 2021;9:533-44. [Crossref] [PubMed]
- Taboada M, Cariñena A, Moreno E, et al. Post-COVID-19 functional status six-months after hospitalization. J Infect 2021;82:e31-3. [Crossref] [PubMed]
- Torres-Castro R, Vasconcello-Castillo L, Alsina-Restoy X, et al. Respiratory function in patients post-infection by COVID-19: a systematic review and meta-analysis. Pulmonology 2021;27:328-37. [Crossref] [PubMed]