IRF7 and IFIT2 in mediating different hemorrhage outcomes for non-small cell lung cancer after bevacizumab treatment
Highlight box
Key findings
• The microvessel density (MVD) of lung adenocarcinoma (LUAD) tissues was higher than that of lung squamous cell carcinoma (LUSC) tissues. Higher IRF7 levels and lower IFIT2 levels in LUAD tumors were associated with a higher MVD in LUAD tissues, which may be responsible for the different hemorrhage outcomes after bevacizumab treatment.
What is known and what is new?
• Significant clinical differences have been observed between LUAD and LUSC after bevacizumab treatment.
• IRF7 and IFIT2 may account for different hemorrhage outcomes in patients with non-small cell lung cancer.
What is the implication, and what should change now?
• The substantial hemoptysis caused by bevacizumab treatment can be prevented via targeting IRF7 and IFIT2 in patients with LUSC.
Introduction
Lung cancer is among the cancers with the highest morbidity and mortality in the world (1). There are approximately 2 million new lung cancer cases and 1.76 million lung cancer deaths per year. Lung cancers can be classified as non-small cell lung cancer (NSCLC; 85% of total diagnoses) and small cell lung cancer (SCLC; 15% of total diagnoses) (2). However, approximately 70% of patients with NSCLC are already in advanced disease stages at the time of their first diagnosis, and these patients have a poor 5-year survival, with only 10–20% surviving past this period (3).
Traditional radiation therapy and chemotherapy strategies are usually unable to meet therapeutic expectations (4). In recent years, molecular targeted therapy and immunotherapy have substantially improved patient survival (5-9). Bevacizumab, a recombinant humanized anti-vascular endothelial growth factor (VEGF) monoclonal antibody, is one of the most important targeted drugs for a range of advanced cancers with poor prognosis, including NSCLC (10,11). However, in a phase II clinical study, Margolin et al. found that bevacizumab treatment in patients with advanced NSCLC caused a substantial adverse reaction in the form of bleeding, which was more common in patients with lung squamous cell carcinoma (LUSC) than in those with lung adenocarcinoma (LUAD) (12); furthermore, based on the pivotal E4599 study, bevacizumab has only been approved for patients with nonsquamous NSCLC (13).
Previous studies have suggested that hemoptysis caused by bevacizumab is related to anatomical characteristics. For example, LUSC is commonly thought to occur in the central airway, in which the pulmonary artery or branches are prone to rupture, with a high probability of substantial hemoptysis when tumors invade (14). However, in a prospective phase II trial involving patients with lung cancers at high risk of hemoptysis, Hellmann et al. evaluated the safety of adjuvant bevacizumab following neoadjuvant chemotherapy and complete surgical resection. They observed no instances of hemoptysis of any grade in patients with central tumors (15). Another study similarly found that no clinical or radiological features (including cavitation and central tumor location) could be used to reliably predict severe pulmonary hemorrhage in bevacizumab-treated patients (16). In a retrospective study involving 536 patients with pulmonary malignancy, Salajka et al. reported that the incidence of hemoptysis was similar between patients with central tumors and those with peripheral tumors. They also suggested that the occurrence of bleeding was related to the histological type of tumor and the tumor size (17). However, in a recent case-control study of risk factors for hemoptysis, multivariate analysis revealed that prior thoracic radiotherapy, the presence of tumor exposure in the central airway, and concomitant radiotherapy were risk factors for hemoptysis (18). This finding suggests that the substantial hemoptysis that occurs during bevacizumab treatment is not simply related to anatomical factors.
However, the reasons for the substantial clinical differences between patients with LUSC and those with LUAD receiving bevacizumab treatment are unclear. Additionally, there is no consensus on the correlation between microvessel density (MVD) and bleeding. Based on the evaluation of MVD, Iordache et al. found a clear correlation between angiogenic parameters and the risk of upper gastrointestinal bleeding in patients with gastric cancer (19). In contrast, some scholars believe that tumors with sufficient MVD do not exhibit activation of the hypoxia-induced signaling pathway and remain sensitive to chemotherapy and radiotherapy. The lack of involvement of such signaling pathways may lead to reduced tumor invasiveness. A low MVD is associated with higher tumor aggressiveness, resulting in higher levels of vascular infiltration and hemorrhage (20,21). In this study, we found that LUAD tumors had a higher MVD than did LUSC tumors. No significant difference in VEGF expression was observed between LUSC- and LUAD-associated endothelial cells. In addition, we found that there was strong heterogeneity between LUAD and LUSC endothelial cells. Moreover, IFIT2 and IRF7 were highly enriched in epithelial cells of NSCLC tumors, and these two genes may underlie the differential hemorrhage outcomes in patients with NSCLC after bevacizumab treatment. These findings may provide new insights into the causes underlying bevacizumab-induced pulmonary hemoptysis. We present the following article in accordance with the MDAR and ARRIVE reporting checklists (available at https://jtd.amegroups.com/article/view/10.21037/jtd-23-389/rc).
Methods
Patients
Tumor tissues from 18 patients with LUSC and 18 patients with LUAD were obtained from the Affiliated Hospital of Jiangnan University (Wuxi, China). The pathophysiological information is displayed in Table 1. The study was approved by the Clinical Research Ethics Committees of Affiliated Hospital of Jiangnan University (No. LS2021019), and written informed consent was obtained from all patients. The study was conducted in accordance with the Declaration of Helsinki (as revised in 2013).
Table 1
Variable | Total cohort (n=36) |
---|---|
Age (years) | 62.9±7.9 |
Sex | |
Male | 27 (75.0) |
Female | 9 (25.0) |
Pathology | |
LUSC | 18 (50.0) |
LUAD | 18 (50.0) |
Differentiation | |
Low | 14 (38.9) |
Moderate | 18 (50.0) |
Well | 4 (11.1) |
Stage | |
I | 8 (22.2) |
II | 14 (38.9) |
III | 12 (33.3) |
IV | 2 (5.6) |
TNM | |
T1 | 13 (36.1) |
T2 | 13 (36.1) |
T3 | 7 (19.5) |
T4 | 3 (8.3) |
N0 | 22 (61.1) |
N1 | 5 (13.9) |
N2 | 9 (25.0) |
M0 | 34 (94.4) |
M1 | 2 (5.6) |
Data are expressed as number of patients (percentage) or mean ± SEM. LUAD, lung adenocarcinoma; LUSC, lung squamous cell carcinoma; SD, standard deviation; TNM, tumor-node-metastasis.
Cell culture
All cells were grown at 37 ℃ with 5% CO2 in a humidified environment. HMEC-1 cells were grown in extracellular matrix (ECM). A549 cells and H358 cells were grown in high glucose Dulbecco’s Modified Eagle Medium (DMEM) with 10% fetal bovine serum (FBS) and 1% penicillin/streptomycin. H2170 cells, H1703 cells, H1299 cells, and H226 cells were grown in RPMI 1640 with 10% FBS and 1% penicillin/streptomycin. Cells were confirmed to be mycoplasma free and were passaged no more than 18 to 25 times after thawing.
Immunohistochemistry
Tissue samples were fixed by immersion in 4% paraformaldehyde overnight at 4 ℃, embedded in regular paraffin wax, and cut into 4-µm sections. Sections were boiled in sodium citrate buffer (pH 6.0) for antigen retrieval, and endogenous peroxidase activity was blocked via incubation with 0.3% hydrogen peroxide. Sections were washed with 10% phosphate-buffered saline (PBS) and marked with a peroxidase-antiperoxidase (PAP) pen. After being blocked with 10% goat serum, the sections were incubated with primary antibodies against CD31 and CD34 for 12 h at 4 ℃. Finally, the samples were incubated with peroxidase-conjugated streptavidin. The images were developed by incubating the slides for several minutes with diaminobenzidine, and nuclei were counterstained with hematoxylin. The subsequent steps were performed using the EnVision FLEX High pH 9.0 Visualization System (Dako). All slides were independently evaluated by 2 pathologists. Microvessels clearly separated from adjacent brown-staining endothelial cells or endothelial cell clusters, tumor cells, and other connective-tissue elements were considered single, countable microvessels. We took photos and assessed the 3 areas with the highest MVD in each section under a 100× field of vision. All counts were performed simultaneously by 2 investigators (Y Yin and L Huang) using a light microscope, and both had to agree on what constituted a single microvessel before any vessel was included in the count.
Total RNA extraction and quantitative real-time polymerase chain reaction
Total RNA was extracted from cells using TRIzol reagent. Complement DNA (cDNA) was synthesized with a PrimeScript RT Reagent KIT (Vazyme China), quantitative real-time polymerase chain reaction (qRT-PCR) analyses were carried out to detect messenger RNA (mRNA) expression using SYBR Premix Ex Taq (Cwbio China), and β-actin was used as an internal control. Primer sequences are shown in Table 2.
Table 2
Gene | Forward primer (5'-3') | Reverse primer (5'-3') |
---|---|---|
VEGF | GAGGAGCAGTTACGGTCTGTG | TCCTTTCCTTAGCTGACACTTGT |
VEGFA | GAGGGCAGAATCATCACGA | TGTGCTGTAGGAAGCTCATCTC |
ISG15 | CGCAGATCACCCAGAAGATCG | TTCGTCGCATTTGTCCACCA |
LY6E | CAGCTCGCTGATGTGCTTCT | CAGACACAGTCACGCAGTAGT |
IFI35 | AACAAAAGGAGCACACGATCA | CTCCGTTCCTAGTCTTGCCAA |
TXNIP | GGTCTTTAACGACCCTGAAAAGG | ACACGAGTAACTTCACACACCT |
IFIT2 | AAGCACCTCAAAGGGCAAAAC | TCGGCCCATGTGATAGTAGAC |
IRF7 | ATATCTCACGTGACCGAGGA | AGCTGATGGTGCTGGAAGTC |
S100A8 | ATGCCGTCTACAGGGATGAC | ACTGAGGACACTCGGTCTCTA |
β-actin | AGTGTGACGTGGACATCCGCAAAG | ATCCACATCTGCTGGAAGGTGGAC |
VEGF, vascular endothelial growth factor; VEGFA, vascular endothelial growth factor A; ISG15, interferon stimulated gene 15; LY6E, lymphocyte antigen 6; IFI35, interferon-induced protein 35; TXNIP, thioredoxin interacting protein; IFIT2, interferon-induced protein with tetratricopeptide repeats 2; IRF7, interferon regulatory factor 7; S100A8, S100 calcium binding protein A8.
Western blot analyses
Proteins were separated on a 10% sodium dodecyl-sulfate polyacrylamide gel electrophoresis (SDS-PAGE) gel and then transferred to polyvinylidene fluoride (PVDF) membranes. After being blocked with 5% nonfat milk, the membrane was incubated with mouse anti-VEGF and rabbit β-actin monoclonal antibodies. A peroxidase-conjugated anti-rabbit antibody was used as the secondary antibody, and the membranes were visualized with enhanced chemiluminescence.
Enzyme-linked immunosorbent assay
A549, H1299, H358, H2170, H226 and H1703 cells (1×105) were resuspended and plated in 24-well plates with 300 µL of complete medium, and the supernatant was collected after 3 days. Enzyme-linked immunosorbent assays (ELISA) were performed to evaluate the expression level of VEGF using ELISA kits (mlBio China) according to the manufacturer’s instructions.
Immunofluorescence
Cell slides were placed in 6-well plates and seeded with the appropriate amount ofA549, H1299, H2170 and H226 cells overnight. After different treatments, the cells were washed 3 times with PBS, fixed with 4% paraformaldehyde for 20 minutes, and then blocked with 10% normal goat serum in PBS for 30 minutes at room temperature (RT). The next day, the cells were incubated with fluorescent secondary antibody for 1 hour at RT, stained with DAPI-containing mounting medium, and finally observed with a fluorescence microscope (#BX53F; Olympus).
Tube formation assay
Ice-cold phenol red Matrigel (300 µL) was added to a 24-well plate using a chilled pipet tip and incubated for 3 hours at 37 ℃. HMEC-1 cells were cocultured with tumor cells for 72 hours. After coculture, the cells were washed twice with PBS and resuspended to 1×105/mL with serum-free ECM medium in a 24-well plate. The cells were observed for 24 hours. Capillary tube formation was quantified by counting the number of pixels from 3 randomly selected fields in each well under microscopy (100×).
Angiogenesis in vivo
A total of 16 six-week-old BALB/c male mice were purchased from the Shanghai Laboratory Animal Center, kept in a constant temperature specific pathogen-free (SPF) environment, and randomly divided evenly into 2 groups. All animal experiments were performed under a project license (No. 20211115c0240130) granted by committee board of Jiangnan University, in compliance with Jiangsu province guidelines for the care and use of animals. A protocol was prepared before the study without registration. A total of 4×106 H2170 or H1299 cells and 1×105 HMEC-1 cells were mixed 1:1 with Matrigel and supplemented with 30 units of heparin and 150 ng of basic fibroblast growth factor (bFGF) in a total volume of 0.5 mL and then subcutaneously injected into both flanks of mice. Two weeks later, when the tumor had grown to 1 cm3, the mice were killed for data collection. The tumors were fixed with 4% paraformaldehyde, embedded in paraffin, sectioned, and then stained with hematoxylin and eosin. For quantitative analysis, the MVD in the tumor was counted in 3 independent fields from 3 sections (22).
Quality control, cell-type clustering, and major cell-type identification
We obtained single-ell sequencing data from lung cancer tissues according to a recent study (23). A total of 42 samples, including 22 LUSC samples, 18 LUAD samples, and 2 NSCLC samples, were examined in this study. Finally, data acquired from 90,406 cells were obtained for downstream analysis. To assign 1 of the 9 major cell types to each cluster, we scored each cluster according to the normalized expression of its canonical markers, and the highest scored cell type was assigned to each cluster. Cancer cell clusters were negative for normal lung epithelial markers and positive for epithelial cellular adhesion molecule (EpCAM). The clusters assigned to the same cell type were grouped together for the subsequent analysis. Through preliminary exploratory detection of differentially expressed genes (DEGs) in each cell cluster, combined with literature research, 9 major cell types were selected, and the DEGs were identified using the FindMarkers function in the “Seurat” package of R software (The Foundation for Statistical Computing).
Functional enrichment analyses
The following cutoff threshold values were used to screen for the DEGs: adjusted P value <0.01, percentage difference >10, and average log fold change (FC) >0.5. Gene set enrichment analysis (GSEA) was used to detect which gene set was significantly enriched in each specific cell cluster. Only gene sets with false-discovery rate (FDR) P values <0.05 were assessed.
Transfection
The following duplex small interfering RNAs (siRNAs) were purchased from RiboBio: si-IRF7-1: 5'-GAGC TGCACGTTCCTATA-3'; si-IRF7-2: 5'-TCGAGTGCTTCCTTATGGA-3'; si-IFIT2-1: 5'-GACTGGCAATAGCAAGCTA-3'; si-IFIT2-2: 5'-GCAACCTACTGGCCTATCT-3. 1×106 HMEC-1 cells were seeded in 6-well culture plates, and siRNAs were transfected into cells using Lipofectamine 2000 (Thermo America) according to the manufacturer’s instructions on the next day.
Statistical analyses
GraphPad Prism 8.0 software (GraphPad Software Inc.) was used to test data with a normal distribution, and the homogeneity of variance was determined. The data are expressed as the mean ± the standard error of the mean (SEM) and were analyzed with Student t test (2 groups) or 1-way analysis of variance (ANOVA) with Tukey post hoc test (more than 2 groups). The data with skewed distributions were tested using nonparametric tests. The results with a P value <0.05 were considered statistically significant.
Results
LUAD tumors had a higher MVD than did LUSC tumors
Bevacizumab plays a pivotal role in the treatment of advanced lung cancer, but in patients with LUSC, it often causes substantial hemoptysis. Since bevacizumab directly targets VEGF to inhibit tumor angiogenesis, we compared the differences in MVD between LUAD and LUSC by staining CD31 and CD34 (Figure 1A,1B). We found that LUAD tumors (CD31: mean ± SEM =18.44±8.33; CD34: mean ± SEM =22.89±12.49) had a higher MVD than did LUSC tumors (CD31: mean ± SEM =11.78±6.70; CD34: mean ± SEM =10.89±5.44) (CD31: P=0.0143; CD43: P<0.0001) (Figure 1C,1D), suggesting that a higher MVD may contribute to less hemorrhage in certain clinical situations.
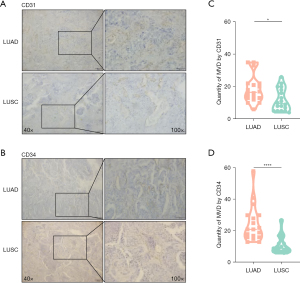
LUAD cells promoted angiogenesis more effectively than did LUSC cells
To further investigate the effect of LUAD and LUSC on angiogenic morphology, we used a tube formation assay in which capillary-like tubular structures resembling microvascular networks could be observed on Matrigel. We first assessed the effects of LUSC and LUAD cells on the capacity of HMEC-1 cells to form tubes using a coculture assay. As expected, tube formation assays showed that LUAD cells could promote endothelial cells to form more capillary tubes than could LUSC cells (LUAD: mean ± SEM =30.33±0.47; LUSC: mean ± SEM =12.33±1.25; Figure 2). Furthermore, we performed in vivo experiments by subcutaneously injecting a mixed solution containing H1299 and H2170 cells, endothelial cells, Matrigel, and various growth factors into BALB/c mice. Consistent with the in vitro experiments, tumors injected with LUAD cells showed a higher MVD than did those injected with LUSC cells (LUAD: mean ± SEM =19.85±0.31; LUSC: mean ± SEM =5.12±0.27 for LUSC; P<0.01; Figure 2).
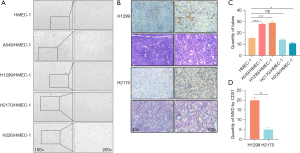
Expression of VEGF was not significantly different between LUSC and LUAD cells
We next explored the reasons for the difference in MVD between the LUSC and LUAD tumors. VEGF is currently recognized as one of the most relevant targets for angiogenesis, and bevacizumab is an antagonist of VEGF. We analyzed whether the difference in MVD was caused by the different levels of VEGF secreted from tumor cells after acting on endothelial cells. Thus, we first detected the expression of VEGF in A549, H1299, H358, H2170, H226 and H1703 cells using Western blotting (Figure 3A), qRT-PCR (Figure 3B), ELISA (Figure 3C), and immunofluorescence (Figure 3D). We found that there was no significant difference in the expression of VEGF between LUSC and LUAD cells. These results suggested that VEGF expression in NSCLC tumor cells has no effect on the difference in angiogenesis.
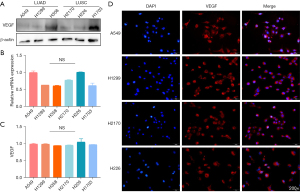
Bioinformatic analysis indicated the heterogeneity of gene expression between LUAD and LUSC
We next investigated the DEGs in endothelial cells from LUAD and LUSC tumors. Lung cancer single-cell sequencing data were obtained from a recent study (23). The 42 samples in this study included 22 LUAD, 18 LUSC and 2 NSCLC samples with accurately defined pathological types. After the final quality control, we obtained data regarding 24,666 LUAD cells, 57,774 LUSC cells. And 7447 other types (Figure 4A). We clustered these cells into 9 groups according to their marker genes, including clusters of immune cells, stromal cells, and epithelial cells (Figure 4B,4C). The results showed that there was a high degree of heterogeneity between LUAD and LUSC in the composition of cells in the tumor microenvironment, especially malignant epithelial cells and endothelial cells. Next, we identified the DEGs in malignant epithelial cells and endothelial cells in LUAD and LUSC tumors. Furthermore, the GSEA results based on the 3 data sets suggested that many signaling pathways related to angiogenesis were significantly enriched (Figure 4D,4E), including the interferon-α response, KRAS signaling up, and P53 signaling pathways. Finally, we analyzed the signaling pathway between LUSC and LUAD through the DEGs (Figure 4F,4G), the data showed that there were significant differences in signaling pathways between the two groups.
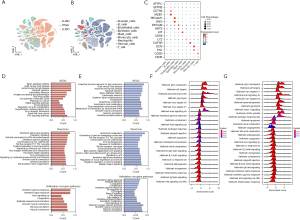
IRF7 and IFIT2 were differentially expressed in LUSC and LUAD cells
We subsequently searched for the DEGs involved in these pathways according to the frequency of occurrence in each pathway, and in combination with a review of the literature, the results indicated the angiogenesis-related genes for subsequent analyses. The selected genes are shown in Figure 5A. To further verify these candidate genes in tumor endothelial cells, we cocultured LUAD and LUSC cell lines with HMEC-1 cells and verified that interferon regulatory factor 7 (IRF7) was upregulated in endothelial cells cocultured with LUAD cells and that interferoninduced protein with tetratricopeptide repeats 2 (IFIT2) was upregulated in endothelial cells cocultured with LUSC cells (Figure 5A). A volcano plot also showed that among these candidate genes, IFIT2 and IRF7 were enriched in the epithelial cells of NSCLC tumors. These results suggested that the 2 genes may play an important role in angiogenesis (Figure 5B,5C). Therefore, we focused on these 2 genes in the subsequent investigations.
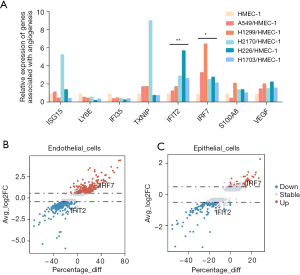
IRF7 and IFIT2 regulated the tube formation of HMEC-1 cells
IRF7 is the most important nuclear transcription factors regulating type-I interferon (IFN) production in mammals (24). IFIT2 is an interferon-stimulated gene with well-established antiviral activity but limited mechanistic understanding (25). In order to determine whether the microvascular differences between LUAD and LUSC were caused by IRF7 and IFIT2, we used 2 small interference sequences to knockdown IRF7 and IFIT2 (Figure 6A,6B). Then, HMEC-1 cells transfected with these small interferences were spread on Matrigel for ring formation evaluation (Figure 6C). We found that IRF7 knockdown significantly inhibited the ring formation of HMEC-1 cells, whereas IFIT2 knockdown promoted the ring formation of HMEC-1 cells. Taken together, these data suggest that the difference in the expression of IRF7 and IFIT2 in the microvasculature leads to the difference in MVD between the LUSC and LUAD tumors.
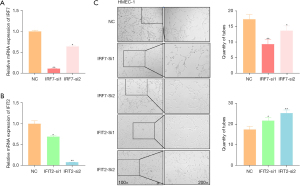
Bevacizumab promoted the effects of IRF7 and IFIT2 on angiogenesis
To explore whether bevacizumab regulates the expression of IRF7 and IFIT2 in endothelial cells as well as the angiogenesis of lung cancer cells, bevacizumab was added to HMEC-1 cells cocultured with H1299 and H2170 cells. As shown in Figure 7A, after bevacizumab treatment (26), HMEC-1 cells cocultured with H1299 cells formed more rings than did those cocultured with H2170 cells (H1299 cell culture mean ± SEM =22.33±1.25; H2170 culture: mean ± SEM =9.67±1.70; P=0.03). Although the expression of VEGF and VEGFA in HMEC-1 cells was downregulated, there were no statistically significant differences between the 2 groups (Figure 7B,7C). Interestingly, after the addition of bevacizumab, IRF7 and IFIT2 levels in HMEC-1 cells cocultured with H1299 and H2170 cells changed even more strikingly than did those in the noncoculture group (Figure 7D,7E). We speculate that bevacizumab could further enhance the effect of these 2 genes on angiogenesis.
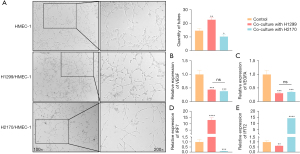
Discussion
In recent years, the prevalence of lung cancer has increased rapidly in China (1,27), and traditional radiotherapy and chemotherapy have been unable to meet treatment expectations. The emergence of bevacizumab has provided a new treatment strategy for lung cancer. Several studies (28,29) have shown that bevacizumab-based therapy may lead to longer survival and significantly prolonged disease remission in patients with advanced NSCLC. However, patients with LUSC are prone to massive hemoptysis during bevacizumab treatment. Due to this serious side effect, bevacizumab has limited use in patients with LUSC. In this study, we explored the causes underlying the differences in hemoptysis in patients with LUSC and LUAD after bevacizumab treatment.
First, we found that LUSC tumors showed lower angiogenesis than LUAD tumors, which was further confirmed by in vitro and in vivo experiments. VEGF is a key regulator of angiogenesis. Therefore, we aimed to explore the differences in VEGF expression between LUSC and LUAD tumors, but no significant difference was observed.
We then downloaded single-cell sequencing data obtained from patients with NSCLC and investigated the pathways and genes related to angiogenesis. The results, combined with the experimental verification, indicated that IRF7 was highly expressed in the endothelial cells of LUAD tumors and that IFIT2 was highly expressed in the endothelial cells of LUSC tumors. Simons et al. reported that IRF3 and IRF7 mediate neoangiogenesis through inflammatory cytokines (30). Lai et al. showed that IFIT2 depletion promotes angiogenesis in metastatic oral squamous cell carcinoma cells (31). These results, all of which are consistent with the observations of study, suggest that IRF7 promotes angiogenesis and that IFIT2 inhibits angiogenesis. Our functional evaluations also showed that IRF7 knockdown reduced the number of vascular loops and decreased lumen integrity, whereas knockdown of IFIT2 increased the number of vascular loops and improved lumen integrity. Moreover, bevacizumab treatment further promoted the effects of IRF7 and IFIIT2 on angiogenesis in lung cancer. Taken together, these results suggest that the difference in the expression of IRF7 and IFIT2 may affect the angiogenesis of LUSC and LUAD tumors.
Investigations of patients with LUSC who are prone to massive hemoptysis after treatment with bevacizumab have mainly focused on macroscopic aspects such as anatomy. In this study, for the first time, we explored the DRGs in tumor endothelial cells in patients with LUSC and LUAD using single-cell sequencing analysis. A differential expression of IRF7 and IFIT2 was observed in LUSC and LUAD cells and was associated with low MVD in LUSC tissue and less blood supply inside the tumor. It is generally believed that MVD is positively correlated with the risk of bleeding. Dansin et al. collected the clinical data of 222 patients with NSCLC treated with bevacizumab to explore potential associations between bleeding events and baseline and disease characteristics. It was found that the overall bleeding rate of noncentral tumors was higher than that of central lung cancer, whereas the incidence of bleeding grade ≥3 was lower (32). According to the 2004 World Health Organization (WHO) classification, LUSC is mainly a central-type lung cancer that occurs in the trachea and bronchus, and approximately 65% of LUAD cases are the peripheral type (33). Synthesizing these finding’s with our results, we hypothesized that due to the differential expression of IRF7 and IFIT2, LUAD has a higher MVD and overall bleeding risk. In addition, due to the abundant blood supply, there is a lower risk of intratumoral cavitation and fatal hemorrhage.
Moreover, some researchers believe that tumors with sufficient MVD do not exhibit activation of hypoxia-induced signaling pathways; therefore, they remain sensitive to chemotherapy and radiotherapy, and the lack of such signaling may lead to reduced tumor invasiveness. Similarly, low MVD is associated with higher tumor aggressiveness, which causes a greater degree of vascular infiltration and leads to bleeding. Our study suggests that the difference in the expression of IRF7 and IFIT2 in the endothelium of LUSC and LUAD tumors causes the difference in MVD between these 2 tumor types. After bevacizumab treatment, the effect of IRF7 and IFIT2 on angiogenesis is further amplified, reducing tumor angiogenesis in LUSC, decreasing vascular stability, increasing tissue hypoxia, and increasing the incidence of hemoptysis.
In this study, we identified the differential expression of IRF7 and IFIT2 in the endothelium of LUSC and LUAD tumors and showed that bevacizumab may affect angiogenesis by enhancing the effect of IRF7 and IFIT2. However, the molecular mechanisms by which IRF7 and IFIT2 participate in and regulate angiogenesis-related pathways and mediate angiogenesis after bevacizumab treatment remain unclear. Our findings clarify the important roles of IRF7 and IFIT2 in angiogenesis-related pathways. Thus, we can prevent the substantial hemoptysis caused by bevacizumab treatment by targeting the levels of IRF7 and IFIT2 in patients with LUSC. Overall, this study can help improve clinical treatment strategies for these patients.
Conclusions
The MVD of LUAD tissues was higher than that of LUSC tissues. Higher IRF7 levels and lower IFIT2 levels in LUAD tumors were associated with a higher MVD in LUAD tissues, which may be responsible for the different hemorrhage outcomes after bevacizumab treatment.
Acknowledgments
Funding: This work was supported by National Natural Science Foundation of China (No. 82173193); the Natural Science Foundation of Jiangsu Province (No. BK20211033); Science and Technology Demonstration Project of Social Development of Jiangsu Province (No. BE2019631); the Double Hundred Medical Professionals Program of Wuxi (Nos. BJ2020053 and BJ2020058); the Wuxi Taihu Lake Talent Plan, Wuxi Medical Key Discipline (No. ZDXK2021002); and the Project of State Key Laboratory of Radiation Medicine and Protection, Soochow University (No. GZK1202202).
Footnote
Reporting Checklist: The authors have completed the MDAR and ARRIVE reporting checklists. Available at https://jtd.amegroups.com/article/view/10.21037/jtd-23-389/rc
Data Sharing Statement: Available at https://jtd.amegroups.com/article/view/10.21037/jtd-23-389/dss
Peer Review File: Available at https://jtd.amegroups.com/article/view/10.21037/jtd-23-389/prf
Conflicts of Interest: All authors have completed the ICMJE uniform disclosure form (available at https://jtd.amegroups.com/article/view/10.21037/jtd-23-389/coif). The authors have no conflicts of interest to declare.
Ethical Statement: The authors are accountable for all aspects of the work in ensuring that questions related to the accuracy or integrity of any part of the work are appropriately investigated and resolved. The study was approved by the Clinical Research Ethics Committees of Affiliated Hospital of Jiangnan University (No. LS2021019), and written informed consent was obtained from all patients. The study was conducted in accordance with the Declaration of Helsinki (as revised in 2013). All animal experiments were performed under a project license (No. 20211115c0240130) granted by committee board of Jiangnan University, in compliance with Jiangsu province guidelines for the care and use of animals. A protocol was prepared before the study without registration.
Open Access Statement: This is an Open Access article distributed in accordance with the Creative Commons Attribution-NonCommercial-NoDerivs 4.0 International License (CC BY-NC-ND 4.0), which permits the non-commercial replication and distribution of the article with the strict proviso that no changes or edits are made and the original work is properly cited (including links to both the formal publication through the relevant DOI and the license). See: https://creativecommons.org/licenses/by-nc-nd/4.0/.
References
- Sung H, Ferlay J, Siegel RL, et al. Global Cancer Statistics 2020: GLOBOCAN Estimates of Incidence and Mortality Worldwide for 36 Cancers in 185 Countries. CA Cancer J Clin 2021;71:209-49. [Crossref] [PubMed]
- Thai AA, Solomon BJ, Sequist LV, et al. Lung cancer. Lancet 2021;398:535-54. [Crossref] [PubMed]
- Bogos K, Kiss Z, Tamási L, et al. Improvement in lung cancer survival: Six-year survival analysis of patients diagnosed between 2011 and 2016 in Hungary. J Clin Oncol 2020;38:e21582. [Crossref]
- Johnson DH, Fehrenbacher L, Novotny WF, et al. Randomized phase II trial comparing bevacizumab plus carboplatin and paclitaxel with carboplatin and paclitaxel alone in previously untreated locally advanced or metastatic non-small-cell lung cancer. J Clin Oncol 2004;22:2184-91. [Crossref] [PubMed]
- Tan AC, Tan DSW. Targeted Therapies for Lung Cancer Patients With Oncogenic Driver Molecular Alterations. J Clin Oncol 2022;40:611-25. [Crossref] [PubMed]
- Mayekar MK, Bivona TG. Current Landscape of Targeted Therapy in Lung Cancer. Clin Pharmacol Ther 2017;102:757-64. [Crossref] [PubMed]
- Hou X, Zhou C, Wu G, et al. Efficacy, Safety, and Health-Related Quality of Life With Camrelizumab Plus Pemetrexed and Carboplatin as First-Line Treatment for Advanced Nonsquamous NSCLC With Brain Metastases (CAP-BRAIN): A Multicenter, Open-Label, Single-Arm, Phase 2 Study. J Thorac Oncol. 2023 Feb 2:S1556-0864(23)00092-8. [Epub ahead of print]. doi:
10.1016/j.jtho.2023.01.083 .10.1016/j.jtho.2023.01.083 - Yu Y, Yang Y, Li H, et al. Targeting HER2 alterations in non-small cell lung cancer: Therapeutic breakthrough and challenges. Cancer Treat Rev 2023;114:102520. [Crossref] [PubMed]
- Breen WG, Leventakos K, Dong H, et al. Radiation and immunotherapy: emerging mechanisms of synergy. J Thorac Dis 2020;12:7011-23. [Crossref] [PubMed]
- Zhao Z, Zhao L, Xia G, et al. Efficacy and safety of bevacizumab biosimilar compared with reference bevacizumab in locally advanced and advanced non-small cell lung cancer patients: A retrospective study. Front Oncol 2022;12:1036906. [Crossref] [PubMed]
- Long J, Lei S, Wu Z, et al. Efficacy and safety of original EGFR-TKI combined with bevacizumab in advanced lung adenocarcinoma patients harboring EGFR-mutation experiencing gradual progression after EGFR-TKI treatment: a single-arm study. Ann Transl Med 2022;10:1334. [Crossref] [PubMed]
- Margolin K, Gordon MS, Holmgren E, et al. Phase Ib trial of intravenous recombinant humanized monoclonal antibody to vascular endothelial growth factor in combination with chemotherapy in patients with advanced cancer: pharmacologic and long-term safety data. J Clin Oncol 2001;19:851-6. [Crossref] [PubMed]
- Sandler A, Gray R, Perry MC, et al. Paclitaxel-carboplatin alone or with bevacizumab for non-small-cell lung cancer. N Engl J Med 2006;355:2542-50. [Crossref] [PubMed]
- Jin F, Li Q, Bai C, et al. Chinese Expert Recommendation for Diagnosis and Treatment of Massive Hemoptysis. Respiration 2020;99:83-92. [Crossref] [PubMed]
- Hellmann MD, Chaft JE, Rusch V, et al. Risk of hemoptysis in patients with resected squamous cell and other high-risk lung cancers treated with adjuvant bevacizumab. Cancer Chemother Pharmacol 2013;72:453-61. [Crossref] [PubMed]
- Reck M, Barlesi F, Crinò L, et al. Predicting and managing the risk of pulmonary haemorrhage in patients with NSCLC treated with bevacizumab: a consensus report from a panel of experts. Ann Oncol 2012;23:1111-20. [Crossref] [PubMed]
- Salajka F. Occurrence of haemoptysis in patients with newly diagnosed lung malignancy. Schweiz Med Wochenschr 1999;129:1487-91. [PubMed]
- Goto K, Endo M, Kusumoto M, et al. Bevacizumab for non-small-cell lung cancer: A nested case control study of risk factors for hemoptysis. Cancer Sci 2016;107:1837-42. [Crossref] [PubMed]
- Iordache S, Saftoiu A, Georgescu CV, et al. Vascular endothelial growth factor expression and microvessel density--two useful tools for the assessment of prognosis and survival in gastric cancer patients. J Gastrointestin Liver Dis 2010;19:135-9. [PubMed]
- Pomme G, Augustin F, Fiegl M, et al. Detailed assessment of microvasculature markers in non-small cell lung cancer reveals potentially clinically relevant characteristics. Virchows Arch 2015;467:55-66. [Crossref] [PubMed]
- Hu P, Wang G, Cao H, et al. Haemoptysis as a prognostic factor in lung adenocarcinoma after curative resection. Br J Cancer 2013;109:1609-17. [Crossref] [PubMed]
- Li J, Zhang Y, Liu Y, et al. Microvesicle-mediated transfer of microRNA-150 from monocytes to endothelial cells promotes angiogenesis. J Biol Chem 2013;288:23586-96. [Crossref] [PubMed]
- Wu F, Fan J, He Y, et al. Single-cell profiling of tumor heterogeneity and the microenvironment in advanced non-small cell lung cancer. Nat Commun 2021;12:2540. [Crossref] [PubMed]
- Lin Z, Wang J, Zhao S, et al. Goose IRF7 is involved in antivirus innate immunity by mediating IFN activation. Dev Comp Immunol 2022;133:104435. [Crossref] [PubMed]
- Tran V, Ledwith MP, Thamamongood T, et al. Influenza virus repurposes the antiviral protein IFIT2 to promote translation of viral mRNAs. Nat Microbiol 2020;5:1490-503. [Crossref] [PubMed]
- Schicher N, Paulitschke V, Swoboda A, et al. Erlotinib and bevacizumab have synergistic activity against melanoma. Clin Cancer Res 2009;15:3495-502. [Crossref] [PubMed]
- Bray F, Ferlay J, Soerjomataram I, et al. Global cancer statistics 2018: GLOBOCAN estimates of incidence and mortality worldwide for 36 cancers in 185 countries. CA Cancer J Clin 2018;68:394-424. [Crossref] [PubMed]
- Zhou Y, He M, Li R, et al. The Safety and Effectiveness of Bevacizumab in the Treatment of Nonsquamous Non-Small-Cell Lung Cancer: A Meta-Analysis of Randomized Controlled Trials. Biomed Res Int 2021;2021:5537899. [Crossref] [PubMed]
- Semrad TJ, Redman MW, Herbst RS, et al. Outcomes for patients treated with or without bevacizumab on SWOG S0819: A randomized, phase III study comparing carboplatin/Paclitaxel or carboplatin/Paclitaxel/bevacizumab with or without concurrent cetuximab in patients with advanced non-small cell lung cancer (NSCLC). J Clin Oncol 2016;34:9082. [Crossref]
- Simons KH, de Vries MR, de Jong RCM, et al. IRF3 and IRF7 mediate neovascularization via inflammatory cytokines. J Cell Mol Med 2019;23:3888-96. [Crossref] [PubMed]
- Lai KC, Liu CJ, Lin TJ, et al. Blocking TNF-α inhibits angiogenesis and growth of IFIT2-depleted metastatic oral squamous cell carcinoma cells. Cancer Lett 2016;370:207-15. [Crossref] [PubMed]
- Dansin E, Cinieri S, Garrido P, et al. MO19390 (SAiL): bleeding events in a phase IV study of first-line bevacizumab with chemotherapy in patients with advanced non-squamous NSCLC. Lung Cancer 2012;76:373-9. [Crossref] [PubMed]
- Petersen I, Warth A. Lung cancer: developments, concepts, and specific aspects of the new WHO classification. J Cancer Res Clin Oncol 2016;142:895-904. [Crossref] [PubMed]
(English Language Editor: J. Gray)