Incidence rate and risk factors of ventilator-associated pneumonia in patients with traumatic brain injury: a systematic review and meta-analysis of observational studies
Highlight box
Key findings
• The risk of ventilator-associated pneumonia (VAP) in patients with brain injury is about 42%. After tracheotomy, mechanical ventilation is a risk factor of VAP, and prophylactic use of antibiotics is a protective factor of VAP.
What is known and what is new?
• The risk of VAP in patients with brain injury in the literature is inconsistent.
• In this study, a meta-analysis was performed to review the existing clinical evidence and quantitatively combine the corresponding risk estimates and the incidence rate of VAP in patients with extracerebral injuries.
What is the implication, and what should change now?
• This paper quantitatively combined the corresponding risk estimates and the incidence rate of VAP in patients with brain injury, providing guidance for clinical practice.
Introduction
Traumatic brain injury (TBI) refers to the structural or functional damage to the brain caused by external forces and is one of the main factors causing death and disability in trauma patients (1). The fatality and disability rate of TBI is about 30–40% and 60%, respectively (2,3). It is estimated that 69 million people worldwide are afflicted with TBI each year (4), and the incidence of ventilation-associated pneumonia (VAP) in patients with TBI is approximately 8.9 per 1,000 mechanical ventilation days. TBI patients usually require craniotomy for hematoma removal, and postoperative tracheal intubation and ventilator support are usually required, which will increase the incidence of VAP (5,6). Ventilator-associated pneumonia is a medically induced pneumonia that occurs within 48 h or more after receiving mechanical ventilation (7,8). The source of infection is usually bacteria colonised in the mouth or contaminated with equipment, so maintaining good oral hygiene and reducing aspiration can prevent the development of ventilator-associated pneumonia (9). In addition, studies have found that the events that trigger the development of secondary brain injury (fever, arterial hypotension, hypoxaemia, hypocapnia or hypercapnia) are also associated with early pneumonia (5,10). Therefore, identifying the risk factors for ventilator-associated pneumonia and the potential consequences of this event is also crucial in the management of head trauma.
Epidemiological evidence shows that the incidence and mortality of VAP in China are 4.7–55.8% and 19.4–51.6%, respectively. Patients with VAP usually require longer hospitalization and higher cost, which brings a larger disease burden to society and patients' lives and health (11). Therefore, Identification of risk factors associated with VAP may allow clinicians to provide more effective infection monitoring of patients and provide more timely infection control measures. However, previous observational studies have conflicting conclusions. Zhang et al. showed that prophylactic antibiotics increase the incidence of VAP in patients with TBI (12), but other studies have shown that prophylactic use of antibiotics is a protective factor for VAP (13,14). There are also inconsistencies in the research on other risk factors and the risk of VAP in patients with TBI in the literature. Therefore, this study aimed to use the methods of systematic review and meta-analysis to review the existing clinical evidence. Corresponding risk estimates and the incidence of VAP in patients with TBI were quantitatively combined, finding potential risk factors for ventilator-associated pneumonia and to provide evidence-based medical evidence for the prevention and treatment of this disease in the clinical setting. We present the following article in accordance with the MOOSE reporting checklist (available at https://jtd.amegroups.com/article/view/10.21037/jtd-23-425/rc).
Methods
Literature search
In this study, literature search was performed by using medical subject headings (MeSH) search terms in databases including PubMed, Embase, ScienceDirect, and Ovid. The search keyword (English and Chinese keywords) strategy was as follows: ("Traumatic brain injury” [MeSH Terms] OR “TBI” OR “craniocerebral trauma”) AND ("ventilator associated pneumonia” [MeSH Terms OR “VAP” OR “pneumonia”) AND (“incidence” OR “incidence rate” OR “prevalence” OR “risk factors”).
Literature screening
The inclusion criteria were the following: (I) the study design type was observational study (retrospective cohort or prospective cohort); (II) the study population was patients with VAP at or above 48 hours after TBI; (III) there was a clear definition of VAP; and (IV) the primary end point of the study consisted of at least 1 of the incidence and risk factors for VAP in patients with BTI, including gender, age, use of sedative barbiturates, use of prophylactic antibiotics, posttracheotomy, use of ventilator, or a history of diabetes.
The exclusion criteria were the following: (I) the study population was a special population, such as children or older adults or a population with a special disease group; (II) the study did not clearly provide the diagnostic criteria for VAP; (III) the experimental group in the study or the control group had a sample size of less than 20; (IV) the literature type was a review, academic conference, or case report. Finally, a total of 11 studies were included for meta-analysis.
Literature data collation and evaluation
Chen and Wu independently screened and extracted the following data from the included literature: type of study (retrospective cohort or prospective cohort), country or region of study population, year of study publication and author, sample size of VAP group and non-VAP group, incidence of VAP in patients with TBI, and risk factors including gender, age, use of sedative barbiturates, use of prophylactic antibiotics, posttracheotomy, use of ventilator, and history of diabetes. All included studies were independently assessed for literature quality by Chen and Wu using the Newcastle-Ottawa Scale (NOS) for each included study based on the following criteria (15): (I) representativeness of exposed groups, (II) selection of nonexposed groups, (III) confirmation of exposure, (IV) comparability of the 2 groups of samples, (V) offset or confounding reports, and (VI) length of follow-up. The screening of studies was mainly divided into 2 steps: (I) study title and abstract and (II) full-text screening. When Chen and Wu were in disagreement, a consensus was reached by discussing with Gao.
Statistical analysis
Stata SE 17.0 (StataCorp) was used for statistical analysis of the data in this study. The primary clinical end point observed was the relative risk (RR) for categorical variables and the mean ± standard deviation for continuous variables. The random effects model can combine the between-study variance into a total variance estimate and a confidence interval, thereby avoiding the narrowing of the confidence interval caused by the use of the fixed effects model. Therefore, in this study, both random effects and fixed effects models were used to combine risk estimates, and between-study heterogeneity was assessed using Cochran’s Q test. If there was significant heterogeneity between studies (I2>50%), the random effects model was used; otherwise, a fixed effects model was used. P<0.05 was considered statistically significant, and publication bias was described using funnel plots and assessed using Egger and Begg tests. All statistical results in this study were considered statistically significant at P≤0.05, and the hypothesis tests were 2-sided.
Results
Search results and literature features
We systematically searched medical databases including PubMed, Ovid, Embase, and ScienceDirect by using the combination of MeSHs. A total of 211 related pieces of literature were generated. After screening, a total of 11 studies were included in the meta-analysis including 2,301 patients with TBI. There were 4 prospective cohort studies and 7 retrospective cohort studies. According to the NOS (the Newcastle-Ottawa Scale, NOS), 11 studies had NOS scores between 5 and 8, of which 1 had an NOS of 8 points; the NOS score of 3 articles was 7 points, the NOS score of 5 articles was 6 points, and the NOS score of 2 articles was 5 points (see Table 1). The detailed process of literature retrieval and screening is shown in the PRISMA (Preferred Reporting Items for Systematic Reviews and Meta-Analyses) flowchart as shown in Figure 1.
Table 1
Author | Study design | Sample size (VAP cases/TBI cases) | VAP incidence rate | Age (over 60 years), RR (95% CI) | Mechanical ventilation, RR (95% CI) | Tracheostomy, RR (95% CI) | Diabetes RR (95% CI) |
Prophylactic antibiotics, RR (95% CI) | Gender (male), RR (95% CI) | Barbiturate use, RR (95% CI) | NOS |
---|---|---|---|---|---|---|---|---|---|---|---|
Zhang et al. [2019] (12) | Retrospective cohort | 27/78 | 35% | 1.163 (0.912, 1.414) |
1.883 (1.485, 2.281) |
2.135 (1.631, 2.639) |
1.006 (0.734, 1.278) |
1.206 (0.759, 1.653) |
1.618 (1.212, 2.024) |
NA | 5 |
Lin et al. [2020] (16) | Retrospective cohort | 18/67 | 27% | NA | NA | 5.983 (2.73, 8.9) |
4.986 (3.29, 7.25) |
NA | NA | NA | 6 |
Robba et al. [2020] (13) | Prospective cohort | 196/962 | 20% | 0.98 (0.97, 0.99) | NA | NA | NA | 0.69 (0.5, 0.96) | NA | 1.21 (0.9, 1.71) | 8 |
Esnault et al. [2017] (14) | Retrospective cohort | 106/243 | 44% | NA | NA | NA | NA | 0.3 (0.1, 0.8) | 1.7 (0.6, 4.4) | 2.2 (0.7, 6.8) | 5 |
Hamele et al. [2016] (17) | Retrospective cohort | 44/128 | 34% | NA | NA | NA | NA | NA | 1.1 (0.7, 1.9) | 3.2 (1.4, 7.3) | 7 |
Jovanovic et al. [2015] (18) | Prospective cohort | 71/144 | 49.30% | 1.04 (1.02, 1.07) | NA | NA | NA | NA | NA | NA | 7 |
Lepelletier et al. [2010] (19) | Retrospective cohort | 34/161 | 21% | NA | NA | NA | NA | 0.25 (0.07, 0.95) | NA | 2.68 (1.06, 6.8) | 6 |
Ewig et al. [1999] (20) | Prospective cohort | 39/48 | 81% | NA | 4.1 (0.7, 23.3) | NA | NA | 0.2 (0.05, 0.86) | NA | NA | 7 |
Zygun et al. [2006] (21) | Prospective cohort | 60/134 | 45% | NA | NA | NA | NA | NA | NA | NA | 6 |
Ding et al. [2010] (22) | Retrospective cohort | 106/192 | 55% | NA | NA | NA | NA | NA | NA | NA | 6 |
Luo et al. [2008] (23) | Retrospective cohort | 79/144 | 55% | NA | NA | NA | NA | NA | NA | NA | 6 |
TBI, traumatic brain injury; NA, not applicable; RR, relative risk; VAP, ventilator-associated pneumonia; NOS, Newcastle-Ottawa Scale.
Incidence of VAP in patients with TBI
A total of 11 studies with 2,301 patients with TBI were included in this whole study. The results of the heterogeneity test indicated that the included 11 studies had a high degree of heterogeneity (H2=26.40; I2=96.21%; P<0.001). The random effects model of the maximum likelihood method included the incidence rate. The results of meta-analysis showed that the incidence of VAP in patients after TBI was about 42% (95% CI: 32–53%), and the difference was statistically significant (P<0.001; Figure 2). The funnel plot in Figure 3 shows that the points are not completely symmetrical on both sides, and some of them are within the confidence interval, showing an inverted funnel shape and indicating a certain publication bias
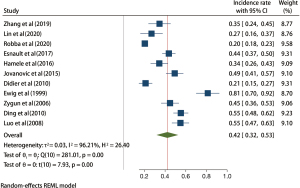
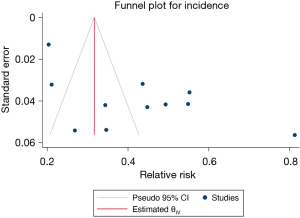
Meta-analysis of risk factors for ventilator-associated pneumonia
Age over 60 years old
A total of 3 studies with 1,578 patients with TBI were included in age analysis. The results of the heterogeneity test indicated that the included 3 studies had high heterogeneity (H2=10.67; I2=90.63%; P<0.001). A random effects model of the natural method incorporated RR. Meta-analysis results showed that age older than 60 years may increase the risk of VAP about 2% in patients with TBI (RR =1.02; 95% CI: 0.96–1.08) and the difference was statistically significant (P<0.001; Figure 4).
Posttracheotomy
A total of 277 patients with TBI from 3 studies were included in the posttracheotomy analysis, and the results of the heterogeneity test indicated that the 3 included studies had moderate heterogeneity (H2=3.03; I2=67.03%; P=0.58). The estimated random effects model incorporates RR. Meta-analysis results showed that tracheotomy significantly increased the risk of VAP in patients with TBI (RR =3.71; 95% CI: 1.48–6.94), and the difference was statistically significant (P=0.05; Figure 5).
Prophylactic antibiotic use
A total of 1,858 patients with TBI from 5 studies were included in the prophylactic antibiotic use analysis, and the results of the heterogeneity test indicated that the included 5 studies had a low degree of heterogeneity (H2=4.74; I2=78.91%; P<0.001). A random effects model of the method included RR. Meta-analysis results showed that the use of prophylactic antibiotics could significantly reduce the incidence of VAP in patients with TBI (RR =0.53; 95% CI: 0.18–0.88), and the difference was statistically significant (P<0.001; Figure 6). The funnel plot in Figure 7 shows that the points are distributed on both sides, within the confidence interval, in an inverted funnel shape, and there is no obvious publication bias, as shown in.
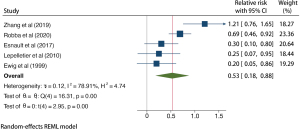
Male gender
A total of 3 studies were included in the male gender analysis, and the results of the heterogeneity test indicated that there was no heterogeneity in the 3 included studies (H2=1.01; I2=1.27%; P=0.36), and the fixed effects model was based on the inverse variance method and incorporated RR. Meta-analysis results showed that compared with female patients, male patients with TBI had a significantly higher risk of VAP by about 46% (RR =1.46; 95% CI: 1.13–1.79; P<0.001; Figure 8).
Use of barbiturates
A total of 1,874 patients with TBI from 4 studies were included in the barbiturate use analysis, and the results of the heterogeneity test indicated that the included 4 studies had a low degree of heterogeneity (H2=1.34; I2=25.27%; P=0.39). The fixed effects model based on inverse variance was used and incorporated RR. Meta-analysis results showed that the use of sedative barbiturates increased the risk of VAP in patients with TBI (RR =1.70; 95% CI: 0.64–2.77), and the difference was statistically significant (P<0.001; Figure 9). The funnel plot in Figure 10 shows that the points are distributed on both sides, within the confidence interval, and in an inverted funnel shape, indicating no obvious publication bias.
Discussion
In this study, the incidence of VAP in patients with TBI was approximately 42% (95% CI: 32–53%), slightly higher than the 10–40% reported in a previous study (24). Moreover, the incidence of VAP was even higher in the intensive care unit, reaching 60% (25). Age is a risk factor for the development of VAP, and the results of this paper show that the risk of ventilator-associated pneumonia is increased by approximately 2% when patients with TBI are older than 60 years (RR =1.02, 95% CI: 0.96, 1.08), which may be due to the fact that older patients with TBI usually require longer periods of mechanical ventilation, which in turn increases the risk of VAP (26). Tracheotomy significantly increased the risk of VAP in patients with TBI (RR =3.71; 95% CI: 1.48–6.94; P<0.05); the use of prophylactic antibiotics could significantly reduce the risk of VAP in patients with TB. The risk of pneumonia (RR =0.53; 95% CI: 0.18–0.88; P<0.05), which is consistent with the results of previous studies. Some studies showed prophylactic antibiotic use reduces risk of VAP by 30% (27,28). However, although the clinical use of prophylactic antibiotics has been recommended by many investigators, it is still not the standard clinical approach due to concerns about bacterial resistance, and the benefits of prophylactic antibiotics do not necessarily outweigh the risks. Compared with female patients, male patients with TBI had a significantly higher risk of VAP by about 46% (RR =1.46; 95% CI: 1.13–1.79; P<0.05). Several studies have reported a relatively low risk of post-traumatic infection and death in female patients (29), and the same consistent conclusion has been reached in female patients with TBI (12,14,17). The female patients with TBI survival benefit has only been found in the older population (>60 years), which may be due to the aging process male patients have more rapid immune senescence (29), thus it is important to beware of VAP in older patients with TBI in clinical practice, especially in men.
In addition, Zhang et al. (12) showed that prolonged mechanical ventilation (>5 days) significantly increased the risk of ventilator-associated pneumonia. Hamele and Lepelletier et al. showed that the use of the sedative barbiturate increased the risk of ventilator-associated pneumonia in patients with TBI (17,19), possibly because the sedative drug caused the oesophageal sphincter in patients with TBI to become This may be because sedative drugs cause the oesophageal sphincter to become relaxed in patients with TBI, which in turn increases the risk of gastric reflux and increases the risk of VAP. Therefore, it has been suggested that during the clinical care of patients with TBI, especially after tracheal intubation, who require prolonged mechanical ventilation, the residual volume of gastric contents should be monitored dynamically to prevent gastric retention and, if necessary, to enhance peristalsis with gastrointestinal stimulants in order to reduce the risk of aspiration leading to VAP (30,31). On the other hand, it has been shown that the positive air pressure of mechanical ventilation may damage the respiratory mucosa, thereby reducing the differences of the airways, so it is important to continuously monitor the artificial airway balloon pressure during post-tracheal intubation and mechanical ventilation in patients with TBI in order to prevent the development of VAP. In addition, it has been shown that H2 receptor antagonists reduce gastric acid secretion and that a less acidic environment promotes bacterial colonization of the stomach, thereby increasing the risk of ventilator-associated pneumonia in hospitalized patients (32-34). The incidence of ventilator-associated pneumonia is also correlated with the degree of trauma Torres et al. found that when TBI patients are combined with thoracic trauma, the severity of trauma is higher, airway bleeding is more severe, ventilator management is more difficult, the duration of use is increased, and the incidence of VAP increases (35).
However, this study had some limitations that should be noted. First, although the diagnosis of most studies was based on laboratory tests, clinical features, and imaging studies, the criteria for the clinical diagnosis of VAP in the included studies were not the same. The included studies mainly used the following 3 types of diagnostic criteria: Centers for Disease Control and Prevention (CDC) criteria, American Thoracic Society (ATS) criteria, and Chinese Respiratory Society criteria. Therefore, future research needs to unify the diagnostic criteria for VAP to homogenize the observed population. Second, this paper extracted data on gender, age, use of sedative barbiturates, use of prophylactic antibiotics, posttracheotomy, use of ventilators, history of diabetes, etc. During the data collection phase, however, some risk factors reported fewer than 3 articles, and we were unable to combine the effect sizes of all risk factors, but we qualitatively described these risk factors in the search results section. Third, the models with some effect sizes combined in this study had high heterogeneity, and due to the small number of studies included in the combined model of each risk factor, we could not conduct subgroup analysis, which led to the inability to identify the source of model heterogeneity. Therefore, future studies should include more literature to enable subgroup analysis or meta-regression to explore heterogeneity and obtain a higher level of clinical evidence.
Conclusions
The risk of VAP in patients with TBI is about 42%. Posttracheotomy and mechanical ventilation are risk factors for VAP, while prophylactic antibiotics are protective against VAP. However, considering that the studies included in this article are not randomized controlled studies and there is heterogeneity, more high-quality studies need to be included for further validation in the future.
Acknowledgments
Funding: None.
Footnote
Reporting Checklist: The authors have completed the MOOSE reporting checklist. Available at https://jtd.amegroups.com/article/view/10.21037/jtd-23-425/rc
Peer Review File: Available at https://jtd.amegroups.com/article/view/10.21037/jtd-23-425/prf
Conflicts of Interest: All authors have completed the ICMJE uniform disclosure form (available at https://jtd.amegroups.com/article/view/10.21037/jtd-23-425/coif). The authors have no conflicts of interest to declare.
Ethical Statement: The authors are accountable for all aspects of the work in ensuring that questions related to the accuracy or integrity of any part of the work are appropriately investigated and resolved.
Open Access Statement: This is an Open Access article distributed in accordance with the Creative Commons Attribution-NonCommercial-NoDerivs 4.0 International License (CC BY-NC-ND 4.0), which permits the non-commercial replication and distribution of the article with the strict proviso that no changes or edits are made and the original work is properly cited (including links to both the formal publication through the relevant DOI and the license). See: https://creativecommons.org/licenses/by-nc-nd/4.0/.
References
- Brett BL, Gardner RC, Godbout J, et al. Traumatic Brain Injury and Risk of Neurodegenerative Disorder. Biol Psychiatry 2022;91:498-507. [Crossref] [PubMed]
- Mena JH, Sanchez AI, Rubiano AM, et al. Effect of the modified Glasgow Coma Scale score criteria for mild traumatic brain injury on mortality prediction: comparing classic and modified Glasgow Coma Scale score model scores of 13. J Trauma 2011;71:1185-92; discussion 1193. [Crossref] [PubMed]
- Dikmen SS, Machamer JE, Powell JM, et al. Outcome 3 to 5 years after moderate to severe traumatic brain injury. Arch Phys Med Rehabil 2003;84:1449-57. [Crossref] [PubMed]
- Dewan MC, Rattani A, Gupta S, et al. Estimating the global incidence of traumatic brain injury. J Neurosurg 2018;1-18. Epub ahead of print. [Crossref] [PubMed]
- Gahagen RE, Beardsley AL, Maue DK, et al. Early-Onset Ventilator-Associated Pneumonia in Pediatric Severe Traumatic Brain Injury. Neurocrit Care 2023; Epub ahead of print. [Crossref] [PubMed]
- Wu D, Wu C, Zhang S, et al. Risk Factors of Ventilator-Associated Pneumonia in Critically III Patients. Front Pharmacol 2019;10:482. [Crossref] [PubMed]
- Hunter JD. Ventilator associated pneumonia. BMJ 2012;344:e3325. [Crossref] [PubMed]
- Oliveira J, Zagalo C, Cavaco-Silva P. Prevention of ventilator-associated pneumonia. Rev Port Pneumol 2014;20:152-61. [Crossref] [PubMed]
- Ludovichetti FS, Zuccon A, Positello P, et al. Preventive oral hygiene and ventilator-associated pneumonia in paediatric intensive care unit. Eur J Paediatr Dent 2022;23:298-302. [PubMed]
- Inoue A, Hifumi T, Sakamoto T, et al. Extracorporeal Cardiopulmonary Resuscitation for Out-of-Hospital Cardiac Arrest in Adult Patients. J Am Heart Assoc 2020;9:e015291. [Crossref] [PubMed]
- Cheng S. Epidemiological characteristics of ventilator-associated pneumonia in ICUs in my country are elucidated. China Medical Information Herald 2019;34:8.
- Zhang Q, Yang Y, Qian W, et al. Pathogen distribution and influencing factors of ventilator-associated pneumonia in patients with traumatic brain injury. Chinese Journal of Disinfection 2019;36:477-79.
- Robba C, Rebora P, Banzato E, et al. Incidence, Risk Factors, and Effects on Outcome of Ventilator-Associated Pneumonia in Patients With Traumatic Brain Injury: Analysis of a Large, Multicenter, Prospective, Observational Longitudinal Study. Chest 2020;158:2292-303. [Crossref] [PubMed]
- Esnault P, Nguyen C, Bordes J, et al. Early-Onset Ventilator-Associated Pneumonia in Patients with Severe Traumatic Brain Injury: Incidence, Risk Factors, and Consequences in Cerebral Oxygenation and Outcome. Neurocrit Care 2017;27:187-98. [Crossref] [PubMed]
- Higgins JP, Altman DG, Gøtzsche PC, et al. The Cochrane Collaboration's tool for assessing risk of bias in randomised trials. Bmj 2011;343:d5928. [Crossref] [PubMed]
- Lin XF, Zheng X, Sun H. Factors affecting ventilator-associated pneumonia after severe traumatic brain injury. China Health Standard Management 2020;11:40-42.
- Hamele M, Stockmann C, Cirulis M, et al. Ventilator-Associated Pneumonia in Pediatric Traumatic Brain Injury. J Neurotrauma 2016;33:832-9. [Crossref] [PubMed]
- Jovanovic B, Milan Z, Markovic-Denic L, et al. Risk factors for ventilator-associated pneumonia in patients with severe traumatic brain injury in a Serbian trauma centre. Int J Infect Dis 2015;38:46-51. [Crossref] [PubMed]
- Lepelletier D, Roquilly A. Retrospective analysis of the risk factors and pathogens associated with early-onset ventilator-associated pneumonia in surgical-ICU head-trauma patients. J Neurosurg Anesthesiol 2010;22:32-7. [Crossref] [PubMed]
- Ewig S, Torres A, El-Ebiary M, et al. Bacterial colonization patterns in mechanically ventilated patients with traumatic and medical head injury. Incidence, risk factors, and association with ventilator-associated pneumonia. Am J Respir Crit Care Med 1999;159:188-98. [Crossref] [PubMed]
- Zygun DA, Zuege DJ, Boiteau PJ, et al. Ventilator-associated pneumonia in severe traumatic brain injury. Neurocrit Care 2006;5:108-14. [Crossref] [PubMed]
- Ding X, Hu S, Zeng M. Clinical analysis of secondary ventilator-associated pneumonia in patients with severe craniocerebral injury. Journal of Modern Integrative Medicine 2010;3166-8.
- Luo R, Zan X. Analysis and countermeasures of related factors of ventilator-associated pneumonia in patients with craniocerebral trauma. Journal of Clinical and Experimental Medicine 2008;7:45.
- Reignier J, Mercier E, Le Gouge A, et al. Effect of not monitoring residual gastric volume on risk of ventilator-associated pneumonia in adults receiving mechanical ventilation and early enteral feeding: a randomized controlled trial. JAMA 2013;309:249-56. [Crossref] [PubMed]
- Colombo SM, Palomeque AC, Li Bassi G. The zero-VAP sophistry and controversies surrounding prevention of ventilator-associated pneumonia. Intensive Care Med 2020;46:368-71. [Crossref] [PubMed]
- Al Bshabshe A, Joseph MRP, Al Hussein A, et al. Multidrug resistance Acinetobacter species at the intensive care unit, Aseer Central Hospital, Saudi Arabia: A one year analysis. Asian Pac J Trop Med 2016;9:903-8. [Crossref] [PubMed]
- Sirvent JM, Torres A, El-Ebiary M, et al. Protective effect of intravenously administered cefuroxime against nosocomial pneumonia in patients with structural coma. Am J Respir Crit Care Med 1997;155:1729-34. [Crossref] [PubMed]
- Acquarolo A, Urli T, Perone G, et al. Antibiotic prophylaxis of early onset pneumonia in critically ill comatose patients. A randomized study. Intensive Care Med 2005;31:510-6. [Crossref] [PubMed]
- Umemura Y, Katayama Y, Kitamura T, et al. Patient age affects sex-based differences in post-traumatic mortality: a national trauma registry study in Japan. Eur J Trauma Emerg Surg 2022;48:2731-40. [Crossref] [PubMed]
- Wang H. The preventive effect of comprehensive predictive nursing intervention combined with high frequency shock expectoration on ventilator-associated pneumonia in mechanically ventilated patients. Journal of Practical Medical Technology 2021;9:1153-4.
- Zhou X, Hu X. Effective nursing outcomes for patients with artificial airway established in the ICU intensive care unit. International Nursing and Health 2022;3.
- Hammond DA, Kathe N, Shah A, Martin BC. Cost-Effectiveness of Histamine2 Receptor Antagonists Versus Proton Pump Inhibitors for Stress Ulcer Prophylaxis in Critically Ill Patients. Pharmacotherapy 2017;37:43-53. [Crossref] [PubMed]
- Grindlinger GA, Cairo SB, Duperre CB. Pneumonia prevention in intubated patients given sucralfate versus proton-pump inhibitors and/or histamine II receptor blockers. J Surg Res 2016;206:398-404. [Crossref] [PubMed]
- Driks MR, Craven DE, Celli BR, et al. Nosocomial pneumonia in intubated patients given sucralfate as compared with antacids or histamine type 2 blockers. The role of gastric colonization. N Engl J Med 1987;317:1376-82. [Crossref] [PubMed]
- Torres A, Carlet J. Ventilator-associated pneumonia. Eur Respir J 2001;17:1034-45. [Crossref] [PubMed]
(English Language Editor: J. Gray)