Long non-coding RNA DINO promotes cisplatin sensitivity in lung adenocarcinoma via the p53-Bax axis
Highlight box
Key findings
• The lncRNA DINO stabilizes p53 and activates the p53-Bax axis, thereby regulating the sensitivity of lung adenocarcinoma to cisplatin.
What is known and what is new?
• (I) DNA damage induces the expression of the lncRNA DINO; (II) the lncRNA DINO stabilizes wild-type p53;
• (I) Cisplatin treatment of lung cancer stimulates lncRNA DINO expression; (II) the lncRNA DINO activates the p53-Bax axis to enhance cisplatin sensitivity.
What is the implication, and what should change now?
• The lncRNA DINO may be a novel therapeutic target to overcome cisplatin resistance.
Introduction
According to the 2023 cancer statistics, lung cancer is the leading cause of cancer-related death, with 350 people dying of lung cancer every day (1). Approximately 85% of lung cancer cases are non-small cell lung cancer (NSCLC), and lung adenocarcinoma (LUAD) is the most common subtype of NSCLC (2). Patients with NSCLC can be treated with chemotherapy, surgery, radiation, targeted therapy, or immunotherapy to improve survival (3). However, due to the lack of typical clinical symptoms for early-stage lung cancer, 65–80% of patients are diagnosed with middle- or advanced-stage disease (4). Chemotherapy is still the primary treatment option for patients without a driver gene mutation or with recurrent lung cancer (5). Platinum compounds have a broad antitumor spectrum, and their mechanism involves forming a complex with DNA via cross-linking to hinder the processes of cell division and replication, thereby exerting an anticancer effect (6,7). Despite the emergence of new-generation platinum drugs, the side effects of high-dose cisplatin (DDP) therapy and drug resistance still limit efficacy (8,9). Therefore, there is an urgent need to develop novel strategies to enhance the therapeutic effect of platinum drugs.
Long non-coding RNA (lncRNA) is a type of long (>200 nucleotides) non-coding RNA molecule that does not have the typical function of encoding a protein (10). Recent research has shown that lncRNAs are involved in the occurrence of cancer pathological conditions, a component of the tumor microenvironment, and the modification of chemoresistance (11-15). In November 2016, Schmitt et al. discovered that human cells transcribed a new lncRNA after being treated with doxorubicin and named lncRNA DINO (damage-induced non-coding) (16). Upregulation of the lncRNA DINO stabilizes the p53 protein and activates the DNA damage repair pathway (17). The treatment of tumors with DDP can also cause DNA damage; however, whether the lncRNA DINO is involved in the treatment of LUAD remains unclear.
The tumor suppressor p53 is critically involved in DDP-induced apoptosis (18-20). After DNA damage, DNA repair requires p53 to activate p21, which blocks the G2/M phase (21). However, if the DNA damage is too severe to repair, the upregulation of Bax by p53 promotes the apoptotic process via the mitochondrial pathway (22,23). Therefore, when p53 is involved in the treatment of NSCLC with DDP, it is necessary to pay attention to the downstream targets of p53 to find suitable therapeutic targets.
In this study, we hypothesized that the lncRNA DINO affects DDP resistance via the p53-Bax axis through p53 stability. To verify this hypothesis, we detected the expression level of the lncRNA DINO in NSCLC cell lines. Through in vitro and in vivo experimental assays, we further investigated the functional relevance of the lncRNA DINO in NSCLC chemoresistance. We present the following article in accordance with the ARRIVE reporting checklist (available at https://jtd.amegroups.com/article/view/10.21037/jtd-23-465/rc).
Methods
Materials and reagents
Phosphate-buffered saline (PBS), RPMI-1640 medium, and fetal bovine serum (FBS) were procured from Gibco (Grand Island, USA). Cell Counting Kit-8 (CCK-8) and cycloheximide (CHX) were obtained from GLPBIO (California, USA). PEN-STREP solution (10,000 units/mL penicillin and 10 mg/mL streptomycin) was produced by Biological Industries (Beit Haemek, Israel). An Annexin V-fluorescein isothiocyanate (FITC)/propidium iodide (PI) cell apoptosis detection kit was provided by KeyGEN Biotech (Nanjing, China). DDP (#D8810) was purchased from Solarbio (Beijing, China). Antibodies against the following proteins were used: p53 (Proteintech, China), p21 (Proteintech, China), Bax (CST, USA), poly ADP-ribose polymerase (PARP) (Wanleibio, China), and glyceraldehyde 3-phosphate dehydrogenase (GAPDH) (Bioworld Technology, China).
Cells and cell culture
The human lung carcinoma cell lines, H460, A549, H1299, H1993, Hcc827, PC9, and H1975, and the normal lung epithelial cell line HBE were obtained from American Type Culture Collection (ATCC, VA, USA). The DDP-resistant cell line, A549R, was established from A549 cells in our laboratory by culturing A549 cells with increasing concentrations of DDP for 6 months; A549R cells were cultured in a medium containing DDP at a concentration of 1 µg/mL to maintain resistance. All the human lung carcinoma cell lines were cultured in RPMI-1640 medium containing 10% heat‐inactivated FBS and 1% PEN-STREP solution in an environment of 5% CO2 at 37 ℃.
Vector construction and transduction
Cell transfection was performed using Lipofectamine 3000 Reagent (Invitrogen, USA) according to the manufacturer’s protocol. DINO small interfering (si) RNAs (si-DINO-1 and si-DINO-2) or a p53 interference plasmid were synthesized by Suzhou GenePharma Co., Ltd. (China). A lentiCRISPRv2 interference plasmid for the Bax gene was obtained from Guangzhou IGE Biotechnology Co., Ltd. (China). A specific interference sequence (si-DINO-2) was cloned into the pLKO.1-puro plasmid and cotransfected with the pVSV-G, pMDLg, and pRSV-Rev plasmids into HEK293T cells to generate viruses. A lentivirus (LV) encoding the lncRNA DINO gene was purchased from Shanghai Genechem Co., Ltd. (China). Information on the interference sequences is shown in Table S1.
RNA isolation and quantitative real-time polymerase chain reaction (qRT-PCR)
All steps were performed in accordance with the manufacturer’s instructions. Total RNA was extracted using TRIzol (Takara, Japan), and the RNA concentration was detected with a NanoDrop2000 spectrophotometer (Thermo Fisher, USA).
Complementary DNA (cDNA)was synthesized by reverse transcription of RNA by using a PrimeScript RT reagent kit (Takara). qRT-PCR was performed using SYBR premix (Takara) and a CFX96 PCR detection system (Bio-Rad, USA). GAPDH was used as a standardization control, and the results were calculated by the 2−ΔΔCt method. The primer sequence information is shown in Table S2.
CCK-8 assay
A total of 10,000 cells per well were cultured in 96-well plates and incubated at 37 ℃ overnight. The next day, the cells were treated with various concentrations of DDP for 48 h and then incubated with 10 µL of CCK-8 solution added to each well (100 µL of medium). After incubation at 37 ℃ for 1 h, the absorbance at 450 nm optical density (OD) value was detected using a microplate reader (Biotex, USA). Moreover, the half-maximal inhibitory concentration (IC50) values were then calculated from the OD value using SPSS software (Chicago, IL, USA). Each experiment was performed in triplicate.
Cell apoptosis analysis
The analysis of NSCLC cell apoptosis was performed using an Annexin V-FITC/PI cell apoptosis detection kit. Briefly, all cells were harvested using trypsin without ethylene diamine tetraacetic acid (EDTA) and washed twice with ice-cold PBS. Next, the cells were resuspended in 1× binding buffer at 1×106 cells/mL. Five microliters of PI and 5 µL of Annexin V-FITC were added, and the suspension was incubated for 15 min at room temperature protected from light and then analyzed using a CytoFLEX cytometer (Beckman-Coulter, USA). Finally, the analysis results were analyzed using FlowJo software (version 10.6.0, LLC, Oregon, USA).
Western blotting
After processing the cells according to the experimental requirements, the samples were washed twice with ice-cold PBS and lysed with Radio Immunoprecipitation Assay (RIPA) buffer. Next, after centrifugation at 12,000 rpm for 15 min, the samples were boiled in a sodium dodecyl sulfate (SDS) loading buffer and separated on 10% SDS-polyacrylamide gel electrophoresis (SDS-PAGE). The proteins were transferred to polyvinylidene fluoride (PVDF) membranes, which were blocked with 5% bovine serum albumin (BSA) for 2 h at 30 ℃, and then incubated with primary antibodies at 4 ℃ overnight. Next, the membranes were incubated for 2 h at 30 ℃ with horseradish peroxidase (HRP)‐conjugated secondary antibodies. Using enhanced chemiluminescence (ECL) detection reagents (Millipore, USA), the membranes were visualized with a ChemiDoc XRS+ system (Bio-Rad, USA).
CHX chase assay
Stable cell lines were constructed with a lentiviral system, and then the medium was supplemented with 25 µg/mL CHX (GLPBIO). After processing cells at the indicated times according to experimental need, the cells were washed with ice-cold PBS and collected in Eppendorf (EP) tubes, followed by the addition of lysis buffer containing protease inhibitors. Western blotting was performed as described previously to detect the levels of the p53 protein.
Tumor xenograft experiments
According to the institutional guidelines of the Guangdong Province, 4- to 6-week-old female nude BALB/c mice were purchased from the Experimental Animal Center of Southern Medical University (Guangzhou, China). The nude mice were reared at 25 ℃ under a 12-h light/dark cycle in cages with sufficient space for exploration and access to fresh food and water at all times. According to the protocols outlined in the literature (24,25), a total of 10 nude mice were injected in the right flank with 200 µL of PBS containing 1×106DINO overexpression LV vector-transfected A549R cells for xenograft tumor formation. Additionally, another 10 nude mice were injected with the same number of negative control (NC) LV vector-transfected A549R cells.
All mice were observed for 7 days; when the tumor volume reached 100 mm3, the 10 tumor-bearing nude mice overexpressing DINO were randomly divided into two groups (n=5 per group). The first group was injected intraperitoneally with DDP (2 mg/kg) every other day, while the second group was injected intraperitoneally with the same amount of PBS every other day. Similarly, 10 tumor-bearing NC gene nude mice were also randomly divided into two groups (n=5 per group). The first group was injected intraperitoneally with DDP (2 mg/kg) every other day, while the second group was injected intraperitoneally with the same amount of PBS every other day. During the treatment, the long and short diameters of the tumors were recorded every 3 days. When the length and diameter of the tumors in the nude mice reached approximately 1 cm, all of the mice were euthanized by CO2 suffocation, after which the tumor was removed, weighed, and photographed. The study was started in Southern Medical University. Animal experiments were performed under a project license (No. 2018A0303130233) granted by institutional ethics board of Southern Medical University, in compliance with institutional guidelines for the care and use of animals. A protocol was prepared before the study without registration.
Immunohistochemistry (IHC) and hematoxylin and eosin (H&E) staining
IHC staining was performed as follows: first, subcutaneous tumors from the nude mice were fixed in formalin, embedded in paraffin, and cut into 5-µm-thick sections. After deparaffinization and rehydration with xylene and ethanol, the sections were boiled with citrate for antigen retrieval and treated with hydrogen peroxide (3%) to abrogate the endogenous peroxidase activity. Next, the non-specific staining was blocked with BSA, and the sections were incubated with a primary antibody overnight at 4 ℃. Subsequently, secondary antibodies (Dako, Denmark) coupled to HRP were applied, and detection was performed with 3,3-diaminobenzidine (DAB) incubation, followed by hematoxylin counterstaining.
H&E staining was conducted as follows: after dewaxing and rehydration, the sections were stained with H&E. Finally, the sections were washed with pure water and observed under a microscope (Leica, Heidelberg, Germany).
Statistical analysis
Data are expressed as the mean ± standard deviation (SD) of at least three independent experiments. Statistical analysis was performed using the t-test or one-way analysis of variance (ANOVA). All comparisons for which the P value was <0.05 were considered statistically significant.
Results
LncRNA DINO was significantly down-regulated in NSCLC and increased by DDP
To investigate the potential role of the lncRNA DINO in NSCLC, we first used real-time PCR to explore the expression of the lncRNA DINO. The results showed that the gene expression levels of DINO were significantly down-regulated in the H460, A549, H1299, H1993, Hcc827, PC9, and H1975 cell lines compared with the normal lung epithelial cell line HBE (Figure 1A). To determine the role of the lncRNA DINO in mediating resistance to chemotherapy, the NSCLC cell line, A549, and DDP-resistant cell line, A549R, were used to investigate the effect of DDP treatment. In the A549 cell line, we observed that the lncRNA DINO expression level was increased by DDP in a dose-dependent manner (Figure 1B). The CCK-8 assay showed that the IC50 of A549R cells was 3.412 µg/mL, which was ~3-fold higher than that of A549 cells (0.947 µg/mL) (Figure 1C). The lncRNA DINO was also highly expressed in the DDP-resistant cell line A549R, which was cultured in a medium containing DDP at a concentration of 1 µg/mL to maintain resistance. However, there were no significant differences between A549 and A549R cells cultured without DDP for 2 weeks (Figure 1D). These results suggested that the expression of the lncRNA DINO increased with DDP treatment in a dose-dependent manner in A549 and A549R cells; therefore, the lncRNA DINO might be involved in the DDP treatment of NSCLC cells.
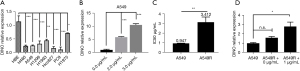
LncRNA DINO enhanced the sensitivity of NSCLC cells to DDP
To determine the role of the lncRNA DINO in mediating sensitivity to chemotherapy, A549 and A549R cells were transfected with a control LV or DINO overexpression LV to upregulate the expression of the lncRNA DINO (Figure 2A). The CCK-8 assay showed that lncRNA DINO overexpression decreased the IC50 values of DDP in the A549 and A549R cell lines (Figure 2B,2C). Next, flow cytometry was performed to observe apoptosis; as shown in Figure 2D-2F), the cell apoptosis of A549 and A549R cells was markedly activated by overexpressing the lncRNA DINO.

Down-regulation of the lncRNA DINO decreased the sensitivity of NSCLC cells to DDP
The effect of lncRNA DINO down-regulation on DDP sensitivity was also investigated via the transfection of si-DINO-1 and si-DINO-2 on NSCLC cells. qRT-PCR confirmed that si-DINO significantly decreased the expression of the lncRNA DINO in the two cell lines after DDP treatment (Figure 3A). As shown in Figure 3B-3D, NSCLC cell apoptosis was found to be significantly suppressed after decreasing DINO expression by flow cytometry. The CCK-8 assay also showed that si-DINO increased the IC50 values of DDP in A549 and A549R cells (Figure 3E-3H).
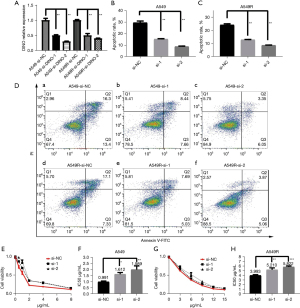
LncRNA DINO targeted p53 in NSCLC regulation
The knockdown achieved with siRNA-2 was better than that achieved with siRNA-1. Therefore, siRNA-2 was packaged into LVs to inhibit lncRNA DINO expression in NSCLC cells. Next, we investigated whether the lncRNA DINO regulates p53 expression levels. By using qRT-PCR and Western blot assays, we found that the lncRNA DINO did not affect the transcription level of p53 (Figure 4A,4B). However, in the Western blot assay, lncRNA DINO overexpression elevated the expression levels of p53, which were decreased by the down-regulation of lncRNA DINO (Figure 4C,4D). CHX was used to block the synthesis of new p53 protein. The results showed that after treatment with CHX for different durations, the expression of the p53 protein in the LV-DINO group was higher than that in the NC group. At the same time, the expression of p53 in the small hairpin (sh)-DINO group was lower (Figure 4E,4F).
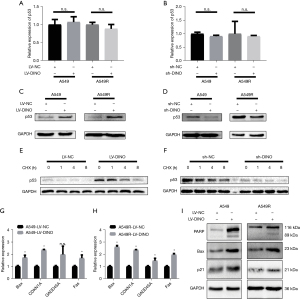
The p53-Bax axis was involved in chemotherapy sensitivity induced by lncRNA DINO overexpression
Since the lncRNA DINO augments p53 stability, we speculated that the lncRNA DINO activates the p53 pathway. qRT-PCR showed that the p53 downstream genes, Bax, CDKN1A, and Fas, were upregulated in two cell lines with stable overexpression of the lncRNA DINO (Figure 4G,4H). In the Western blot assay, the expressions of the proapoptotic proteins, Bax and cleaved PARP, were increased in the LV-DINO group (Figure 4I). These results suggested that the lncRNA DINO stabilizes p53 and regulates the sensitivity of NSCLC cells to DDP, which may involve the p53-Bax axis.
Overexpression of DINO reversed the chemotherapy resistance induced by silencing the p53-Bax axis
We further examined whether the lncRNA DINO indeed exerts its function through the p53-Bax axis. First, an interference plasmid (sh-p53) targeting the p53 mRNA transcript was synthesized and transfected into A549 cells. We co-transfected A549 cells with a lncRNA DINO overexpression LV and an interference plasmid (sh-p53), and the cells were divided into the following groups: DINO(−) + sh-p53(−), DINO(−) + sh-p53(+), DINO(+) + sh-p53(−), and DINO(+) + sh-p53(+). The Western blot results showed that the apoptosis-related proteins, p53, Bax, PARP, and cleaved PARP, were decreased in the group with DINO(−) + sh-p53(+). However, when DINO was present in the group with DINO(+) + sh-p53(+), it partially reversed the apoptosis-related proteins levels (Figure 5A). The IC50 was slightly increased in the group with DINO(−) + sh-p53(+) but partially reversed in the group with DINO(+) + sh-p53(+) (Figure 5B). A similar experiment yielded comparable results, as flow cytometry showed moderate enhancement of cell apoptosis in the LV-DINO + sh-p53 group compared to the LV-NC + sh-p53 group (Figure 5C,5D).
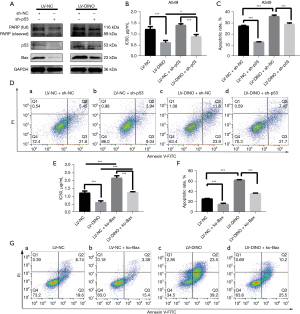
The Bax gene was regulated by the tumor suppressor p53 and was an important component in cell apoptosis
We generated Bax knockout (ko-Bax) cells using the CRISPR/Cas9 system. The CCK-8 assays showed that the IC50 was significantly reduced after the cotransfection of LV-DINO and ko-Bax vectors (as compared to the LV-NC + ko-Bax group) (Figure 5E). Also, flow cytometry demonstrated a significant reduction in apoptosis in the LV-NC + ko-Bax group. However, in the group with cotransfection of LV-DINO + ko-Bax, this effect on apoptosis was reversed (Figure 5F,5G). These data suggest that the lncRNA DINO regulates chemotherapy-induced apoptosis via the p53-Bax axis.
LncRNA DINO enhanced DDP sensitivity in vivo
To further assess the effect of the lncRNA DINO on the sensitivity of NSCLC cells to DDP in vivo, A549R-LV-NC or A549R-LV-DINO cells were inoculated into the right flank of immunocompromised nude mice. After 7 days of tumor formation, the nude mice were intraperitoneally injected with DDP (2 mg/kg) or PBS, and the tumor volume was subsequently measured. After 21 days of recording, the A549R-LV-DINO-inoculated mice showed a significantly decreased tumor volume and weight compared with the A549R-LV-NC mice (Figure 6A-6C). H&E staining showed that the group with lncRNA DINO up-regulation had a higher degree of necrosis, while the LV-NC group exhibited almost no observable necrosis. IHC analysis showed that the lncRNA DINO up-regulation significantly increased the apoptosis-related protein, Bax, and decreased the anti-apoptotic protein, Bcl-2 (Figure 6D). Together, these data suggested that lncRNA DINO overexpression sensitized NSCLC cells to DDP in vivo.
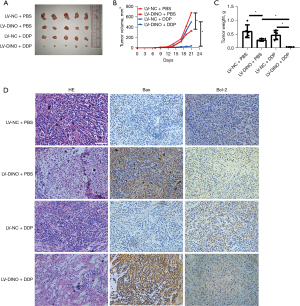
Discussion
At present, the main pathological type of lung cancer is NSCLC, which has the highest mortality rate among all malignant tumors (1); 57% of lung cancer patients have metastatic disease at the time of diagnosis, and their 5-year relative survival rate is less than 20% (26). Currently targeted therapy is recommended as first line treatment for patient with sensitive mutation. But all patients with mutation will be resistant to targeted therapy eventually. Then chemotherapy will be the treatment choice. Thus, the issue of DDP resistance will be problem for all patients, with or without sensitive mutation (27,28). Herein, we reported that the lncRNA DINO reduced DDP resistance via the p53-Bax axis through p53 stability.
We first analyzed the relative expression of the lncRNA DINO in eight cell lines and found that compared with normal lung epithelial HBE cells, the lung cancer cell lines showed significant down-regulation of the lncRNA DINO. A growing number of studies have noted that lncRNAs can be biomarkers; for example, prostate cancer associated 3 (PCA3) is the first biomarker approved by the U.S. Food and Drug Administration to predict prostate tumorigenesis (13). Follow-up reports have also found that a higher PCA3 score (>60) is significantly related to identifying cancer from an initial prostate biopsy (29). Furthermore, AA174084 is expressed at low levels in gastric cancer, and the area under the receiver operating characteristic (ROC) curve is 0.848, suggesting that AA174084 may be a biomarker for predicting gastric cancer (30). Increasing theoretical and experimental studies have shown that the down-regulation of the lncRNA DINO in lung mass biopsies may be a potential biomarker to assist pathologists in the diagnosis of early-stage lung cancer. In the future, biopsy specimens will be collected to test the relationship between lncRNA DINO and the clinical stage of lung cancer.
The lncRNA DINO is located at chr6:36,675,485–36,678,559 (GRCh38/hg38) and consists of 951 nucleotides in the upstream reverse transcription region of the CDKN1A gene. In the initial study, as human cells were damaged by doxorubicin, the expression of lncRNA DINO was upregulated, which promoted DNA damage repair pathways or cell apoptosis by binding to and stabilizing the p53 protein (16). Subsequent research reports have pointed out that in acute liver injury, the lncRNA DINO is regulated by nuclear receptor subfamily 2 group E member 3 (NR2E3) to activate the p53 pathway to reduce severe liver injuries (31). In cervical cancer, inducing lncRNA DINO expression can also increase the stability of the p53 protein, thereby increasing the sensitization to chemotherapeutic agents and making cells more easily affected by metabolic pressure (32). Moreover, human papillomavirus 16 (HPV16) E7 can increase the expression level of p53, with the lncRNA DINO acting as the middle link between them (33). In HTR-8/SVneo human chorionic trophoblast cells, DINO has been shown to down-regulate the expression of matrix metallopeptidase 2 (MMP2) and inhibit the invasion and migration of these cells (34). In addition, DINO deficiency in mice has been linked to spontaneous tumorigenesis, with the tumor type being similar to that of human cancer, suggesting that DINO is an effective tumor suppressor molecule (35).
To identify the potential role of lncRNAs in DNA damage induced by DDP treatment, we investigated the functional relevance of the lncRNA DINO expression to DDP sensitivity in NSCLC. In the context of upregulated lncRNA DINO expression, increased apoptosis was observed during DDP treatment, and the p53-Bax axis was activated by the increase in p53 stability. However, in the absence of lncRNA DINO expression, apoptosis was greatly reduced, and the p53-bax axis hardly responded. To further verify that the lncRNA DINO affects the DDP sensitivity of cells through the p53-Bax axis, a p53 interference plasmid was transfected; this treatment completely silenced the downstream genes of the p53-Bax axis and markedly reduced apoptosis. Consistent with these findings, in the virus group overexpressing the lncRNA DINO, the expression of the downstream genes of the p53-Bax axis was restored, and the drug resistance of the treated cells was reversed. Under normal circumstances, Bax needs to form a homologous complex to activate the mitochondria-dependent apoptosis program (36,37). However, recent reports have pointed out that p53 can directly activate Bax in the cytoplasm by binding to structural regions, thereby triggering the corresponding cell death program (38). To explore the relationship between the lncRNA DINO and p53-Bax axis, a CRISPR-CAS9 system was designed to knock out the Bax gene as much as possible. Similarly, the CRISPR-CAS9 system that knocked out the Bax gene partially reversed DDP resistance after lentiviral overexpression of DINO.
Cell models are easily affected by the external environment in the culture medium, while the body is complex and relatively stable. For this reason, nude mice were used as a model for in vivo experiments to investigate the role of lncRNA DINO in the body. After subcutaneous tumor formation in the nude mice, the tumor volume in the lncRNA DINO overexpression group was relatively low. Evaluation of the dissected tumors also showed that the proapoptotic Bax protein was increased in the tumors of the lncRNA DINO overexpression group and that the antiapoptotic protein Bcl-2 was decreased, and H&E staining showed increased necrosis. Before clinical application, safety and efficacy are the most important issues to be overcome with lncRNA, but by combining with nanomaterials, some lncRNA gene therapies have been applied in animal experiments and are expected to undergo subsequent clinical trials. For example, folate (FA)-modified liposomal (FA-LP) nanoparticles were loaded with the lncRNA metallothionein 1D, pseudogene (MT1DP) to regulate a protein kinase in NSCLC, which caused increased iron apoptosis (39). The lncRNA growth arrest specific 5 (GAS5) is significantly reduced in the serum of patients with type 2 diabetes mellitus (T2DM); therefore, to stabilize the level of GAS5 in T2DM, Shi et al. used the small molecule NP-C86, which can bind to GAS5 with high affinity and increase the levels of GAS5 and glucose uptake in the adipocytes of diabetic patients (40).
Conclusions
The lncRNA DINO stabilizes p53 to mediate the activation of the p53-Bax axis and regulate the sensitivity of LUAD to DDP. Together, our results suggest that research on the lncRNA DINO may provide a new understanding of the mechanisms of DDP sensitivity and help in the development of novel and effective strategies to treat NSCLC.
Acknowledgments
Funding: This work was supported by grants from the National Natural Science Foundation of China (Nos. 81974434 and 81903119), the Science and Technology Foundation of Guangdong Province (Nos. 2020A0505100038 and 2021A1515010793), the Science and Technology Program of Guangzhou City (No. 202201020097), the Affiliated Cancer Hospital & Institute of Guangzhou Medical University (No. 2020-YZ-01), and the Clinical Key Specialty Construction Project of Guangzhou Medical University (No. YYPT202017).
Footnote
Reporting Checklist: The authors have completed the ARRIVE reporting checklist. Available at https://jtd.amegroups.com/article/view/10.21037/jtd-23-465/rc
Data Sharing Statement: Available at https://jtd.amegroups.com/article/view/10.21037/jtd-23-465/dss
Peer Review File: Available at https://jtd.amegroups.com/article/view/10.21037/jtd-23-465/prf
Conflicts of Interest: All authors have completed the ICMJE uniform disclosure form (available at https://jtd.amegroups.com/article/view/10.21037/jtd-23-465/coif). The authors have no conflicts of interest to declare.
Ethical Statement: The authors are accountable for all aspects of the work in ensuring that questions related to the accuracy or integrity of any part of the work are appropriately investigated and resolved. Animal experiments were performed under a project license (No. 2018A0303130233) granted by institutional ethics board of Southern Medical University, in compliance with institutional guidelines for the care and use of animals.
Open Access Statement: This is an Open Access article distributed in accordance with the Creative Commons Attribution-NonCommercial-NoDerivs 4.0 International License (CC BY-NC-ND 4.0), which permits the non-commercial replication and distribution of the article with the strict proviso that no changes or edits are made and the original work is properly cited (including links to both the formal publication through the relevant DOI and the license). See: https://creativecommons.org/licenses/by-nc-nd/4.0/.
References
- Siegel RL, Miller KD, Wagle NS, et al. Cancer statistics, 2023. CA Cancer J Clin 2023;73:17-48. [Crossref] [PubMed]
- Seguin L, Durandy M, Feral CC. Lung Adenocarcinoma Tumor Origin: A Guide for Personalized Medicine. Cancers (Basel) 2022;14:1759. [Crossref] [PubMed]
- Ettinger DS, Wood DE, Aisner DL, et al. Non-Small Cell Lung Cancer, Version 3.2022, NCCN Clinical Practice Guidelines in Oncology. J Natl Compr Canc Netw 2022;20:497-530. [Crossref] [PubMed]
- Maconachie R, Mercer T, Navani N, et al. Lung cancer: diagnosis and management: summary of updated NICE guidance. BMJ 2019;364:l1049. [Crossref] [PubMed]
- Chen P, Liu Y, Wen Y, et al. Non-small cell lung cancer in China. Cancer Commun (Lond) 2022;42:937-70. [Crossref] [PubMed]
- Guo WJ, Zhang YM, Zhang L, et al. Novel monofunctional platinum (II) complex Mono-Pt induces apoptosis-independent autophagic cell death in human ovarian carcinoma cells, distinct from cisplatin. Autophagy 2013;9:996-1008. [Crossref] [PubMed]
- De Santis M, Bellmunt J, Mead G, et al. Randomized phase II/III trial assessing gemcitabine/carboplatin and methotrexate/carboplatin/vinblastine in patients with advanced urothelial cancer who are unfit for cisplatin-based chemotherapy: EORTC study 30986. J Clin Oncol 2012;30:191-9. [Crossref] [PubMed]
- Lu J, Wang W, Liu H, et al. Cisplatin induces calcium ion accumulation and hearing loss by causing functional alterations in calcium channels and exocytosis. Am J Transl Res 2019;11:6877-89. [PubMed]
- Jungwirth U, Xanthos DN, Gojo J, et al. Anticancer activity of methyl-substituted oxaliplatin analogs. Mol Pharmacol 2012;81:719-28. [Crossref] [PubMed]
- Eptaminitaki GC, Stellas D, Bonavida B, et al. Long non-coding RNAs (lncRNAs) signaling in cancer chemoresistance: From prediction to druggability. Drug Resist Updat 2022;65:100866. [Crossref] [PubMed]
- Li Y, Jiang T, Zhou W, et al. Pan-cancer characterization of immune-related lncRNAs identifies potential oncogenic biomarkers. Nat Commun 2020;11:1000. [Crossref] [PubMed]
- Schmitt AM, Chang HY. Long Noncoding RNAs: At the Intersection of Cancer and Chromatin Biology. Cold Spring Harb Perspect Med 2017;7:a026492. [Crossref] [PubMed]
- Flynn RA, Chang HY. Long noncoding RNAs in cell-fate programming and reprogramming. Cell Stem Cell 2014;14:752-61. [Crossref] [PubMed]
- Liao W, Du J, Wang Z, et al. The role and mechanism of noncoding RNAs in regulation of metabolic reprogramming in hepatocellular carcinoma. Int J Cancer 2022;151:337-47. [Crossref] [PubMed]
- She K, He S, Lu X, et al. LncRNA SNHG7 promotes non-small cell lung cancer progression and cisplatin resistance by inducing autophagic activity. J Thorac Dis 2023;15:155-67. [Crossref] [PubMed]
- Schmitt AM, Garcia JT, Hung T, et al. An inducible long noncoding RNA amplifies DNA damage signaling. Nat Genet 2016;48:1370-6. [Crossref] [PubMed]
- Huarte M. p53 partners with RNA in the DNA damage response. Nat Genet 2016;48:1298-9. [Crossref] [PubMed]
- Kastenhuber ER, Lowe SW. Putting p53 in Context. Cell 2017;170:1062-78. [Crossref] [PubMed]
- Fetoni AR, Paciello F, Troiani D. Cisplatin Chemotherapy and Cochlear Damage: Otoprotective and Chemosensitization Properties of Polyphenols. Antioxid Redox Signal 2022;36:1229-45. [Crossref] [PubMed]
- Ren N, Chen L, Li B, et al. Purified Tea (Camellia sinensis (L.) Kuntze) Flower Saponins Induce the p53-Dependent Intrinsic Apoptosis of Cisplatin-Resistant Ovarian Cancer Cells. Int J Mol Sci 2020;21:4324. [Crossref] [PubMed]
- Balakrishnan K, Burger JA, Quiroga MP, et al. Influence of bone marrow stromal microenvironment on forodesine-induced responses in CLL primary cells. Blood 2010;116:1083-91. [Crossref] [PubMed]
- Han MK, Song EK, Guo Y, et al. SIRT1 regulates apoptosis and Nanog expression in mouse embryonic stem cells by controlling p53 subcellular localization. Cell Stem Cell 2008;2:241-51. [Crossref] [PubMed]
- Gao Y, Ma H, Gao C, et al. Tumor-promoting properties of miR-8084 in breast cancer through enhancing proliferation, suppressing apoptosis and inducing epithelial-mesenchymal transition. J Transl Med 2018;16:38. [Crossref] [PubMed]
- Wang W, Zhao M, Cui L, et al. Characterization of a novel HDAC/RXR/HtrA1 signaling axis as a novel target to overcome cisplatin resistance in human non-small cell lung cancer. Mol Cancer 2020;19:134. [Crossref] [PubMed]
- Zhong YF, Cheng J, Liu Y, et al. DNA Nanostructures as Pt(IV) Prodrug Delivery Systems to Combat Chemoresistance. Small 2020;16:e2003646. [Crossref] [PubMed]
- Wang DY, Hong Y, Chen YG, et al. PEST-containing nuclear protein regulates cell proliferation, migration, and invasion in lung adenocarcinoma. Oncogenesis 2019;8:22. [Crossref] [PubMed]
- Poels KE, Schoenfeld AJ, Makhnin A, et al. Identification of optimal dosing schedules of dacomitinib and osimertinib for a phase I/II trial in advanced EGFR-mutant non-small cell lung cancer. Nat Commun 2021;12:3697. [Crossref] [PubMed]
- Hui R, Garon EB, Goldman JW, et al. Pembrolizumab as first-line therapy for patients with PD-L1-positive advanced non-small cell lung cancer: a phase 1 trial. Ann Oncol 2017;28:874-81. [Crossref] [PubMed]
- Sanda MG, Feng Z, Howard DH, et al. Association Between Combined TMPRSS2:ERG and PCA3 RNA Urinary Testing and Detection of Aggressive Prostate Cancer. JAMA Oncol 2017;3:1085-93. [Crossref] [PubMed]
- Shao Y, Ye M, Jiang X, et al. Gastric juice long noncoding RNA used as a tumor marker for screening gastric cancer. Cancer 2014;120:3320-8. [Crossref] [PubMed]
- Khanal T, Leung YK, Jiang W, et al. NR2E3 is a key component in p53 activation by regulating a long noncoding RNA DINO in acute liver injuries. FASEB J 2019;33:8335-48. [Crossref] [PubMed]
- Sharma S, Munger K. Expression of the Long Noncoding RNA DINO in Human Papillomavirus-Positive Cervical Cancer Cells Reactivates the Dormant TP53 Tumor Suppressor through ATM/CHK2 Signaling. mBio 2020;11:e01190-20. [Crossref] [PubMed]
- Sharma S, Munger K. KDM6A-Mediated Expression of the Long Noncoding RNA DINO Causes TP53 Tumor Suppressor Stabilization in Human Papillomavirus 16 E7-Expressing Cells. J Virol 2020;94:e02178-19. [Crossref] [PubMed]
- Wu K, Liu Y, Zhang H, et al. DNA damage induced long non-coding RNA (DINO) down-regulates the expression of MMP2 and inhibits the invasion and migration of human chorionic trophoblast cells. Xi Bao Yu Fen Zi Mian Yi Xue Za Zhi 2022;38:736-42. [PubMed]
- Marney CB, Anderson ES, Baum R, et al. A Unique Spectrum of Spontaneous Tumors in Dino Knockout Mice Identifies Tissue-Specific Requirements for Tumor Suppression. Cells 2022;11:1818. [Crossref] [PubMed]
- Godefroy N, Bouleau S, Gruel G, et al. Transcriptional repression by p53 promotes a Bcl-2-insensitive and mitochondria-independent pathway of apoptosis. Nucleic Acids Res 2004;32:4480-90. [Crossref] [PubMed]
- Huang K, O'Neill KL, Li J, et al. BH3-only proteins target BCL-xL/MCL-1, not BAX/BAK, to initiate apoptosis. Cell Res 2019;29:942-52. [Crossref] [PubMed]
- Follis AV, Llambi F, Merritt P, et al. Pin1-Induced Proline Isomerization in Cytosolic p53 Mediates BAX Activation and Apoptosis. Mol Cell 2015;59:677-84. [Crossref] [PubMed]
- Gai C, Liu C, Wu X, et al. MT1DP loaded by folate-modified liposomes sensitizes erastin-induced ferroptosis via regulating miR-365a-3p/NRF2 axis in non-small cell lung cancer cells. Cell Death Dis 2020;11:751. [Crossref] [PubMed]
- Shi Y, Parag S, Patel R, et al. Stabilization of lncRNA GAS5 by a Small Molecule and Its Implications in Diabetic Adipocytes. Cell Chem Biol 2019;26:319-330.e6. [Crossref] [PubMed]
(English Language Editor: A. Kassem)