Store-operated calcium entry enhances the polarization and chemotaxis of neutrophils in the peripheral venous blood of patients with bronchial asthma by upregulating ERM protein
Highlight box
Key findings
• The polarization and chemotaxis of neutrophils of patients with asthma are abnormal. This may be due to the abnormal expression and distribution of Ezrin, radixin and moesin proteins (ERMs) and F-actin as a result of abnormal store-operated calcium entry (SOCE) function.
What is known and what is new?
• Neutrophils can be recruited rapidly and are abundant in the airways of asthmatics.
• This study reveals the abnormal polarization and chemotaxis of neutrophils in patients with asthma and explores the underlying mechanism.
What is the implication, and what should change now?
• In this study, the directed polarization and chemotaxis of neutrophils in asthmatic patients were significantly higher than those in healthy controls, and the expression and distribution of cytoskeletal proteins F-actin and ERM were abnormal. This may be due to an increased Ca2+ level caused by abnormal SOCE function in the neutrophils of patients with asthma.
Introduction
Bronchial asthma (asthma) is one of the most common chronic respiratory diseases. Asthma is a chronic inflammatory disease involving a variety of cells and cellular components. Its clinical manifestations are repeated wheezing and shortness of breath with or without chest tightness or cough and accompanied by airway hyperresponsiveness and variable airflow restriction. The progression of asthma can lead to changes in airway structure, specifically airway remodeling (1). According to the Global Burden of Disease Study 2015 (GBD 2015) , there were 358 million people with asthma worldwide in 2015, and the prevalence was 12.6% higher than that in 1990 (2). The overall prevalence of asthma has been also increasing (3).
Asthma is considered a heterogeneous disease, and gene–environment interactions are involved in its emergence and progression (4,5). A variety of environmental, biological, and social factors play an important role in the occurrence of asthma. Risk factors for asthma include nutrition (5,6), inhaled and ingested allergens (7), pollution—especially traffic-related air pollution (5)—tobacco smoke, and microbial and psychosocial factors (8). The interaction of many factors makes the understanding the pathogenesis of asthma complicated.
Neutrophils are the most abundant white blood cells, the fastest moving cells in the body, and an important component of the innate immune response (9). Many diseases and conditions, including asthma (10), rheumatoid arthritis (11), sepsis (12), atherosclerosis (13), ischemia–reperfusion injury, viral myocarditis, allergic reaction (14), inflammatory skin diseases, and the occurrence and metastasis of tumors, are all related to the migration function of neutrophils (15).
Many studies have shown that the chemotaxis and polarization of neutrophils in the peripheral venous blood of patients with bronchial asthma are abnormal. Sackmann et al. (16) established a microfluidic device and analyzed the chemotaxis of neutrophils in the peripheral venous blood of patients with asthma. They determined that neutrophils from asthmatic patients migrate significantly more slowly toward the chemoattractant compared with nonasthmatic patients (P=0.002). Their results also showed that the sensitivity and specificity for a diagnosis of asthma were 96% and 73%, respectively, when a cutoff value for the chemotaxis rate of 1.55 µm/min was used. They suggested that the neutrophil chemotaxis rate may be a potential biomarker of asthma/ However, Mosca et al. reported that the phagocytic and chemotactic activities of neutrophils in the peripheral blood of patients with asthma with stable and acute disease were higher than those in healthy persons without asthma and in patients with allergies without asthma (17). Dong and colleagues confirmed Th1-Th2-Th17 imbalances in neutrophilic asthma and children with asthma. The former has more severe immune imbalances, are more difficult to treat and have longer hospital stays. The levels of ICOSL in peripheral blood and bronchoalveolar fluid (BALF) samples of children with neutrophil asthma were higher than those of normal people (18). Our previous experiment showed that the directional polarization rate of neutrophils in the peripheral venous blood of patients with asthma was significantly higher than that of healthy, nonsmoking persons (19).
Recent studies have shown that neutrophils exhibit considerable plasticity and polarization. They have a highly variable transcriptome profile, depending on their tissue location and microenvironment (20). Tumor-associated neutrophils are thought to be polarized between antitumor and tumor-inducing neutrophilic phenotypes (21). Sun and colleagues (22) demonstrated that IL-33 induces Th2/ th9 type polarization of neutrophils. In mouse models, these polarized neutrophils contribute to the pathogenesis of airway allergic inflammation. They provide additional evidence of the differentially polarized neutrophils produced by cytokines and chemokines.
Neutrophil movement toward the chemical factors, chemokines, is known as chemotaxis. Neutrophils represent the first line of cellular defense against invading microorganisms by rapidly moving across the blood–endothelial cell (EC) barrier and exerting effector cell functions (23). There are 2 types of neutrophil chemokines. The first are factors released by tissue injury, such as fibrin fragments and collagen, complement activation products, and immune cytokines such as interleukin 8 (IL-8) (24). The second are polypeptide from microorganisms containing N-formyl methionine residues, such as N-formyl-methionine-leucine-phenylalanine (fMLP) (25,26). In this study, fMLP and IL-8 were used as chemokines to stimulate neutrophils, and experiments were conducted by simulating exogenous and endogenous chemokines, respectively.
When neutrophils are stimulated by chemokines, they develop a polarized morphology; that is, they form a lamellipodium and a tail foot which moves them toward the chemokines. The most obvious manifestation of cell shape change is the change of the cell longitudinal axis length (27). Pseudopodia formation is the initial step of neutrophil polarization. Ezrin is the prototype of the ezrin protein subfamily members, which are mainly present in the brush border and placental microvilli. It is currently believed that there are 3 ezrin proteins in vertebrates, ezrin, radixin, and moesin, which are collectively known as ERM. Ezrin plays an important role in the polarization of neutrophils. Its main mechanism involves blocking the Rac signaling pathway and inhibiting the formation of pseudopodia. (I) The early stage of pseudopodia formation involves anchoring of ERM family proteins in the spherical pseudopodia structure (28). (II) When the ERM protein is found missing in T cells, pseudopodia cannot be formed (29). (III) A recent study showed that a key role of moesin is limiting the formation of pseudopodia and helping to maintain the polarization of neutrophils during movement (30). (IV) The binding of phosphorylation and membrane induces the active formation of ERM proteins and moesin which prevents cell polarization by inhibiting GPR35, the small GTPases Rac, Rho, and Cdc42 (27,31,32).
At present, the classical signaling pathway of neutrophil polarity is the PI3K-dependent pathway. When stimulated by chemoattractants for a few minutes, neutrophils change the shape: The longitudinal axis of neutrophils was changed, the leading edge was broad and the trailing edge was caudate (33). Then, neutrophils begin to migrate toward the chemoattractants, this migration is resulting from a polarization of the cytoskeleton, which is driven by the asymmetric distribution of signaling molecules, these signaling molecules have been deemed to both phosphoinositide 3-kinase (PI3K)-dependent and -independent signaling pathways in vitro (34).
Breaking the symmetry of cell morphology requires cells to translate extracellular signals and establish a polarized distribution of signal molecules in the cells, such as an asymmetric distribution of proteins and lipids, thus remodeling the cytoskeletal structure. An important signal molecule in the physiological process of cells is Ca2+, and it has been shown to be involved in the polarization of neutrophils. As nonexcitatory cells, neutrophils’ Ca2+ signaling is mainly affected by an external Ca2+ influx caused by the emptying of Ca2+ stored in the endoplasmic reticulum, which is called the “store-operated calcium entry” (SOCE) mechanism. SOCE is influenced by Ca2+-sensing matrix interaction molecules (STIM) 1 and 2 in the endoplasmic reticulum, and Orai1 molecules on the cell membrane (35). SOCE is the key mechanism that regulates the increase of Ca2+ in nonexcitatory cells (36,37). However, no studies have examined if the polarization of neutrophils in the venous blood of patients with bronchial asthma is abnormal or if any changes are related to SOCE.
SOCE and Orai1 are upstream transcription factors such as activated T nuclear factor that promote inflammation
Ca2+ is a key second messenger involved in a variety of physiological and pathological processes involved in airway inflammation and remodeling in asthma (38). SOCE is the most important extracellular Ca2+ influx pathway for many immune and structural cells involved in asthma (39). Compared with the control mice, the expression of STIM1 and Orai1 in the lung of asthmatic mice was up-regulated. In addition, SOCE blockers SKF-96365, BTP-2, and RP3128 reduced airway inflammation and airway hyperreactivity in asthmatic mouse models (40). Wrennall and colleagues (41) have produced a novel peptide that reduces Orai1 levels in the lungs, thereby reducing inflammation in mouse models of asthma.
To date, studies of neutrophil chemotaxis and migration have focused on neutrophil chemotaxis and abnormal cell surface adhesion molecules, such as the PI3K pathway. However, the origin of this abnormal migration, and the abnormal polarization of neutrophils, has not been reported yet. This study conducted an in-depth study on the relationship between abnormal neutrophil polarization and SOCE in asthmatic, and also providing a new vision and target for clinical diagnosis, treatment and prevention for asthma suffers.
In this study we examined the hypothesis that SOCE can enhance the polarization and chemotaxis of neutrophils in patients with asthma by upregulating the expression and distribution of ERM proteins to thus aggravate the airway inflammatory reaction. We present the following article in accordance with the MDAR reporting checklist (available at https://jtd.amegroups.com/article/view/10.21037/jtd-23-467/rc).
Methods
Reagents and methods
The chemicals fMLP, IL-8, thapsigargin (TG), CaCl2, and deguelin were purchased from Sigma Chemical Company. Fluo-4 AM was purchased from Invitrogen (Thermo Fisher Scientific, Waltham, MA, USA). Antibodies against ERM, STIM1, STIM2, and Orai1 were purchased from Santa Cruz Biotechnology.
Study participants
Based on the 2019 Global Initiative for Asthma (GINA) (42) diagnostic criteria, 30 bronchial patients with asthma with stable disease treated at the Department of Respiratory and Critical Care Medicine of Huizhou Central People’s Hospital were recruited for this study from October 2019 to May 2021. Thirty healthy persons without asthma were recruited to serve as a control group. Persons in both groups ranged in age from 18 to 75 years old. People in the control group had no chronic airway disease, had taken no medications in the previous 8 weeks, and had no history of smoking. Patients with asthma were clinically stable and had no medication changes at least 8 weeks prior to study participation. Clinically, physiologically, and radiologically exclude evidence of alternative and concomitant disease. This study followed the ethical guidelines set forth by the Department of Respiratory at Huizhou Central People’s Hospital. All patients who participated in this study signed an informed consent form, and this study was approved by the Ethics Committee of Huizhou Central People’s Hospital (No. LLBA201913A). The study was conducted in accordance with the Declaration of Helsinki (as revised in 2013).
Isolation of blood neutrophils
Neutrophils were isolated from fresh whole blood samples using the density gradient technique (43). Purified neutrophils (1×106 cells/mL) were resuspended in D-Hanks’ medium without CaCl2 prior to use. Wright-Giemsa staining was used to confirm the presence of neutrophils, and trypan blue exclusion was used to confirm a >98% cell viability rate.
Evaluation of cell shape
Cell morphology was detected using an Olympus IX51 microscope (Olympus Corporation, Tokyo, Japan) and 40× objective lens. After stimulated by the gradient concentrations of fMLP or IL-8 in a Zigmond chamber (Neuroprobe, Gaithersburg, MD, USA) for 15 minutes (the point at which cell polarizability reaches its peak), at least 250 cells were observed with inverted microscopy to determine the proportion of polarized cells and to classify the cells into 3 categories: spherical, nonpolar, and polar (44,45). All experiments were performed in triplicate, and shape polarization was defined as a ratio of cell length to cell width >1.5 (45).
Analyses of cell polarity
Neutrophils (1×106) were suspended in 20 µL of D-Hanks’, dispensed onto coverslips (48 mm × 60 mm) at room temperature, and allowed to adhere for 5 minutes. Coverslips were inverted onto a Zigmond chamber (Neuroprobe) and immediately exposed to an fMLP gradient (0–100 nM). Digital images of neutrophils were captured in 5 random fields every 30 seconds for 15 minutes using an Olympus IX71 inverted microscope (Olympus Corporation). The recorded images were analyzed with Image-Pro Plus 6.0 software (Media Cybernetics Inc., Rockville, MD, USA).
Neutrophil polarity was analyzed by a technician who was not aware of the treatment and was characterized as follows: spontaneous polarization—the number of polarized neutrophils prior to exposure to the fMLP concentration gradient; directional polarization—the number of polarized neutrophils positioned at a 45-degree angle along the gradient (46).
Intracellular Ca2+ measurement
Neutrophils were labeled with a fluorescent Ca2+ indicator, Fluo-4 AM (2 µM). Intracellular Ca2+ was monitored using a confocal laser scanning microscope (Olympus FV1000-IX71, Olympus Corporation). In certain experiments, neutrophils were maintained in Ca2+ unbuffered fluid for 30 minutes, followed by Ca2+ image recording.
Western blotting
Protein samples (30 µg) were separated using sodium dodecyl-sulfate polyacrylamide gel electrophoresis (SDS-PAGE), transferred to polyvinylidene fluoride (PVDF) membranes, and blocked. The following primary antibodies were used: mouse polyclonal anti-STIM1 (1:1,000; Abcam, Cambridge, UK), anti-STIM2 (1:1,000; Abcam), and anti-Orai1 (1:1,000; Abcam). The membranes were incubated with secondary antibodies (1:5,000) (Zhongshan Biotech, Zhongshan, China), and protein band density was quantified using a Gel-Prep Analyzer (Media Cybernetics).
Reverse transcription polymerase chain reaction
First, total RNA was extracted. The isolated neutrophils were rinsed 3 times with precooled phosphate-buffered saline (PBS), and then 1 mL of TRIzol was added. After standing for 5 minutes, the lysed cells were suspended on an ice cube tray. Then, acute 3-phase separation was performed to precipitate the RNA, which was then followed by washing, dissolving, and diluting of the RNA. A 1.5-µL sample was extracted and analyzed with an ND-1000 full wavelength ultraviolet/visible light scanning spectrophotometer, and the A260/A280 values and concentrations were used to determine the total RNA concentration. The complement DNA (cDNA) was synthesized with reverse transcription, and then quantitative reverse transcription polymerase chain reaction (qRT-PCR) was performed using the standard 3-step method. A complex hole was made for all reactions to analyze the dissolution curves. A 5-µL RNA sample was taken for agarose gel electrophoresis, and the gel was photographed using a Bio-Rad gel imaging system (Bio-Rad Laboratories). The experimental results were statistically analyzed using GraphPad Prism 8 version 8.4.2 software (San Diego, CA, USA).
Statistical analyses
Statistical analyses were performed using SPSS version 23.0 software (IBM Corporation, Armonk, NY, USA). Data were analyzed with t test or 1-way analysis of variance (ANOVA) and are expressed as the mean ± standard error of the mean (SEM). Where there was a significant difference among groups, pairwise comparisons were performed using the Newman-Keuls test. Values of P<0.05 were considered statistically significant.
Results
Patient demographic data
Since it is known that asthma is associated with an increase in pulmonary and systemic inflammation, we carefully selected healthy individuals who were never-smokers, which were tested as a normal lung function and without respiratory symptoms as control participants. All control participants were age- and sex-matched because age and sex are both believed to affect many facets of neutrophil function, including migration (47-49).
Patient and control participant data are summarized in Table 1. The age and sex distribution of the 2 groups was similar. Patients with asthma had a higher eosinophil count and fractional exhaled nitric oxide (FeNO) level as well as a lower of first second forced expiratory volume predicted (FEV1%) and first second forced expiratory volume/forced vital capacity (FEV1/FVC) compared with healthy control participants.
Table 1
Group characteristics | Healthy control | Controlled asthma |
---|---|---|
Total number of participants | 30 | 30 |
Age, years, mean [range] | 43 [35–63] | 45 [32–65] |
Number of males | 12 | 14 |
Eosinophil count of peripheral venous blood, N/mm3, mean ± SD | 154±45** | 319±86 |
Predicted FEV1, %, mean [range] | 97 [92–105]** | 86 [80–92] |
FEV1/FVC, mean [range] | 88 [82–94]** | 68 [57–88] |
FeNO, ppb, mean ± SD | 13.33±6.34** | 42.63±11.68 |
**, significant analysis of variance test (P<0.01) between the groups. FEV1, forced expiratory volume in the first second; FVC, forced vital capacity. FeNO, fractional exhaled nitric oxide; SD, standard deviation.
Based on our preliminary experimental analysis, we used a concentration of 100 nM fMLP and a concentration of 10 nM IL-8 in the experiments. We observed cell morphology using a Zigmond chamber and counted the number of polarized cells to compare the polarization between patients with asthma and healthy persons. The polarizations of neutrophils of the 2 groups are shown in Figure 1. When neutrophils were exposed to a concentration gradient of fMLP or IL-8, their morphology changed, and the cells developed a polar shape with a wide head and a narrow tail, with the polar head being oriented toward the concentration gradient of fMLP and IL-8. Without stimulation of the fMLP concentration gradient, the spontaneous polarization rate of neutrophils in the peripheral blood of patients with stable asthma was not different than that of healthy control participants (7.99%±1.31% vs. 5.76%±0.91%; N=30; Figure 1A,1B). After stimulation by an fMLP concentration gradient (0–100 nM) for 15 minutes, the directional polarization rate of neutrophils in the venous blood of patients with asthma was significantly increased (7.44%±1.31% vs. 2.75%±0.82%; P<0.01; N=30; Figure 1C,1D).
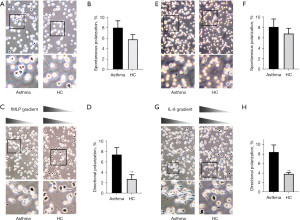
Bedouhene, Yadav and their colleagues (50,51) proposed that fMLP, as the terminal chemokine of neutrophils, activates cells through the PI3K-independent P38 MAPK pathway, while the intermediate chemokine IL-8 activates cells through the PI3K-dependent signaling pathway. If terminal and intermediate chemokines are present at the same time, the PI3K-independent P38MAPK pathway would be dominant. Therefore, in this study IL-8 was used as a chemotactic agent to stimulate neutrophils.
Without stimulation of an IL-8 concentration gradient, the spontaneous polarization rate of neutrophils in the blood of patients with asthma was not significantly different from that of healthy control participants (8.13%±1.51% vs. 6.84%±1.02%; N=30; Figure 1E,1F). Under stimulation of an IL-8 concentration gradient (0–10 nM), the directional polarization rate of neutrophils in the blood of patients with asthma was significantly increased (8.44%±1.47% vs. 3.83%±0.32%; P<0.01; Figure 1G,1H).
Aberrant chemotaxis polarization in bronchial patients with asthma
Transwell assays were used to compare the chemotactic function of neutrophils in the blood of patients with asthma and healthy control participants. The number of neutrophils migrating toward the chemotactic stimuli in the 2 groups was counted (Figure 2). Compared with the healthy control group, the number of neutrophils migrating toward fMLP in patients with asthma was significantly increased (166.42±14.01 vs. 105.86±13.74; P<0.01; Figure 2A,2B). Similar results were obtained when IL-8 was used as the chemotactic stimuli (149.55±12.20 vs. 103.66±18.72; P<0.01; Figure 2C,2D).
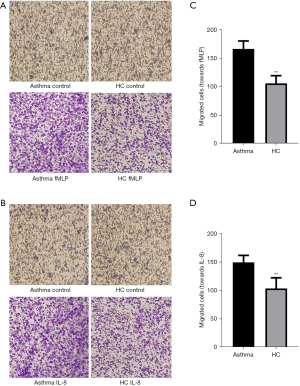
Neutrophils in bronchial patients with asthma exhibited more obvious F-actin polymerization
Polymeric F-actin is a signature skeleton protein molecule in the process of cell polarity, and its intracellular distribution is the first step of cell polarity change. Polymeric F-actin immunofluorescence experiments were conducted to further observe the formation of the polar morphology of neutrophils in peripheral blood of patients with asthma induced by chemotactic stimuli. The difference of intracellular distribution of polymeric F-actin in the neutrophils of patients with asthma induced by fMLP and IL-8 was analyzed.
Laser confocal imaging results are shown in Figure 3. Rhodamine phalloidin-labeled polymeric F-actin exhibited red fluorescence, and DAPI-stained nuclei exhibited blue fluorescence. Red fluorescence with a circular and symmetrical distribution appeared on the plasma membrane of unstimulated neutrophils, and the fluorescence intensity was weak. After 5 minutes of stimulation with 100 nM of fMLP, the red fluorescence in the plasma membrane of neutrophils of patients with asthma exhibited an obvious asymmetric distribution, and the fluorescence in the cell head was strong and abundant. There was also marked red fluorescence in cell tails. There was no change in the fluorescence of neutrophils of the healthy control group. These results indicate that a large amount of polymerized F-actin in the neutrophils of patients with asthma facilitates the formation of cell polar morphology (Figure 3). Similar results were obtained when the chemotactic stimulator was 10 nM of IL-8.
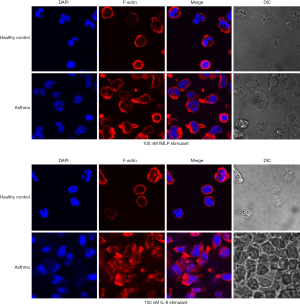
Abnormal expression and distribution of ezrin in the neutrophils of patients with asthma under chemotactic stimulation
Ezrin plays an important role in controlling cell morphology, and white blood cells mainly express moesin and ezrin, but do not express radixin (52). When white blood cells are activated, ERM is thought to redistribute membrane proteins from microvilli to the tail foot during polarization and transduce signals that affect adhesion and other reactions.
Immunofluorescence assays and qRT-PCR were performed to observe the distribution and expression of the ezrin protein on the surface of neutrophils in patients with asthma.
As shown in Figure 4, after stimulation with 100 nM fMLP for 5 minutes, the red fluorescence–labeled by ezrin antibody exhibited an obvious asymmetric distribution in plasma membrane of neutrophils of patients with asthma and was primarily present in the cell tails. On the other hand, there was no asymmetric distribution of fluorescence of neutrophils in healthy control participants. Similar results were obtained when the neutrophils were stimulated by 10 nM of IL-8 for 5 minutes.
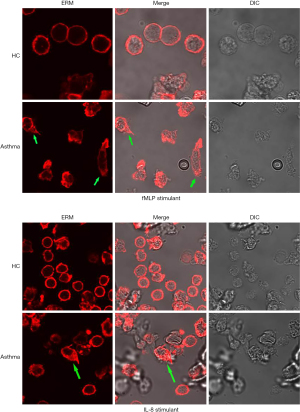
RT-PCR was used to examine the messenger RNA (mRNA) expression of moesin and ezrin, which are the main components of ezrin, in neutrophils of the 2 groups, as shown in Figure 5. The results indicated that the mRNA expression of moesin and ezrin were significantly increased in the neutrophils of patients with asthma.
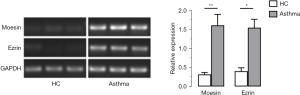
Enhanced calcium influx in neutrophils from patients with asthma
In order to determine whether neutrophil polarization and signal transduction in patients with asthma are due to changes in cytoplasmic Ca2+ influx, Fluo-4-mediated Ca2+ imaging was used experimentally, in which TG, a SERCA pump inhibitor, is a very effective tool for studying SOCE (53,54), was introduced. As shown in Figure 6A-6C, supplementation of 2 mM of Ca2+ in the medium may indicate SOCE. Basal levels of cytoplasmic Ca2+ were restored by 2 mM Ca2+ supplementation prior to TG addition, normalized to ΔF/F0~0, and ΔF/F0 was used as a measure of increased cytoplasmic Ca2+ influx.
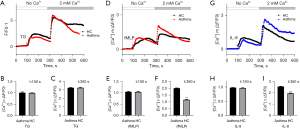
As shown in the right panel of Figure 6D-6F, when the chemotactic stimulus was changed to 100 nM of fMLP there was only a relatively small increase in cytosolic Ca2+ in neutrophils from patients with asthma compared to those in healthy control participants. However, interestingly, the readdition of Ca2+ in extracellular matrix resulted in a rapid Ca2+ influx into the neutrophils, and this increase was about 70% greater in patients with asthma compared with that in healthy control participants. When the chemotactic stimulus was 10 nM of IL-8, readdition of extracellular Ca2+ also resulted in a rapid Ca2+ influx into the cells, which was about 30% greater in patients with asthma compared with that in healthy control participants, as shown in Figure 6G-6I.
Expression of SOCE components STIM1, STIM2 and Orai1 in neutrophils of asthmatic patients
Western blotting showed that asthmatic patients had higher levels of STIM1, STIM2 and Orai1 in their neutrophils than healthy age - and sex-matched controls (Figure 7).
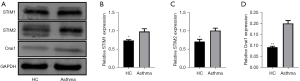
Discussion
Asthma comprises a heterogeneous group of respiratory inflammatory disorders characterized by a similar clinical pattern of cough, wheezing, and reversible airway obstruction (55,56). According to an inflammatory phenotype classification (57), allergic asthma belongs to the “eosinophilic phenotype”. Notably, while patients with more than 3% sputum eosinophils are considered eosinophilic, up to 60% sputum neutrophils can be present (56-58). A study that has examined the role of eosinophils in allergic asthma pathogenesis have underscored a potential proinflammatory, deleterious effect that facilitates type 2–mediated disease development (59).
Neutrophil chemotaxis requires a binding of chemokines to receptors, and neutrophils reach the chemotactic site through the processes of recognition, adhesion, rolling, and migration within blood vessels (60). Increased levels of the chemokine IL-8 can be detected in the sputum and nasal secretions of patients with asthma (61). The chemotactic effect of IL-8 causes neutrophils to migrate across endothelial cells via a combination of selectin and integrin and the secretion of protease to penetrate basement membrane, wherein they ultimately reach the inflammatory site and participate in the airway inflammatory reaction (62). Many studies reported the abnormal chemotaxis of neutrophils in the peripheral venous blood of patients with asthma (16,17). However, there has been little research exploring whether the polarization of neutrophils in the peripheral venous blood of patients with asthma is abnormal. The results of this study showed that stimulation with the chemokines fMLP and IL-8 significantly increased the directional polarization rate and chemotaxis of neutrophils in the venous blood of patients with controlled asthma as compared with that of normal healthy control participants. These results were consistent with those of staining for polymerized F-actin via immunofluorescence which showed a more obvious polar distribution of F-actin in the neutrophils of patients with asthma. F-actin recombines at the anterior and posterior pole cells simultaneously. The possible explanation for this is that the anterior and posterior pole cells contain different actin assemblies (63).
ERMs are plasma membrane organizers that connect actin cytoskeletons to the cytoplasmic tails of transmembrane proteins, many of which are adhesion receptors, to regulate the formation of f-actin structures (e.g., microspikes and microvilli) (64). ERMs also affect signal transmission from the plasma membrane to the cell through septal activation of small gtpase Rho, Rac, and Cdc42. Ezrin and moesin are ERMs that are highly expressed in white blood cells (65).
The front pole of polarized neutrophils is primarily composed of actin gathering in the protuberant part, and the rear pole is a complex of actin and myosin. This different aggregation pattern of actin plays an important role in transmitting and regulating intracellular signals. Our RT-PCR results also showed that the expression of moesin and ezrin were significantly increased in the neutrophils of patients with asthma. Taken together, the evidence regarding the morphological changes of cells and F-actin polymerization suggests that the abnormal distribution and function of ERM plays an important role in the “excessive” polarization of neutrophils in patients with asthma.
Based on the aforementioned results, we speculated that neutrophils in the venous blood of patients with controlled asthma are in an excessive “aggressive” state. This state leads to a persistent and difficult-to-control airway inflammatory reaction in patients with asthma, resulting in persistent airflow restriction and lung tissue damage (66). The abnormal expression and distribution of ERM is an important step in the hyperpolarization of neutrophils in patients with asthma and may be used as a marker for the diagnosis and treatment of asthma in the future.
The change of intracellular free calcium concentration is involved in the physiological and pathological responses of neutrophils to various stimuli, such as cell polarization, migration, the production of reactive oxygen species, and the birth and death of cells. Many studies have suggested that calcium influx via the SOCE mechanism is the primary mechanism for increasing intracellular free calcium ions and is involved in various physiological and pathological processes of neutrophils (36,67,68). Neutrophil polarization is the basis of chemotactic function (69), and SOCE plays an important role in the process of neutrophil polarization, which also affects neutrophil chemotactic function. Two protein matrix interaction molecules, STIM1 and STIM2, and Orai1 are considered essential for SOCE (70).
Our results showed there was little difference in the increase of intracellular calcium caused by calcium emptying of the endoplasmic reticulum stimulated by TG between patients with controlled asthma and healthy control participants. Therefore, we speculated that there was no difference in the function of the SOCE channel of neutrophils between the 2 groups. However, when fMLP or IL-8 was used as a stimulus, the intracellular calcium level of neutrophils in patients with asthma due to the SOCE was significantly higher than that of healthy control participants. Western blotting subsequently showed that protein expression levels of STIM1, STIM2, and Orai1, the main functional components of SOCE, were significantly greater in the neutrophils of patients with asthma compared to those of healthy control participants. This suggests that abnormal components and functions of SOCE play a role in the pathogenesis of asthma. The increased expression of the 3 key protein components of SOCE might indicate a susceptibility of the individual to asthma.
Conclusions
The results of this study showed that the directional polarization and chemotaxis of neutrophils in the venous blood of patients with asthma are significantly higher than those in healthy control participants, and the expression and distribution of cytoskeletal proteins F-actin and ERM were abnormal. This may be due to an increased Ca2+ level caused by abnormal SOCE function in the neutrophils of patients with asthma. These findings may help further the understanding of the pathophysiological mechanism of asthma and aid in the development of biomarkers for asthma.
Acknowledgments
Funding: This study was supported by funding from the National Natural Science Foundation of China (No. 82270024) and the Priority Support Foundation of the Huizhou Municipal Central Hospital.
Footnote
Reporting Checklist: The authors have completed the MDAR reporting checklist. Available at https://jtd.amegroups.com/article/view/10.21037/jtd-23-467/rc
Data Sharing Statement: Available at https://jtd.amegroups.com/article/view/10.21037/jtd-23-467/dss
Peer Review File: Available at https://jtd.amegroups.com/article/view/10.21037/jtd-23-467/prf
Conflicts of Interest: All authors have completed the ICMJE uniform disclosure form (available at https://jtd.amegroups.com/article/view/10.21037/jtd-23-467/coif). The authors have no conflicts of interest to declare.
Ethical Statement: The authors are accountable for all aspects of the work, including ensuring that any questions related to the accuracy or integrity of any part of the work have been appropriately investigated and resolved. All patients who participated in this study signed an informed consent form, and this study was approved by institutional ethics board of Huizhou Central People’s Hospital (No. LLBA201913A). The study was conducted in accordance with the Declaration of Helsinki (as revised in 2013).
Open Access Statement: This is an Open Access article distributed in accordance with the Creative Commons Attribution-NonCommercial-NoDerivs 4.0 International License (CC BY-NC-ND 4.0), which permits the non-commercial replication and distribution of the article with the strict proviso that no changes or edits are made and the original work is properly cited (including links to both the formal publication through the relevant DOI and the license). See: https://creativecommons.org/licenses/by-nc-nd/4.0/.
References
- Asthma group of Chinese Throacic Society. Guidelines for bronchial asthma prevent and management(2020 edition) Asthma group of Chinese Throacic Society. Zhonghua Jie He He Hu Xi Za Zhi 2020;43:1023-48. [PubMed]
- Global, regional, and national deaths, prevalence, disability-adjusted life years, and years lived with disability for chronic obstructive pulmonary disease and asthma, 1990-2015: a systematic analysis for the Global Burden of Disease Study 2015. Lancet Respir Med 2017;5:691-706. [Crossref] [PubMed]
- Song WJ, Kang MG, Chang YS, et al. Epidemiology of adult asthma in Asia: toward a better understanding. Asia Pac Allergy 2014;4:75-85. [Crossref] [PubMed]
- Best KP, Sullivan TR, Palmer DJ, et al. Prenatal omega-3 LCPUFA and symptoms of allergic disease and sensitization throughout early childhood - a longitudinal analysis of long-term follow-up of a randomized controlled trial. World Allergy Organ J 2018;11:10. [Crossref] [PubMed]
- Lavigne É, Bélair MA, Rodriguez Duque D, et al. Effect modification of perinatal exposure to air pollution and childhood asthma incidence. Eur Respir J 2018;51:1701884. [Crossref] [PubMed]
- Hansen S, Strøm M, Maslova E, et al. Fish oil supplementation during pregnancy and allergic respiratory disease in the adult offspring. J Allergy Clin Immunol 2017;139:104-111.e4. [Crossref] [PubMed]
- Incorvaia C, Riario-Sforza GG. Allergy testing in the diagnosis of asthma. Lancet Respir Med 2015;3:e16. [Crossref] [PubMed]
- Flanigan C, Sheikh A. Prenatal maternal psychosocial stress and offspring's asthma and allergic disease: A systematic review and meta-analysis. Clin Exp Allergy 2018;48:403-14. [Crossref] [PubMed]
- Summers C, Rankin SM, Condliffe AM, et al. Neutrophil kinetics in health and disease. Trends Immunol 2010;31:318-24. [Crossref] [PubMed]
- Singhal A, Kumar S. Neutrophil and remnant clearance in immunity and inflammation. Immunology 2022;165:22-43. [Crossref] [PubMed]
- Hidalgo A, Libby P, Soehnlein O, et al. Neutrophil extracellular traps: from physiology to pathology. Cardiovasc Res 2022;118:2737-53. [Crossref] [PubMed]
- Gonzalez RJ, von Andrian UH. Quo vadis, neutrophil? Cell 2022;185:759-61. [Crossref] [PubMed]
- Kuley R, Stultz RD, Duvvuri B, et al. N-Formyl Methionine Peptide-Mediated Neutrophil Activation in Systemic Sclerosis. Front Immunol 2021;12:785275. [Crossref] [PubMed]
- Özcan A, Boyman O. Mechanisms regulating neutrophil responses in immunity, allergy, and autoimmunity. Allergy 2022;77:3567-83. [Crossref] [PubMed]
- Ley K, Hoffman HM, Kubes P, et al. Neutrophils: New insights and open questions. Sci Immunol 2018;3:eaat4579. [Crossref] [PubMed]
- Sackmann EK, Berthier E, Schwantes EA, et al. Characterizing asthma from a drop of blood using neutrophil chemotaxis. Proc Natl Acad Sci U S A 2014;111:5813-8. [Crossref] [PubMed]
- Mosca T, Menezes MC, Silva AV, et al. Chemotactic and Phagocytic Activity of Blood Neutrophils in Allergic Asthma. Immunol Invest 2015;44:509-20. [Crossref] [PubMed]
- Dong H, Wang T, Wang M, et al. The role of inducible costimulatory molecular ligand (ICOSL) in children with neutrophilic asthma. Transl Pediatr 2020;9:469-79. [Crossref] [PubMed]
- Deng F, Dong H, Zou M, et al. Polarization of neutrophils from patients with asthma, chronic obstructive pulmonary disease and asthma-chronic obstructive pulmonary disease overlap syndrome. Zhonghua Yi Xue Za Zhi 2014;94:3796-800. [PubMed]
- Lakschevitz FS, Visser MB, Sun C, et al. Neutrophil transcriptional profile changes during transit from bone marrow to sites of inflammation. Cell Mol Immunol 2015;12:53-65. [Crossref] [PubMed]
- Fridlender ZG, Sun J, Kim S, et al. Polarization of tumor-associated neutrophil phenotype by TGF-beta: "N1" versus "N2" TAN. Cancer Cell 2009;16:183-94. [Crossref] [PubMed]
- Sun B, Zhu L, Tao Y, et al. Characterization and allergic role of IL-33-induced neutrophil polarization. Cell Mol Immunol 2018;15:782-93. [Crossref] [PubMed]
- Filippi MD. Neutrophil transendothelial migration: updates and new perspectives. Blood 2019;133:2149-58. [Crossref] [PubMed]
- Sanz MJ, Kubes P. Neutrophil-active chemokines in in vivo imaging of neutrophil trafficking. Eur J Immunol 2012;42:278-83. [Crossref] [PubMed]
- Petri B, Sanz MJ. Neutrophil chemotaxis. Cell Tissue Res 2018;371:425-36. [Crossref] [PubMed]
- Majumdar R, Tavakoli Tameh A, Arya SB, et al. Exosomes mediate LTB4 release during neutrophil chemotaxis. PLoS Biol 2021;19:e3001271. [Crossref] [PubMed]
- Hind LE, Vincent WJ, Huttenlocher A. Leading from the Back: The Role of the Uropod in Neutrophil Polarization and Migration. Dev Cell 2016;38:161-9. [Crossref] [PubMed]
- Li Q, Li N, Liu CY, et al. Ezrin/Exocyst complex regulates mucin 5AC secretion induced by neutrophil elastase in human airway epithelial cells. Cell Physiol Biochem 2015;35:326-38. [Crossref] [PubMed]
- Pestonjamasp K, Amieva MR, Strassel CP, et al. Moesin, ezrin, and p205 are actin-binding proteins associated with neutrophil plasma membranes. Mol Biol Cell 1995;6:247-59. [Crossref] [PubMed]
- Matsumoto M, Hirata T. Moesin regulates neutrophil rolling velocity in vivo. Cell Immunol 2016;304-305:59-62. [Crossref] [PubMed]
- Jia M, Yan X, Jiang X, et al. Ezrin, a Membrane Cytoskeleton Cross-Linker Protein, as a Marker of Epithelial Damage in Asthma. Am J Respir Crit Care Med 2019;199:496-507. [Crossref] [PubMed]
- De Giovanni M, Tam H, Valet C, et al. GPR35 promotes neutrophil recruitment in response to serotonin metabolite 5-HIAA. Cell 2022;185:815-830.e19. [Crossref] [PubMed]
- Rocha-Gregg B, Huttenlocher A. Signal integration in forward and reverse neutrophil migration: Fundamentals and emerging mechanisms. Curr Opin Cell Biol 2021;72:124-30. [Crossref] [PubMed]
- Tan SY, Weninger W. Neutrophil migration in inflammation: intercellular signal relay and crosstalk. Curr Opin Immunol 2017;44:34-42. [Crossref] [PubMed]
- Ahmad M, Narayanasamy S, Ong HL, et al. STIM Proteins and Regulation of SOCE in ER-PM Junctions. Biomolecules 2022;12:1152. [Crossref] [PubMed]
- Courjaret R, Machaca K. Native SOCE complexes: Small but mighty? Cell Calcium 2021; Epub ahead of print. [Crossref] [PubMed]
- Conte E, Imbrici P, Mantuano P, et al. Alteration of STIM1/Orai1-Mediated SOCE in Skeletal Muscle: Impact in Genetic Muscle Diseases and Beyond. Cells 2021;10:2722. [Crossref] [PubMed]
- Hong J, Bao Y, Chen A, et al. Chinese guidelines for childhood asthma 2016: Major updates, recommendations and key regional data. J Asthma 2018;55:1138-46. [Crossref] [PubMed]
- Camoretti-Mercado B, Lockey RF. Airway smooth muscle pathophysiology in asthma. J Allergy Clin Immunol 2021;147:1983-95. [Crossref] [PubMed]
- Sutovska M, Kocmalova M, Franova S, et al. Pharmacodynamic evaluation of RP3128, a novel and potent CRAC channel inhibitor in guinea pig models of allergic asthma. Eur J Pharmacol 2016;772:62-70. [Crossref] [PubMed]
- Wrennall JA, Ahmad S, Worthington EN, et al. A SPLUNC1 Peptidomimetic Inhibits Orai1 and Reduces Inflammation in a Murine Allergic Asthma Model. Am J Respir Cell Mol Biol 2022;66:271-82. [Crossref] [PubMed]
- Singh D, Agusti A, Anzueto A, et al. Global Strategy for the Diagnosis, Management, and Prevention of Chronic Obstructive Lung Disease: the GOLD science committee report 2019. Eur Respir J 2019;53:1900164. [Crossref] [PubMed]
- Kremserova S, Nauseef WM. Isolation of Human Neutrophils from Venous Blood. Methods Mol Biol 2020;2087:33-42. [Crossref] [PubMed]
- Keller HU, Zimmermann A. Shape, movement and function of neutrophil granulocytes. Biomed Pharmacother 1987;41:285-9. [PubMed]
- Schaff UY, Dixit N, Procyk E, et al. Orai1 regulates intracellular calcium, arrest, and shape polarization during neutrophil recruitment in shear flow. Blood 2010;115:657-66. [Crossref] [PubMed]
- Heit B, Liu L, Colarusso P, et al. PI3K accelerates, but is not required for, neutrophil chemotaxis to fMLP. J Cell Sci 2008;121:205-14. [Crossref] [PubMed]
- Vázquez-Prieto MLÁ, Lascurais-Santamaría N, Fernández-Eufrasio NB, et al. Sex-dependent effect of aging on calcium signaling and expression of TRPM2 and CRAC channels in human neutrophils. Hum Immunol 2022;83:645-55. [Crossref] [PubMed]
- Fulop T, Larbi A, Douziech N, et al. Signal transduction and functional changes in neutrophils with aging. Aging Cell 2004;3:217-26. [Crossref] [PubMed]
- Scotland RS, Stables MJ, Madalli S, et al. Sex differences in resident immune cell phenotype underlie more efficient acute inflammatory responses in female mice. Blood 2011;118:5918-27. [Crossref] [PubMed]
- Bedouhene S, Liu M, Senani N, et al. Prolyl-Isomerase Pin1 Controls Key fMLP-Induced Neutrophil Functions. Biomedicines 2021;9:1130. [Crossref] [PubMed]
- Yadav SK, Stojkov D, Feigelson SW, et al. Chemokine-triggered microtubule polymerization promotes neutrophil chemotaxis and invasion but not transendothelial migration. J Leukoc Biol 2019;105:755-66. [Crossref] [PubMed]
- Hirata T, Nomachi A, Tohya K, et al. Moesin-deficient mice reveal a non-redundant role for moesin in lymphocyte homeostasis. Int Immunol 2012;24:705-17. [Crossref] [PubMed]
- Shibukawa Y, Suzuki T. Ca2+ signaling mediated by IP3-dependent Ca2+ releasing and store-operated Ca2+ channels in rat odontoblasts. J Bone Miner Res 2003;18:30-8. [Crossref] [PubMed]
- Liu X, O'Connell A, Ambudkar IS. Ca2+-dependent inactivation of a store-operated Ca2+ current in human submandibular gland cells. Role of a staurosporine-sensitive protein kinase and the intracellular Ca2+ pump. J Biol Chem 1998;273:33295-304. [Crossref] [PubMed]
- Wenzel SE. Asthma phenotypes: the evolution from clinical to molecular approaches. Nat Med 2012;18:716-25. [Crossref] [PubMed]
- Schleich FN, Manise M, Sele J, et al. Distribution of sputum cellular phenotype in a large asthma cohort: predicting factors for eosinophilic vs neutrophilic inflammation. BMC Pulm Med 2013;13:11. [Crossref] [PubMed]
- Simpson JL, Scott R, Boyle MJ, et al. Inflammatory subtypes in asthma: assessment and identification using induced sputum. Respirology 2006;11:54-61. [Crossref] [PubMed]
- Wenzel SE. Asthma: defining of the persistent adult phenotypes. Lancet 2006;368:804-13. [Crossref] [PubMed]
- Radermecker C, Louis R, Bureau F, et al. Role of neutrophils in allergic asthma. Curr Opin Immunol 2018;54:28-34. [Crossref] [PubMed]
- Ellett F, Marand AL, Irimia D. Multifactorial assessment of neutrophil chemotaxis efficiency from a drop of blood. J Leukoc Biol 2022;111:1175-84. [Crossref] [PubMed]
- Ray A, Kolls JK. Neutrophilic Inflammation in Asthma and Association with Disease Severity. Trends Immunol 2017;38:942-54. [Crossref] [PubMed]
- Matsushima H, Geng S, Lu R, et al. Neutrophil differentiation into a unique hybrid population exhibiting dual phenotype and functionality of neutrophils and dendritic cells. Blood 2013;121:1677-89. [Crossref] [PubMed]
- Matsushima K, Yang D, Oppenheim JJ. Interleukin-8: An evolving chemokine. Cytokine 2022;153:155828. [Crossref] [PubMed]
- Fehon RG, McClatchey AI, Bretscher A. Organizing the cell cortex: the role of ERM proteins. Nat Rev Mol Cell Biol 2010;11:276-87. [Crossref] [PubMed]
- García-Ortiz A, Serrador JM. ERM Proteins at the Crossroad of Leukocyte Polarization, Migration and Intercellular Adhesion. Int J Mol Sci 2020;21:1502. [Crossref] [PubMed]
- Liu X, Yang T, Suzuki K, et al. Moesin and myosin phosphatase confine neutrophil orientation in a chemotactic gradient. J Exp Med 2015;212:267-80. [Crossref] [PubMed]
- Chen F, Yu M, Zhong Y, et al. The role of neutrophils in asthma. Zhejiang Da Xue Xue Bao Yi Xue Ban 2021;50:123-30. [Crossref] [PubMed]
- Blatter LA. Tissue Specificity: SOCE: Implications for Ca(2+) Handling in Endothelial Cells. Adv Exp Med Biol 2017;993:343-61. [Crossref] [PubMed]
- Ambudkar IS, de Souza LB, Ong HL. TRPC1, Orai1, and STIM1 in SOCE: Friends in tight spaces. Cell Calcium 2017;63:33-9. [Crossref] [PubMed]
- Servant G, Weiner OD, Herzmark P, et al. Polarization of chemoattractant receptor signaling during neutrophil chemotaxis. Science 2000;287:1037-40. [Crossref] [PubMed]
(English Language Editor: J. Gray)