Agitated saline with 10% blood increases number and stability of microbubbles in detection right-to-left shunt by contrast-enhanced transcranial Doppler: an in vitro and in vivo observational study
Highlight box
Key findings
• Agitated saline (AS) with 10% blood (10% BAS) produced a great number of and stable microbubbles, which significantly increased the efficacy of contrast-enhanced transcranial Doppler (c-TCD) examinations in detecting large right-to-left shunt (RLS).
What is known and what is new?
• Previous research has shown that adding blood improved the performance of AS in diagnosing RLS with c-TCD.
• Blood volume in AS may affect the characteristics of the microbubbles and lead to variations in c-TCD results.
What is the implication, and what should change now?
• 10% BAS should be applied more in c-TCD procedures to improve the efficacy and consistency of the RLS results.
Introduction
The patent foramen ovale (PFO) is one of the most common anatomic variants and is found in nearly 30% of the general population (1). The occasional opening of the PFO results in an abnormal right-to-left shunt (RLS). A thrombus and tumor straddling the PFO provide direct evidence that the RLS-related paradoxical embolism is correlated with cryptogenic stroke, migraine, and some of other symptoms (2,3). Given the increasingly understanding of PFO closure for stroke prevention, current guidelines recommend PFO screenings for stroke patients as necessary (4,5).
A PFO screening relies on morphological findings and a functional assessment. Transesophageal echocardiography (TEE) provides clear morphological images and is considered the gold standard for diagnosing PFO. However, due to the discomfort of patients during the procedure and difficulties related to patients agreeing to cooperate in further examinations, TEE is the last option for PFO screening (6). Contrast-enhanced transcranial Doppler (c-TCD) has been widely used for RLS screening because of its high tolerance and safety. Microbubbles produced by contrast agents go to the brain vessels and are semi-quantified by c-TCD; the higher the RLS level, the stronger the correlation between the PFO and symptoms (7).
Previous studies have shown that adding blood significantly improves the performance of agitated saline (AS) in diagnosing RLS; however, controversy remains as to the optimal amount of blood, from one drop to 10%, in the AS, and the mechanism for improving c-TCD performance is unclear. Gentile et al. showed 1 mL blood in AS significantly improved RLS results (8). Differently, Hao and colleagues demonstrated the superiority of adding one drop of blood to AS in detecting RLS (9). No matter how much of blood was added, the mechanism for improving c-TCD performance might come from the enhancement by red blood cells or more bubbles (10,11). In this study, we compared the characterization of microbubbles induced by AS without blood, AS with 5% blood (5% BAS), and AS with 10% blood (10% BAS), which were common agents applied in clinical practice, in vitro and compared the positive rate and RLS classification by c-TCD in patients using 3 contrast agents to investigate the correlation between the characterization of AS with blood and the clinical performance of c-TCD. We present the following article in accordance with the MDAR reporting checklist (available at https://jtd.amegroups.com/article/view/10.21037/jtd-23-284/rc).
Methods
In vitro analysis of contrast-induced microbubbles
The number, size, and stability of the microbubbles induced by different contrast agents were recorded and compared under a microscope. The contrast agents were prepared as follows: AS without blood, containing 1 mL of air and 9 mL of saline; 5% BAS, containing 1 mL of air, 0.5 mL of whole blood, and 8.5 mL of saline; and 10% BAS, containing 1 mL of air, 1 mL of whole blood, and 8 mL of saline. The agent was agitated by mixing 10 times through 2×10 mL syringes connected with a 3-way stopcock. One drop of mixture was placed on a slide and observed at 40× magnification under a microscope. The observations of each agent were repeated 10 times. The microbubble number was calculated from the average of 3 fields at each observation. The microbubble size was measured according to the scale bar. Microbubble stability was compared by numbers and sizes at 5 min and 10 min post-agitation.
In vivo analysis of the detection of RLS by c-TCD
Patients at the Congenital Heart Disease Center, Wuhan Asia Heart Hospital, who were suspected to have RLS and were aged >18 years, were continuously recruited for this study from May to July 2021. Patients were excluded from the study if they met any of the following exclusion criteria: (I) had a vascular puncture difficulty; (II) had multiple organ failure; and/or (III) were unable to cooperate. The study was conducted in accordance with the Declaration of Helsinki (as revised in 2013). The study protocol was reviewed and approved by the Ethics Committee of Wuhan Asia Heart Hospital (No. 2021-016). Informed written consent was obtained from all the patients while recruiting.
The c-TCD examination was performed by 2 senior technicians with a transcranial Doppler machine (Delica Medical Equipment, Shenzhen, China) using a probe at 1.6 MHz. The patient underwent the examination in the supine position. An 18-gauge needle (Becton Dickinson, Franklin Lakes, NJ, USA) was inserted into the cubital vein to inject the contrast agent. The left middle cerebral artery was isolated for cranial signal detection. All the patients underwent examinations with 3 contrast agents. The examination started randomly, and the tests using the different contrast agents were performed with 15-min breaks. The 3 agents were prepared and mixed as described above before the examination. The contrast agent (10 mL) was injected rapidly after being well prepared. The examinations were repeated at rest and with the Valsalva maneuver. The patients performed the Valsalva maneuver using a breathing assistant instrument to ensure better cooperation. The microbubble signal was determined by a Doppler flow velocity curve during the examination. The number of microbubbles was detected by TCD within 25 s of the injection and recorded for later analysis. The detection time was defined as that from the contrast injection to the first signal and was also recorded.
The level of RLS was classified according to the current guidelines (12) as follows: Level 0: no signal detected, no RLS; level I: 1–20 microbubbles, mild RLS; level II: 30–50 microbubbles, moderate RLS; and level III: >50 microbubbles, large RLS.
Statistical analysis
The data are presented as the mean ± standard deviation for the normally distributed continuous data. The descriptive data are presented as the count and percentage. A 1-way analysis of variance was applied to compare the microbubble number among the 3 contrast agents, followed by a least significant difference (LSD) or Tamhane’s T2 test according to the variance. The differences in detection times among the 3 agents were compared using a paired-sample t test. The categorical variable as RLS classification were compared by Crosstabs with the χ2 test. A two-sided P value <0.05 was considered statistically significant. All the statistical analyses were performed using SPSS 21.0 (IBM, Armonk, NY, USA).
Results
In vitro characterizations of AS with different blood amounts
Images of different agent-induced microbubbles under microscopy are shown in Figure 1. The numbers of microbubbles differed significantly among the contrast agents (F=62.38, P<0.001). 10% BAS produced 43.9±12.7/field microbubbles immediately after agitation, which was much more than that of the AS without blood (5.4±2.4/field, P<0.001) and the 5% BAS (30.4±4.2/field, P=0.025). A great number of microbubbles in the AS without blood group were observed when injecting the mixture on the slide; however, these microbubbles disappeared very quickly and before capture. Differences in the numbers between the 5% BAS and 10% BAS were the greatest at 5 minutes post-agitation and remained at 10 minutes post-agitation. Nearly 70% of the microbubbles (30.7±6.9/field) remained in the 10% BAS 5 min post-agitation, and half of the microbubbles (15.4±4.4/field) from the 5% BAS remained under microscopy (Figure 2). At 10 min post-agitation, an 18.5±6.1/field could be observed in the 10% BAS, which was still more than that in the 5% BAS (7.1±2.0/field, P<0.001). The microbubbles induced by the 10% BAS were larger than those induced by the 5% BAS immediately post-agitation (13.6±6.3 vs. 9.2±8.2 µm, P<0.001). The small microbubbles were eliminated faster in the 5% BAS group, which resulted in a size increase from 5 to 10 min post-agitation (17.3±10.7 vs. 22.1±10.6 µm, P=0.018). The size of the microbubbles in the 10% BAS group did not change significantly from 5 to 10 min post-agitation (14.5±5.3 vs. 12.6±6.4 µm, P=0.276).

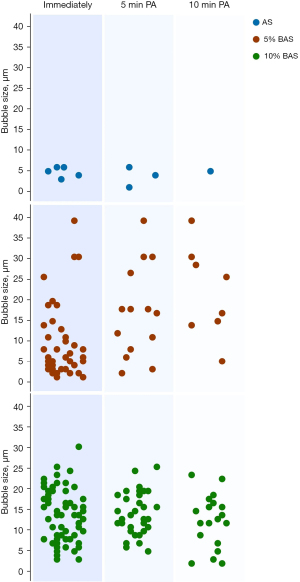
In vivo results of c-TCD using AS with different blood amounts
A total of 74 patients were included in the study. No adverse events occurred during or after the study. The clinical characteristics of the patients are shown in Table 1. The c-TCD results were inconsistent when different contrast agents were used in the same patient (Figure 3). The microbubble detection time using the AS without blood (4.0±1.5 s) was longer than that of either the 5% BAS (1.1±0.7 s, P<0.0001) or the 10% BAS (1.0±0.8, P<0.0001). Table 2 sets out the RLS classification by c-TCD using different contrast agents. RLS was found in 47 (63.5%) patients using the AS without blood, 50 (67.6%) using the 5% BAS, and 53 (71.6%) using the 10% BAS. Differences in the overall positive rate among the 3 contrast agents did not reach statistical significance. However, the AS with blood had more moderate to large RLS than the AS without blood. There were 35 (47.3%) patients with RLS levels II and III in the c-TCD using the 5% BAS, and 38 (51.4%) using the 10% BAS, which was significantly more than that using the AS without blood (17, 23.0%, P=0.001). Moreover, the RLS classification when using the 10% BAS yielded the highest rate of RLS level III compared to the other 2 contrast agents (Table 2).
Table 1
Clinical characteristics | N (%) |
---|---|
Sex (male/female) | 30 (40.5)/44 (59.5) |
Age (years), mean ± SD | 39.9±16.1 |
Symptom | |
Ischemic stroke | 30 (40.5) |
TIA | 29 (39.2) |
Dizziness | 11 (14.9) |
Migraine | 39 (52.7) |
Syncope | 3 (4.1) |
Aphasia | 1 (1.4) |
SD, standard deviation; TIA, transient ischemic attack.
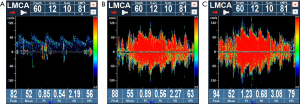
Table 2
Agent | Level 0 | Level I | Level II | Level III |
---|---|---|---|---|
AS | 27 (36.5%) | 30 (40.5%) | 8 (10.8%) | 9 (12.2%) |
5% BAS | 24 (32.4%) | 15 (20.3%) | 16 (21.6%) | 19 (25.7%) |
10% BAS | 21 (28.4%) | 15 (20.3%) | 12 (16.2%) | 26 (35.1%) |
χ2 | 1.110 | 10.278 | 3.181 | 10.718 |
P | 0.574 | 0.006 | 0.204 | 0.005 |
RLS, right-to-left shunt; c-TCD, contrast-enhanced transcranial Doppler; AS, agitated saline without blood; 5% BAS, agitated saline with 5% blood;10% BAS, agitated saline with 10% blood.
Discussion
Our in vitro study demonstrates that the BAS significantly increased the number of microbubbles. The microbubbles in the BAS were more stable than those in the AS. Moreover, the number was the highest and the stability of the microbubbles was the best in the 10% BAS. Similarly, our in vivo study showed that the BAS contrast agents significantly increased the positive rate and the level of RLS detected by c-TCD in patients. The use of 10% BAS in the c-TCD further increased the positive rate and RLS level more than the 5% BAS; however, the difference was not statistically significant.
Contrast agents containing gas bubbles have been used to expand the diagnostic yield of ultrasonography for many years. The gas bubbles not only trace the tissue profile for differential diagnosis, but also increase the blood flow signal used to assess flow direction and velocity (13). Importantly, the size and stability of gas bubbles affects the diagnosis rate (14). Studies have shown that adding blood to AS significantly improves the performance of contrast agents (11). Jeon et al. compared the characteristics of microbubbles of different contrast agents and found that the number of microbubbles was significantly more in the 10% air–10% blood-saline mixture than in the 10% air-90% saline, which resulted in higher accuracy in the measurement of pulmonary artery systolic pressure (15).
Similarly, our study showed that adding blood significantly increased the microbubble number. The number of microbubbles increased as the blood amount increased. We observed that a large amount of microbubbles in the AS without blood could be observed under the microscope; however, they disappeared too quickly for capture. The AS without blood had the lowest recorded number, as the microbubbles were very unstable. This might be one reason why AS had the lowest diagnosis rate when it was used in c-TCD in later investigations. Our study also showed that both the size and number of microbubbles were most stable in the 10% BAS, which could be the reasons why the 10% BAS had the highest positive rate and RLS levels in c-TCD.
PFO diagnosis is recommended in current stroke prevention and management guidelines (4). c-TCD is becoming more important for RLS screenings and diagnoses because of its good tolerance and good safety profile. Yang observed a similar detection rate between c-TCD and c-TEE (16). With a sensitivity of 80%, c-TCD had a higher diagnostic rate and a lower misdiagnosis rate and negative likelihood ratio than c-TTE when applied to PFO detection (17). Unlike c-TTE, c-TCD positive was correlated with a pathogenic PFO that produced moderate to large RLS (18). c-TCD has been recommended for the first screening of PFO when considering cardiogenic RLS (12); however, the results relied on the operator and standard protocol, which is still being debated.
Studies have reported variations in the RLS diagnosis rate when using different contrast agents (19,20). Compared to commercial contrasts and AS without blood, the addition of blood increases the sensitivity of c-TCD to as high as 100% (10). Further, the blood amount in the AS is controversial given clinical safety and practices. Gentile noted that adding 1 mL of a patient’s blood significantly increased the number of microbubbles, and led to a higher positive rate and RLS levels in patients (8). Conversely, Hao et al. reported that adding 1 drop of blood significantly improved RLS diagnosis of c-TCD by producing more microbubbles (9). Our study showed similar results; that is, the RLS levels in c-TCD differed when different contrast agents were used. The detection time was much shorter when the BAS was used than when the AS without blood was used. The use of the BAS led to higher RLS levels than the use of the AS without blood. There was a trend of more positive and higher RLS levels when using the 10% BAS; however, the differences between the 5% BAS and 10% BAS were not statistically significant. We tried to include AS with 1 drop of blood in our study; however, inconsistences between the replications led to the exclusion of the data from further analysis.
Our study included an in vitro analysis and in vivo observations to demonstrate the effectiveness of using BAS for c-TCD; however, our study had a number of limitations. Firstly and most importantly, the number of cases included in our study was small, which might lead to the results of no statistically significant differences among three agents. Secondly, we only studied three amounts of blood in AS, which were none, 5%, and 10% based on previous reports and clinical experiences. Other amounts of blood in AS may further address the difference and significance. Thirdly, unlike in previous studies, we directly observed the microbubbles on slide without covering the slide, which might have resulted in the movement and elimination of microbubbles.
Conclusions
In conclusion, we showed that adding blood to AS significantly improved c-TCD performance by increasing the number and stability of microbubbles when detecting RLS. The use of 10% BAS will improve the diagnosis of PFO.
Acknowledgments
We would like to thank Bowen Jin, Qiu Qiu, Qin Li, and Xin Yu (All of them come from Congenital Heart Disease Center, Wuhan Asia Heart Hospital, Wuhan, China) for their help during the daily RLS screenings. We also would like to thank all our colleagues at the Congenital Heart Disease Center of Wuhan Asia Hospital and Center of Structural Heart Disease in Zhongnan Hospital of Wuhan University for their help.
Funding: The work was supported by the Scientific Research Program of Health and Family Planning Commission of Wuhan Municipality (No. WX21Z26 to QSS).
Footnote
Reporting Checklist: The authors have completed the MDAR reporting checklist. Available at https://jtd.amegroups.com/article/view/10.21037/jtd-23-284/rc
Data Sharing Statement: Available at https://jtd.amegroups.com/article/view/10.21037/jtd-23-284/dss
Peer Review File: Available at https://jtd.amegroups.com/article/view/10.21037/jtd-23-284/prf
Conflict of Interest: All authors have completed the ICMJE uniform disclosure form (available at https://jtd.amegroups.com/article/view/10.21037/jtd-23-284/coif). The authors have no conflicts of interest to declare.
Ethical Statement: The authors are accountable for all aspects of the work in ensuring that questions related to the accuracy or integrity of any part of the work are appropriately investigated and resolved. The study was conducted in accordance with the Declaration of Helsinki (as revised in 2013). The study protocol was reviewed and approved by the Ethics Committee of Wuhan Asia Heart Hospital (No. 2021-016). Informed written consent was obtained from all the patients while recruiting.
Open Access Statement: This is an Open Access article distributed in accordance with the Creative Commons Attribution-NonCommercial-NoDerivs 4.0 International License (CC BY-NC-ND 4.0), which permits the non-commercial replication and distribution of the article with the strict proviso that no changes or edits are made and the original work is properly cited (including links to both the formal publication through the relevant DOI and the license). See: https://creativecommons.org/licenses/by-nc-nd/4.0/.
References
- Hara H, Virmani R, Ladich E, et al. Patent foramen ovale: current pathology, pathophysiology, and clinical status. J Am Coll Cardiol 2005;46:1768-76. [Crossref] [PubMed]
- Shang X, Li D, Qiu Q, et al. First direct evidence of a Patent Foramen Ovale (PFO): a large thrombus straddling the foramen ovale. Eur Heart J 2016;37:782. [Crossref] [PubMed]
- Vondran M, Ghazy T, Andrási TB, et al. ST-Segment Elevation Myocardial Infarction and Right Atrial Myxoma. Thorac Cardiovasc Surg Rep 2022;11:e33-7. [Crossref] [PubMed]
- Horlick E, Kavinsky CJ, Amin Z, et al. SCAI expert consensus statement on operator and institutional requirements for PFO closure for secondary prevention of paradoxical embolic stroke: The American Academy of Neurology affirms the value of this statement as an educational tool for neurologists. Catheter Cardiovasc Interv 2019;93:859-74. [Crossref] [PubMed]
- Kleindorfer DO, Towfighi A, Chaturvedi S, et al. 2021 Guideline for the Prevention of Stroke in Patients With Stroke and Transient Ischemic Attack: A Guideline From the American Heart Association/American Stroke Association. Stroke 2021;52:e364-467. [Crossref] [PubMed]
- Mojadidi MK, Roberts SC, Winoker JS, et al. Accuracy of transcranial Doppler for the diagnosis of intracardiac right-to-left shunt: a bivariate meta-analysis of prospective studies. JACC Cardiovasc Imaging 2014;7:236-50. [Crossref] [PubMed]
- Chino S, Mochizuki Y, Mizuma K, et al. Transcranial Doppler for stratification of high-risk morphology of patent foramen ovale in patients with cryptogenic stroke. Heart Vessels 2022;37:2119-27. [Crossref] [PubMed]
- Gentile M, De Vito A, Azzini C, et al. Adding blood to agitated saline significantly improves detection of right-to-left shunt by contrast-transcranial color-coded duplex sonography. Ultrasound Med Biol 2014;40:2637-41. [Crossref] [PubMed]
- Hao N, Liu K, Guo ZN, et al. Comparison of two contrast agents for right-to-left shunt diagnosis with contrast-enhanced transcranial Doppler. Ultrasound Med Biol 2014;40:2317-20. [Crossref] [PubMed]
- Mojadidi MK, Zhang L, Chugh Y, et al. Transcranial Doppler: Does Addition of Blood to Agitated Saline Affect Sensitivity for Detecting Cardiac Right-to-Left Shunt? Echocardiography 2016;33:1219-27. [Crossref] [PubMed]
- Jeon DS, Luo H, Iwami T, et al. The usefulness of a 10% air-10% blood-80% saline mixture for contrast echocardiography: Doppler measurement of pulmonary artery systolic pressure. J Am Coll Cardiol 2002;39:124-9. [Crossref] [PubMed]
- Zetola VF, Lange MC, Scavasine VC, et al. Latin American Consensus Statement for the Use of Contrast-Enhanced Transcranial Ultrasound as a Diagnostic Test for Detection of Right-to-Left Shunt. Cerebrovasc Dis 2019;48:99-108. [Crossref] [PubMed]
- Wilson SR, Burns PN. Microbubble contrast for radiological imaging: 2. Applications. Ultrasound Q 2006;22:15-8. [PubMed]
- Burns PN. Overview of echo-enhanced vascular ultrasound imaging for clinical diagnosis in neurosonology. J Neuroimaging 1997;7:S2-14. [PubMed]
- Jeon DS, Miyamoto T, Fontana G, et al. Pulmonary artery compression by a saphenous vein graft aneurysm and contrast echocardiography using an agitated mixture of ten percent air, ten percent blood, and ten percent saline. J Am Soc Echocardiogr 2002;15:1529-32. [Crossref] [PubMed]
- Yang J, Zhang H, Wang Y, et al. The Efficacy of Contrast Transthoracic Echocardiography and Contrast Transcranial Doppler for the Detection of Patent Foramen Ovale Related to Cryptogenic Stroke. Biomed Res Int 2020;2020:1513409. [Crossref] [PubMed]
- Yang X, Wang H, Wei Y, et al. Diagnosis of Patent Foramen Ovale: The Combination of Contrast Transcranial Doppler, Contrast Transthoracic Echocardiography, and Contrast Transesophageal Echocardiography. Biomed Res Int 2020;2020:8701759. [Crossref] [PubMed]
- Chen J, Chen L, Hu W, et al. A comparison of contrast transthoracic echocardiography and contrast transcranial Doppler in cryptogenic stroke patients with patent foramen ovale. Brain Behav 2019;9:e01283. [Crossref] [PubMed]
- Puledda F, Toscano M, Pieroni A, et al. Right-to-left shunt detection sensitivity with air-saline and air-succinil gelatin transcranial Doppler. Int J Stroke 2016;11:229-38. [Crossref] [PubMed]
- Droste DW, Kriete JU, Stypmann J, et al. Contrast transcranial Doppler ultrasound in the detection of right-to-left shunts: comparison of different procedures and different contrast agents. Stroke 1999;30:1827-32. [Crossref] [PubMed]
(English Language Editor: L. Huleatt)