Preventing atelectasis during bronchoscopy under general anesthesia
Introduction
Atelectasis is a prominent and well-defined phenomenon in patients undergoing surgery under general anesthesia with mechanical ventilation, initially described in the anesthesiology literature nearly 60 years ago (1,2). Since then, perioperative atelectasis has been studied widely with multiple studies focusing on pulmonary physiology, the effects of mechanical ventilation and muscle relaxation, and clinical outcomes (3-6). However, this phenomenon and its relevance was not recognized in the field of bronchoscopy until very recently (7).
The diagnosis of peripheral lung nodules using navigational bronchoscopy has made tremendous advances in the last decade, aided by state-of-the-art technology, including electromagnetic navigation and shape sensing platforms, robotic bronchoscopy, and the use of intraprocedural three-dimensional images using cone beam computed tomography (CBCT) (8-13). However, this form of bronchoscopy generally requires mechanical ventilation with muscular relaxation in order to reduce lung motion during the procedure, and is therefore not immune to intraoperative atelectasis (14). While atelectasis is of concern in the surgical population because of its association with postoperative pulmonary complications such as hypoxemia, pneumonia and ventilator induced lung injury, intraoperative atelectasis poses a more immediate real-time challenge to the bronchoscopist: obscuring the target lesion, creating computed tomography (CT) to body divergence, or mimicking lung tumors (7,15,16). Hence the need to further understand and prevent this phenomenon. In this review, we will describe the pathophysiology, incidence, clinical impact, and preventive strategies for atelectasis occurring during bronchoscopy under general anesthesia. The incidence and relevance of atelectasis during bronchoscopy under moderate sedation is not known and needs to be studied.
Physiological basis of atelectasis
Physiologically, atelectasis occurs when collapsing forces acting upon terminal lung units (bronchioles and alveoli) overcome expanding forces. Several factors, mechanistically and biochemically, are at play when patients undergo mechanical ventilation and general anesthesia that contribute not only to the development of atelectasis, but also to the clinical outcomes related to atelectasis (17).
Under normal physiologic circumstances, inward-acting (collapsing) forces and outward-acting (expanding) forces are at equilibrium, allowing terminal bronchioles and alveoli to remain open and contribute to gas exchange. Increasing positive pleural pressures and surface tension act as collapsing forces on terminal bronchioles and alveoli, whereas tethering forces, derived from attachments of bronchioles and alveolar walls to adjacent structures, and surfactant, a lipoprotein secreted by type II alveolar epithelial cells, act as outward forces to keep these lung units open (18). However, general anesthesia and mechanical ventilation increase the tendency toward atelectasis through various processes that increase pleural pressure, decrease alveolar pressures, and result in surfactant impairment.
One mechanism for increase in pleural pressure during general anesthesia is the reduction and loss of diaphragmatic tone. Under awake and supine conditions, the diaphragm reduces transmission of abdominal pressure to the lungs (19), and its dome shape allows for more lung expansion in dependent areas than non-dependent locations. With general anesthesia, both of these functions become impaired, and thus, increase the likelihood of developing atelectasis. Furthermore, relaxation and paralysis of accessory muscles needed for respiration result in decreased lung aeration during anesthesia. An additional cause for increased pleural pressures during general anesthesia is from compression of dependent lung by the weight of the non-dependent lung and other mediastinal structures when patients are supine for extended periods of time.
The minimum pressures needed to open terminal lung units in dependent regions of the lung are higher than those in non-dependent regions. However, the ideal positive end-expiratory pressure (PEEP) needed to keep alveolar pressures above closing pressures during expiration is not always known, so a decrease in alveolar pressures is common during general anesthesia. Also, the transmission of upper airway pressures to distal lung regions can be compromised during general anesthesia and mechanical ventilation. Obstructive atelectasis develops when airway obstruction, most commonly from retained secretions or mucous plugging, decreases transmission of pressures to distal airways. Some anesthetics are also known to have a higher tendency to cause ciliary dysmotility (20), and decreased mucociliary clearance is more common with a cuffed endotracheal tube than laryngeal mask airway (21), both of which contribute to obstructive atelectasis.
Several factors also impair surfactant function during general anesthesia and contribute to atelectasis. Mechanical ventilation can cause overdistention and stretching of alveoli that results in decreased surfactant production, and inflammation within the lung also results in reduced production of surfactant (22-24). Ultimately, atelectasis during general anesthesia in dependent and caudal lung regions has been detected in up to 90% of patients (25), with up to 20% to 25% of normal lung noted to be either poorly aerated or atelectatic on computed tomography during anesthesia (26).
In addition to the above described changes that derive from general anesthesia and promote atelectasis, several pro-atelectatic maneuvers occur during bronchoscopy. The specific pathophysiology of atelectasis during bronchoscopy has not been studied. However, it is not unreasonable to think that the presence of a bronchoscope obstructing the endotracheal tube and the airways, the use of suction, the potential bleeding/clotting with biopsies, and the lack of a tight seal in the anesthesia circuit can all result in decreased alveolar pressures, that will in turn promote atelectasis.
Incidence of atelectasis during bronchoscopy
In order to investigate the incidence, anatomic location and risk factors for developing atelectasis during bronchoscopy under general anesthesia, Sagar et al. designed the I-LOCATE trial (Incidence and Location of Atelectasis) (16). This was a prospective observational study, in which patients undergoing peripheral bronchoscopy were subject to an atelectasis survey carried out by radial-probe ultrasound (RP-EBUS) under fluoroscopic guidance. The primary objective was to estimate the portion of patients identified as developing intraprocedural atelectasis, while secondary endpoints investigated the location and number of atelectatic segments per patient. The authors performed a systematic evaluation of 8 study target segments in dependent areas of the lungs: RB2, RB6, RB9, RB10, LB2, LB6, LB9, and LB10. For each segment, two subsegments (a and b) were evaluated. RP-EBUS images were categorized as either aerated lung (“snowstorm” pattern), or as having a nonaerated or atelectatic pattern. Categorization was performed by 3 independent readers, and intraclass correlation agreement was 0.82 (95% CI: 0.79–0.89). Fifty-seven patients were included in the study with 89% being found to have atelectasis in at least one of the eight evaluated segments, 79% having atelectasis in at least three segments, 72% with atelectasis in at least four segments, 58% with atelectasis in at least five segments and 32% having atelectasis in at least six of the eight evaluated segments. The number of segments with atelectasis per patient increased with higher body mass index (BMI) and increased time to the atelectasis survey (and therefore longer time under general anesthesia-with a median time to atelectasis survey of 33 minutes). As had been noted in the earlier study, atelectasis was once again not evident by fluoroscopy in any of the patients. Their finding of an incidence of greater than 50% of atelectasis in all dependent lower segments after 30 minutes of anesthesia highlighted a significant obstacle to performing successful bronchoscopy for peripheral lung lesions and called for an immediate need to develop preventive measures to avoid atelectasis.
Clinical impact of atelectasis during bronchoscopy
Navigational and diagnostic yield
Diagnostic yield in peripheral bronchoscopy is influenced, from a technical perspective, at three distinct levels or phases (27). These are “navigation”, “confirmation”, and “acquisition”. “Navigation”, defined as the ability to reach the target lesion, is influenced by many factors including target lesion related factors (size, location etc.), operators’ ability, and the type of navigational tools and technology used. “Confirmation” could be defined as the ability to demonstrate with images that navigation or sampling tools are in contact with a target. Finally, “acquisition” is the ability to obtain diagnostic samples. While there are no prospective studies in the literature designed to specifically analyze the influence of atelectasis on the diagnostic yield of bronchoscopy, it is clear from the available data that atelectasis can negatively impact the three phases of peripheral bronchoscopy described above. The actual extent of this negative impact is not known, but it is obvious that the effect will be greater for patients with lesions located in dependent areas of the lung, and patients at higher risk for atelectasis (higher BMI, longer procedures).
The initial phase of “navigation” can be particularly affected when electromagnetic or shape sensing navigational technologies are utilized. This can be explained by the phenomenon denominated “CT-body divergence”. The latter refers to the difference in volume and shape of the lungs between static preprocedural CT reconstructions (at full inspiration) and the dynamic, breathing lung during bronchoscopy (14). Despite the registration process performed to align the preprocedural CT scan to the patient’s airways and lungs, atelectasis can “deform” the lungs and increase even further the distance between the “virtual” and the “actual” lung target (28).
“Confirmation” is the phase that is more heavily impacted by atelectasis. Until very recently, when CBCT was added to the pulmonologists’ armamentarium, RP-EBUS was the sole method utilized to confirm that a peripheral lung target was reached. In fact, RP-EBUS is still the most commonly utilized method for confirmation since CBCT is not widely available. Recent publications have demonstrated that atelectasis can easily mimic targets on RP-EBUS, creating both eccentric and concentric ultrasound images that can sometimes be indistinguishable from lung tumors (7,16). In the absence of other confirmatory techniques, these falsely positive images can lead to sampling of atelectatic parenchyma resulting in non-diagnostic tissue. Thus, atelectasis may be partly responsible for the known gap between navigational yield and diagnostic yield previously reported in studies of peripheral bronchoscopy with RP-EBUS studies (29). Even when more advanced imaging techniques such as CBCT are available and help us recognize atelectasis (and avoid biopsies of atelectatic parenchyma), if atelectasis completely obscure the target (Figure 1), it will still prevent the operator from taking samples, also resulting in a non-diagnostic bronchoscopy. A prospective observational cohort study on the use of CBCT to guide peripheral bronchoscopy performed in 2018 by Casal et al. (7)—the first to report the incidental finding of atelectasis during bronchoscopy—is a clear example of this. Eight of their 20 patients (40%) demonstrated atelectasis, and in half of these cases, the atelectasis was obscuring the actual target lesion. Despite temporary increases in PEEP and tidal volumes, there was a failure to resolve atelectasis in these patients and samples were not obtained, and a diagnosis was not made. Of note, none of the atelectasis that had developed was visible with two dimensional (2D)-fluoroscopy.
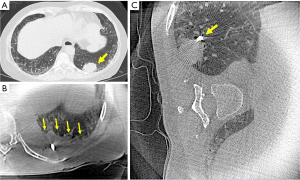
Challenges of procedural order: staging EBUS or peripheral bronchoscopy first?
The recent awareness of the high incidence of atelectasis during bronchoscopy has presented a new dilemma for bronchoscopists who see patients needing both diagnosis and staging of possible lung cancer. Bronchoscopists face a challenge in deciding whether to perform staging endobronchial ultrasound (EBUS) or peripheral bronchoscopy first, particularly when evaluating patients with lung nodules and radiologically normal lymph nodes. EBUS staging has traditionally been performed first (when on-site cytology exam is available) because if any lymph node shows malignancy, both diagnosis and nodal staging have been established, thereby avoiding an extra procedure (i.e., peripheral bronchoscopy) which also has a higher risk of complications. However, if EBUS is performed first and it is negative, and peripheral bronchoscopy needs to follow, then the inevitable issue of atelectasis will be encountered which can potentially affect the navigational and diagnostic yield of peripheral bronchoscopy, especially for lesions located in dependent areas. Given the high relevance of this dilemma, Kammer et al. conducted a Monte Carlo simulation to help determine which strategy would yield the highest number of diagnostic bronchoscopies (30). This model suggested that a peripheral bronchoscopy first approach (with a radiologically normal mediastinum) may result in more diagnostic bronchoscopies with a lesser need for additional interventions and a favorable net complication rate. Each procedure needs to be planned with a strong understanding of the pretest probability of malignant lymph nodes while appreciating the relationship of the development of intraprocedural atelectasis with increased procedure length. Fortunately, as you will see in the following sections, we may be able to prevent atelectasis long enough to perform nodal staging first and avoid unnecessary peripheral bronchoscopies when lymph nodes are involved.
Strategies to mitigate the development of intraprocedural atelectasis
Lung navigation ventilation protocol (LNVP)
Bhadra et al. retrospectively evaluated with intraprocedural CBCT a LNVP which was optimized for guided bronchoscopy in an attempt to minimize intraprocedural respiratory motion and the development of atelectasis (31). In their retrospective study, they included a total of 50 patients, each with peripheral lung lesions less than 30 mm. In the first group of 25 consecutive patients, a conventional ventilation protocol was utilized. In the second group of 25 consecutive patients a dedicated LNVP, which was developed by the authors, was used during the bronchoscopy. The authors did not utilize RP-EBUS, instead opting for CBCT to confirm the tool-in-lesion. Conventional ventilation, as defined in their study, utilized either continuous or standard intermittent mandatory ventilation. Specifically PEEP, tidal volume, fraction of inspired oxygen (FiO2), respiratory rate and airway pressure valves were not subject to any particular requirements-with FiO2 being typically set at 100% FiO2 and the PEEP at either 0 or 5 cmH2O (per the discretion of the anesthesiologist, since this was not done within a prospective research study). The authors’ dedicated LNVP utilized rapid intubation with an 8.5 or larger endotracheal tube, paralysis with nondepolarizing muscle relaxants, and lowering the FiO2 to the lowest tolerable FiO2 (Table 1). This was followed by 4 alveolar recruitment maneuvers (which were hand-delivered by bagging the patient with 30 cmH2O over 30 seconds or 40 cmH2O over 40 seconds). A dual ventilation strategy with pressure-controlled continuous mechanical ventilation and patient specific tidal volume (10–12 cc/kilogram ideal body weight) was utilized. The LNVP protocol delivered PEEP based on the location of the lesion with upper/middle lobe lesions receiving PEEP of 10–15 cmH2O and lower lobe lung lesions receiving 15–20 cmH2O. The primary endpoints of the study were dependent atelectasis, sublobar/lobar atelectasis and lesion being obscured by atelectasis (determined by CBCT during the tool-in-lesion scan). Their secondary endpoint was diagnostic yield.
Table 1
LNVP | VESPA | |
---|---|---|
Airway | Endotracheal tube | Endotracheal tube |
Mode of ventilation | Dual ventilation strategy with pressure-controlled continuous mechanical ventilation and patient specific VT | Volume control |
VT | 10–12 cc/kg of IBW | 6–8 cc/kg of IBW |
FiO2 | Lowering to the lowest tolerable FiO2 | <100% (titrated as low as possible to maintain an oxygen saturation of >94%) |
PEEP | Upper/middle lobe target:10–15 cmH2O | 8–10 cmH2O |
Lower lobe target: 15-20 cmH2O‡ | ||
Recruitment maneuver | Performed post-intubation → 4 alveolar recruitment maneuvers, hand-delivered via bagging the patient with 30 cmH2O over 30 seconds or 40 cmH2O over 40 seconds. Variability in timing and pressures based on anesthesiology personnel | Performed immediately post-intubation → 10 consecutive breaths at a plateau pressure of 40 cmH2O, with a PEEP of 20 cmH2O in pressure control mode* |
*, VESPA recruitment maneuver repeated if any accidental disconnection of the ventilatory circuit (from the ETT) occurred, or if the ETT cuff was deflated to adjust the ETT position; ‡, LNVP protocol delivered PEEP based on the location of the lesion (upper/middle vs. lower lobe lesions). LNVP, lung navigation ventilation protocol; VESPA, ventilatory strategy to prevent atelectasis; PEEP, positive end-expiratory pressure; IBW, ideal body weight; VT, tidal volume; ETT, endotracheal tube.
Atelectasis overall was found to be far more prevalent in the conventional ventilation group, predominantly affecting the lower lobes in both groups. Two independent reviewers observed significantly less atelectasis in dependent areas using LNVP (reader 1: 36% and reader 2: 16% vs. 64% and 68%, respectively, in conventional ventilation patients; P=0.00014). They also found a decrease in sublobar/lobar atelectasis using LNVP (reader 1: 20% and reader 2: 32%, vs. 48% and 56%, respectively; P=0.01). They reported a trend towards a greater diagnostic yield in the LNVP group (92% vs. 70%), however, this was not statistically significant. Moreover, factors such as their experience with CBCT may have biased those results (authors had less experience with CBCT when they utilized conventional ventilation, and more experience with CBCT when they utilized LNVP).
This was the first report of a dedicated ventilation strategy for bronchoscopy of peripheral lung lesions, which successfully reduced the rate of intraprocedural atelectasis. One of its limitations was the lack in consistency in the ventilatory parameters among patients in both groups (specifically the conventional ventilation strategy). Additionally, it is unclear for how long this ventilatory strategy would be successful during bronchoscopy since the duration of general anesthesia at the time of imaging with CBCT was not reported, and CBCTs obtained during peripheral bronchoscopy (within a few minutes from starting the procedure) were utilized for assessment of atelectasis. LNVP utilized higher levels of PEEP for those patients with lower lobe (more dependent) lung lesions and a BMI of 35 or greater, in order to maintain small airway patency. Despite the very high levels of PEEP, no significant barotrauma, pneumothorax or pneumomediastinum was noted in any of these patients. Strikingly, despite these high levels of intrathoracic pressures achieved, there were no reports of hemodynamic instability. This could be due to the lack of documentation in the absence of a priori definitions of complications.
Ventilatory strategy to prevent atelectasis (VESPA)
Prior to the report of the LNVP study described above, Salahuddin et al. had designed and were conducting a 1:1 prospective, randomized control study to investigate whether a dedicated ventilatory strategy could reduce the incidence of atelectasis during bronchoscopy under general anesthesia as compared to conventional mechanical ventilation. This was the multicenter VESPA trial (NCT04311723) (32). Patients undergoing bronchoscopy for nodal staging with convex-probe endobronchial ultrasound (CP-EBUS) with or without peripheral bronchoscopy were included in this trial.
The control group (conventional mechanical ventilation) received ventilation through a laryngeal mask airway with tidal volume of 6–8 cc/kilogram (kg) of ideal body weight, FiO2 of 100% and 0 cmH2O of PEEP. The treatment group (VESPA protocol) received ventilation through an endotracheal tube, tidal volume of 6–8 cc/kg of ideal body weight, FiO2 less than 100% titrated as low as possible (in order to meet a minimum oxygen saturation of 94%), PEEP of 8–10 cmH2O and an immediate post intubation recruitment maneuver (Table 1). This recruitment maneuver was conducted with 10 consecutive breaths at a plateau pressure of 40 cmH2O, with a PEEP of 20 cmH2O in pressure control mode. This maneuver was repeated in the case of any incidents of accidental disconnection of the ventilatory circuit, or if the endotracheal cuff was deflated to adjust position. Following insertion of the artificial airway, all patients underwent an initial (time 1) CBCT scan of bilateral lung bases, followed by the time 1 RP-EBUS survey for atelectasis. 20–30 minutes after time 1 surveys, the procedure was interrupted to perform the second (time 2) CBCT and RP-EBUS surveys. The RP-EBUS survey was conducted in the identical fashion as the original I-LOCATE trial, with ultrasound images being recorded similarly as either aerated or nonaerated/atelectatic pattern, also read by three independent readers (16). The primary endpoint of the study was to determine if the VESPA protocol could reduce intraprocedural atelectasis during bronchoscopy with general anesthesia, as compared to the conventional ventilation protocol. This was determined by comparing the portion of patients with atelectasis on CBCT at time 2. Secondary endpoints included comparison of atelectasis at time 1, comparison of atelectatic segments as seen by RP-EBUS at time 1 and 2, and a progression of atelectasis between time 1 and time 2 as seen on CBCT.
A total of 76 patients were included in the study, with equal randomization between the two arms. Time 2 (from anesthesia induction to CBCT survey) was 40.95±7.99 min (mean ± SD) for the control group and 43.58±4.82 min (mean ± SD) for the VESPA group. The proportion of patients with any atelectasis (bilateral or unilateral) at time 2 was 84.2% in the control group versus 28.9% in the VESPA group. The portion of patients with bilateral atelectasis at time 2 was 71.1% in the control group versus 7.9% in the VESPA group (P<0.0001). At time 2, 3.84±1.67 (mean ± SD) bronchial segments in the control group vs. 1.21±1.63 in the VESPA group were deemed atelectatic (P<0.0001). There were no major complications in either group, and no significant differences were detected between the 2 groups in terms of complications. There were no cases of pneumomediastinum or pneumothorax. Transient hypotension requiring vasopressors was reported in 10 patients (26.3%) in the control group vs. 12 patients (31.6%) in the VESPA group (P=0.6024).
VESPA is the first and only published randomized control trial where both arms were subjected to strictly controlled ventilation protocols and where the assessment of atelectasis was performed at two different times. The authors purposefully included mostly patients undergoing EBUS for nodal staging, to assess if the VESPA strategy would persist over the duration of the procedure and despite the pro-atelectatic maneuvers that occur with CP-EBUS (large bronchoscope obstructing the airways, bleeding/clotting, suctioning, etc.). One of the strengths of this study was, indeed, its ability to mimic a real-world practice setting where CP-EBUS for nodal staging of lung cancer is performed prior to peripheral bronchoscopy. VESPA not only reduced the incidence of atelectasis during a period long enough to perform nodal staging, but it did so in a safe fashion.
As any study, the VESPA trial also had its limitations. The survey for atelectasis was performed roughly at 40–50 min from induction of anesthesia, and whether VESPA would continue to prevent atelectasis beyond this point is not known. While a staging CP-EBUS could be performed in this period, a combined staging and peripheral bronchoscopy procedure may last longer. The VESPA trial was not designed to evaluate its impact on diagnostic yield for peripheral bronchoscopy (in fact, a minority of patients had peripheral bronchoscopy). It is logical to think that avoiding atelectasis would mostly impact the diagnostic yield of patients with lesions located in dependent areas of the lungs (prone to atelectasis). With the strong evidence showing the high rate of atelectasis in these areas, randomizing those patients to receive or not receive a ventilatory therapy (that is safe and has no additional cost) to prevent atelectasis could be considered unethical, and bronchoscopists may not feel comfortable in conducting such trials. While very effective, the VESPA trial failed to fully eradicate atelectasis, and further highlighted the need for additional strategies to do so, such as positional strategies.
Patient positioning
A novel technique to prevent atelectasis was described by Lin et al., utilizing lateral decubitus positioning for the biopsy of posteriorly located peripheral pulmonary nodules with shape-sensing robotic bronchoscopy and mobile CBCT guidance (33). After anesthesia induction, endotracheal intubation, and a brief bronchoscopic airway exam to clear secretions, patients were turned to the lateral decubitus position with the target lesion side up (Figure 2). Patients were kept in that position with the help of a bean bag and foam straps. The dependent arm was abducted at 90 degrees and the nondependent arm was left in neutral position on the patient’s side. Eleven patients were included in this brief report with a median BMI of 28.7, and lesions located posteriorly at a median of 5.9 mm from the pleura (range, 4.5 to 10.4 mm). Interestingly, the high pressure ventilatory strategies were not utilized in this patient population and they were ventilated through an 8.5 endotracheal tube, utilizing high FiO2 and low PEEP (<5 cmH2O). The median time from induction to the first mobile CBCT scan for tool-in-lesion confirmation was 52 minutes. Despite a conventional ventilation strategy being utilized, none of these 11 patients were found to have atelectasis on the side of the target lesion, including on final CBCT. No complications were reported in this small series. While this technique may not be feasible with other navigational technologies (i.e., electromagnetic navigation), it appears to be safe and highly successful, even more so than VESPA. However, these results need to be confirmed in a prospective trial. Moreover, since it would be cumbersome to perform EBUS for nodal staging in this position, it is also relevant to establish in the future whether lateral decubitus can be used after performing EBUS staging in supine position, and if it able to get rid of established atelectasis. Figure 1 displays an intraprocedural CBCT of one of our patients with a left lower lobe mass (Figure 1A) who initially underwent bronchoscopy in the traditional supine position developing atelectasis that obscured the target lesion (Figure 1B). The patient was then positioned in the right lateral decubitus position, with the left lower lobe now in the non-dependent position. The repeat CBCT image shows resolution of atelectasis, with the target lesion now being completely visible (Figure 1C).
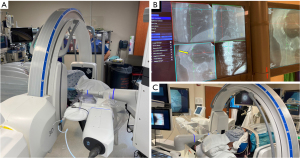
Bhadra et al. described CBCT guided bronchoscopy in one patient who was placed in a prone position for a second bronchoscopic procedure after initial bronchoscopy noted atelectasis in the supine position (34). The second procedure (in prone position) demonstrated no atelectasis. While the patient described here tolerated the procedure well, prone positioning can be logistically challenging if there is limited experience and it could lead to potentially serious complications (accidental extubation, facial trauma). Additionally, while it is very simple and takes a few seconds to transition a patient from lateral decubitus to supine position in case of bleeding or other complications during bronchoscopy, the transition from prone to supine is a longer and harder task.
Conclusions
In conclusion, with the increasing use of CBCT and dedicated studies to investigate the development of intraprocedural atelectasis, it is now established that atelectasis is fairly common during bronchoscopy under general anesthesia. A higher body mass index and increased time under general anesthesia is associated with atelectasis development. The impact of atelectasis on the diagnostic yield of peripheral bronchoscopy has not been specifically studied, but data has already shown that atelectasis can obscure targets (preventing the operators from obtaining samples) and can cause falsely positive RP-EBUS images. This new knowledge has, in fact, led to questioning the standard practice of performing nodal staging with EBUS followed by peripheral bronchoscopy, in fear of allowing atelectasis to set in. To date, VESPA is the only ventilatory strategy that has been properly evaluated in a multicenter randomized controlled fashion. VESPA has been proven to substantially reduce the incidence of atelectasis, to be well-tolerated, and to have a sustained effect over time despite the bronchoscopic maneuvers performed during nodal staging. Promising alternative strategies based on patient positioning are being explored and may be especially useful in patients who cannot tolerate a high PEEP/high-volume ventilatory strategy. The pendulum is headed in the right direction with increased awareness of intraprocedural atelectasis which will continue to lead to studies that compare these different strategies separately or in combination with each other to eradicate atelectasis during bronchoscopy.
Acknowledgments
Funding: None.
Footnote
Conflicts of Interest: All authors have completed the ICMJE uniform disclosure form (available at https://jtd.amegroups.com/article/view/10.21037/jtd-23-97/coif). RFC serves as an unpaid editorial board member of Journal of Thoracic Disease from April 2022 to March 2024. RFC received research grants from Siemens, Intuitive Surgical, and Johnson and Johnson. He is also a paid consultant for Siemens and Intuitive Surgical. The other authors have no conflicts of interest to declare.
Ethical Statement: The authors are accountable for all aspects of the work in ensuring that questions related to the accuracy or integrity of any part of the work are appropriately investigated and resolved.
Open Access Statement: This is an Open Access article distributed in accordance with the Creative Commons Attribution-NonCommercial-NoDerivs 4.0 International License (CC BY-NC-ND 4.0), which permits the non-commercial replication and distribution of the article with the strict proviso that no changes or edits are made and the original work is properly cited (including links to both the formal publication through the relevant DOI and the license). See: https://creativecommons.org/licenses/by-nc-nd/4.0/.
References
- Bendixen HH, Hedley-Whyte J, Laver MB. Impaired oxygenation in surgical patients during general anesthesia with controlled ventilation. a concept of atelectasis. N Engl J Med 1963;269:991-6. [Crossref] [PubMed]
- Ferris BG Jr, Pollard DS. Effect of deep and quiet breathing on pulmonary compliance in man. J Clin Invest 1960;39:143-9. [Crossref] [PubMed]
- Brismar B, Hedenstierna G, Lundquist H, et al. Pulmonary densities during anesthesia with muscular relaxation--a proposal of atelectasis. Anesthesiology 1985;62:422-8. [Crossref] [PubMed]
- Lagier D, Zeng C, Fernandez-Bustamante A, et al. Perioperative Pulmonary Atelectasis: Part II. Clinical Implications. Anesthesiology 2022;136:206-36. [Crossref] [PubMed]
- Martin JB, Garbee D, Bonanno L. Effectiveness of positive end-expiratory pressure, decreased fraction of inspired oxygen and vital capacity recruitment maneuver in the prevention of pulmonary atelectasis in patients undergoing general anesthesia: a systematic review. JBI Database System Rev Implement Rep 2015;13:211-49. [Crossref] [PubMed]
- Hartland BL, Newell TJ, Damico N. Alveolar recruitment maneuvers under general anesthesia: a systematic review of the literature. Respir Care 2015;60:609-20. [Crossref] [PubMed]
- Casal RF, Sarkiss M, Jones AK, et al. Cone beam computed tomography-guided thin/ultrathin bronchoscopy for diagnosis of peripheral lung nodules: a prospective pilot study. J Thorac Dis 2018;10:6950-9. [Crossref] [PubMed]
- Folch EE, Pritchett MA, Nead MA, et al. Electromagnetic Navigation Bronchoscopy for Peripheral Pulmonary Lesions: One-Year Results of the Prospective, Multicenter NAVIGATE Study. J Thorac Oncol 2019;14:445-58. [Crossref] [PubMed]
- Wang Memoli JS, Nietert PJ, Silvestri GA. Meta-analysis of guided bronchoscopy for the evaluation of the pulmonary nodule. Chest 2012;142:385-93. [Crossref] [PubMed]
- Gex G, Pralong JA, Combescure C, et al. Diagnostic yield and safety of electromagnetic navigation bronchoscopy for lung nodules: a systematic review and meta-analysis. Respiration 2014;87:165-76. [Crossref] [PubMed]
- Pritchett MA, Schampaert S, de Groot JAH, et al. Cone-Beam CT With Augmented Fluoroscopy Combined With Electromagnetic Navigation Bronchoscopy for Biopsy of Pulmonary Nodules. J Bronchology Interv Pulmonol 2018;25:274-82. [Crossref] [PubMed]
- Verhoeven RLJ, Fütterer JJ, Hoefsloot W, et al. Cone-Beam CT Image Guidance With and Without Electromagnetic Navigation Bronchoscopy for Biopsy of Peripheral Pulmonary Lesions. J Bronchology Interv Pulmonol 2021;28:60-9. [Crossref] [PubMed]
- Aboudara M, Roller L, Rickman O, et al. Improved diagnostic yield for lung nodules with digital tomosynthesis‐corrected navigational bronchoscopy: Initial experience with a novel adjunct. Respirology 2020;25:206-13. [Crossref] [PubMed]
- Pritchett MA, Bhadra K, Calcutt M, et al. Virtual or reality: divergence between preprocedural computed tomography scans and lung anatomy during guided bronchoscopy. J Thorac Dis 2020;12:1595-611. [Crossref] [PubMed]
- Tusman G, Böhm SH, Warner DO, et al. Atelectasis and perioperative pulmonary complications in high-risk patients. Curr Opin Anaesthesiol 2012;25:1-10. [Crossref] [PubMed]
- Sagar AES, Sabath BF, Eapen GA, et al. Incidence and Location of Atelectasis Developed During Bronchoscopy Under General Anesthesia. Chest 2020;158:2658-66. [Crossref] [PubMed]
- Zeng C, Lagier D, Lee JW, et al. Perioperative Pulmonary Atelectasis: Part I. Biology and Mechanisms. Anesthesiology 2022;136:181-205. [Crossref] [PubMed]
- Albert RK. The role of ventilation-induced surfactant dysfunction and atelectasis in causing acute respiratory distress syndrome. Am J Respir Crit Care Med 2012;185:702-8. [Crossref] [PubMed]
- Hubmayr RD, Sprung J, Nelson S. Determinants of transdiaphragmatic pressure in dogs. J Appl Physiol (1985) 1990;69:2050-6. [Crossref] [PubMed]
- Feldman KS, Kim E, Czachowski MJ, et al. Differential effect of anesthetics on mucociliary clearance in vivo in mice. Sci Rep 2021;11:4896. [Crossref] [PubMed]
- Keller C, Brimacombe J. Bronchial Mucus Transport Velocity in Paralyzed Anesthetized Patients. Anesth Analg 1998;86:1280-2. [PubMed]
- Verbrugge SJ, Böhm SH, Gommers D, et al. Surfactant impairment after mechanical ventilation with large alveolar surface area changes and effects of positive end-expiratory pressure. Br J Anaesth 1998;80:360-4. [Crossref] [PubMed]
- Letsiou E, Kitsiouli E, Nakos G, et al. Mild stretch activates cPLA2 in alveolar type II epithelial cells independently through the MEK/ERK and PI3K pathways. Biochim Biophys Acta 2011;1811:370-6. [Crossref] [PubMed]
- Arold SP, Bartolák-Suki E, Suki B. Variable stretch pattern enhances surfactant secretion in alveolar type II cells in culture. Am J Physiol Lung Cell Mol Physiol 2009;296:L574-81. [Crossref] [PubMed]
- Hedenstierna G, Tokics L, Reinius H, et al. Higher age and obesity limit atelectasis formation during anaesthesia: an analysis of computed tomography data in 243 subjects. Br J Anaesth 2020;124:336-44. [Crossref] [PubMed]
- Reber A, Engberg G, Sporre B, et al. Volumetric analysis of aeration in the lungs during general anaesthesia. Br J Anaesth 1996;76:760-6. [Crossref] [PubMed]
- Casal RF. Cone Beam CT-Guided Bronchoscopy. J Bronchology Interv Pulmonol 2018;25:255-6. [Crossref] [PubMed]
- Chen A, Pastis N, Furukawa B, et al. The effect of respiratory motion on pulmonary nodule location during electromagnetic navigation bronchoscopy. Chest 2015;147:1275-81. [Crossref] [PubMed]
- Tanner NT, Yarmus L, Chen A, et al. Standard Bronchoscopy With Fluoroscopy vs Thin Bronchoscopy and Radial Endobronchial Ultrasound for Biopsy of Pulmonary Lesions. Chest 2018;154:1035-43. [Crossref] [PubMed]
- Kammer MN, Heideman BE, Maldonado F. Should We Start With Navigation or Endobronchial Ultrasound Bronchoscopy? Chest 2022;162:265-8. [Crossref] [PubMed]
- Bhadra K, Setser RM, Condra W, et al. Lung Navigation Ventilation Protocol to Optimize Biopsy of Peripheral Lung Lesions. J Bronchology Interv Pulmonol 2022;29:7-17. [Crossref] [PubMed]
- Salahuddin M, Sarkiss M, Sagar AES, et al. Ventilatory Strategy to Prevent Atelectasis During Bronchoscopy Under General Anesthesia. Chest 2022;162:1393-401. [Crossref] [PubMed]
- Lin J, Sabath BF, Sarkiss M, et al. Lateral Decubitus Positioning for Mobile CT-guided Robotic Bronchoscopy. J Bronchology Interv Pulmonol 2022;29:220-3. [Crossref] [PubMed]
- Bhadra K, Condra W, Setser RM. Out of the Box Thinking. J Bronchology Interv Pulmonol 2022;29:e57-60. [Crossref] [PubMed]