Isoproterenol pre-treatment improve the therapeutic efficacy of cardiosphere-derived cells transplantation for myocardial infarction
Highlight box
Key findings
• 10−6M concentrations of isoproterenol pretreatment could improve the differentiation and transplantation power of cardiosphere-derived cells (CDCs) for myocardial infarction therapy.
What is known and what is new?
• Cardiosphere-derived cells (CDCs) were used to myocardial regeneration.
• Isoproterenol pre-treats the CDCs before transplant into myocardia infraction.
What is the implication, and what should change now?
• Isoproterenol pre-treated CDCs can provide a better protective effect against myocardial infarction than the untreated CDCs.
Introduction
Myocardial infarction (MI) is the most severe form of coronary artery and a leading cause of morbidity and mortality worldwide (1). Acute MI is estimated to cause approximately 7.3 million deaths per year globally (2). Currently, the treatments of coronary artery disease, such as coronary artery bypass grafting and percutaneous transluminal coronary angioplasty, can effectively restore the blood perfusion in the infarct area but cannot repair the damaged myocardium. The infarcted myocardium is gradually replaced with myocardial scarring, which leads to ventricular remodeling and heart failure (3-5). Therefore, that drives the urgency to develop an effective therapeutic method for MI.
Cardiosphere-derived cells (CDCs) therapy is a new experimental therapy for the treatment of end-stage heart disease in recent years (6-8). In both pre-clinical and clinical studies, various types of stem cells, including bone marrow mesenchymal stem cells (9,10), endothelial progenitor cells (11,12), embryonic stem cells (ESCs) (13) and CDCs (13) have been injected through coronary infusion or directly into the infarcted myocardium with the purpose to reduce the infarct size, promote cardiomyocyte regeneration and cardiac function.
However, cell therapy for MI is still challenging, such as the low success rates of colonization of the transplanted cells and differentiation into functional cardiomyocytes and the risk of arrhythmia (14-16). In addition, since the transplanted cells are difficult to survive and proliferate in the harsh microenvironment of the infarct area, traditional stem cell therapy exhibits limited and transient therapeutic efficacy (17,18). Therefore, the keys for successful cell therapy are to improve the local microenvironment of the diseased heart, the colonization, homing, proliferation and differentiation of stem cells, which in turn repairs the damaged myocardium (19).
Cardiosphere-derived cells (CDCs) are a set of special cell subpopulation that can be obtained from clinical cardiac biopsies from all possible sources of seeding cells for cardiomyocytes regeneration (20). CDCs can be induced to differentiate into endothelial progenitor cells, cardiac stromal cells, and cardiomyocytes (20,21). Nevertheless, it has been shown that cardiac progenitor cell transplantation has short-term and limited efficacy, and improvements of the survival, proliferation, colonization, and differentiation of transplanted cells significantly enhance cardiac function and regeneration (17). Three clinical trials were conducted to compare the safety and efficacy of allogeneic versus autologous CDC therapy: interventions for dilated cardiomyopathy using allogeneic myocardially-regenerative cells (DYNAMIC) trial (NCT02293603); the use of allogeneic heart stem cells for myocardial regeneration (ALLSTAR) trial (NCT01458405) in patients post-MI; and a treatment to stop cardiomyopathy progression (HOPE)-Duchenne trial (NCT02485938) in patients with Duchenne muscular dystrophy (22-24). Cardiosphere-derived autologous stem cells to reverse ventricular dysfunction (CADUCEUS) trial also shows the intracoronary delivery of autologous CDCs could reduce the scar size to reverse LV dysfunction after MI (25,26). Another phase-I autologous human cardiac-derived stem cell To treat ischemic cardiomyopathy (ALCADIA) trial (NCT00981006) has shown autologous CDCs treatment could increased LVEF and decreased scar size 6 months after treatment (27).
The β-adrenergic receptors (β-Ars) belong to the superfamily of guanosine-binding protein-coupled receptor (28) and show extensive expression in cardiomyocytes (29,30). Upon ligands binding, the activation of downstream signaling pathways by β-Ars plays a role in regulating a wide range of physiological processes (31). β-Ars signaling has been shown to play important roles in cardiac function (31,32). During the pathogenesis of cardiac failure, the elevated levels of circulating catecholamines continuously over-stimulates β-AR in patient, progressively deteriorating cardiac function of the patient (33). In addition, β1-AR gene knockout leads to defects in embryonic mouse heart development (34), indicating that β1-Ars are implicated in cardiac organogenesis. Yan et al. have reported that isoproterenol (a non-selective β-AR agonist) can induce the differentiation of murine ESCs towards the cardiomyocyte (35). We recently demonstrated that β-Ars signaling pathway and p38 kinases play essential functions in the proliferation and differentiation of CDCs, and isoproterenol treatment can induce CDCs to differentiate into cardiomyocytes (36).
In this study, therefore, we aimed to evaluate if in vitro isoproterenol pre-treatment can enhance the therapeutic efficacy of CDCs transplantation for MI using a rat model. We present this article in accordance with the ARRIVE reporting checklist (available at https://jtd.amegroups.com/article/view/10.21037/jtd-22-1593/rc).
Methods
Animals
Male adult-specific pathogen-free (SPF) Sprague-Dawley (SD) rats (8-week-old, about 200 g) were procured from the Laboratory Animal Center located at Sun Yat-sen University. Experiments were performed under a project license (No. SYSU-IACUC-2020-000509) granted by the Institutional Animal Care and Use Committee (IACUC) of Sun Yat-sen University, in compliance with Chinese national or institutional guidelines for the care and use of animals. A protocol was prepared before the study without registration. All the animal experiments were carried out at China Key Laboratory on Assisted Circulation, Ministry of Health, Guangzhou.
Isolation and expansion of CDCs
The isolation and expansion of CDCs were performed as previously described (27) from 6 rats. Briefly, after a mixture of xylazine (5 mg/kg) and ketamine (100 mg/kg) intraperitoneal injection anesthesia, the opened-chest rat with proximal aortic occlusion was performed by using retrograde perfusion with 10 mL of 4 ℃ cold cardioplegic solution (5% glucose, 68.6 mM mannitol, 12.5 mM potassium chloride, 12.5 mM sodium bicarbonate, 10 U/mL heparin). After cardiac arrest, the heart was extracted and rinsed in cold PBS containing heparin, then cut into 1 mm3 tissue blocks. These tissue blocks were treated twice for 5 min at 37 ℃ with 0.25% trypsin/EDTA, followed by collagenase for 20 min at 37 ℃ and cardiac explant tissue was obtained.
Isolated CDCs, the cardiac explant tissue was subjected to culture by using a complete explant medium (CEM) which contains 78% Iscove’s Modified Dulbecco’s Medium (IMDM), 2 mM L-Glutamine, 0.1 mM two-mercaptoethanol, 20% Fetal Bovine Serum (FBS), and 100 U/mL penicillin-streptomycin. 50% of culture medium was substituted every 3 days with fresh CEM. Following 1 week-long culture, mesenchymal-like cells (CDCs) growing surrounded by the cardiac explant tissue were subjected to trypsinization and cultured with cardiosphere-growth medium (CGM) containing 35% IMDM, 65% DMEM/F-12, 3.5% FBS, 0.1 mM two-mercaptoethanol, 2% B27, 1 U/mL thrombin, 10 ng/mL EGF, 20 ng/mL bFGF, 4 ng/mL Cardiotrophin-1, 2 mM L-glutamine, 100 U/mL penicillin-streptomycin, 50 µg/mL Vit C at cell densities of 2×104/mL. At a confluence of 80–90%, the cultured CDCs were passaged.
Immunofluorescence staining
Glass coverslip-cultured Passage 3 (P3) CDCs were fixed using a 4% paraformaldehyde, the cells were then incubated using primary antibody and fluorescent secondary antibody. Primary antibodies including c-Kit (Santa Cruz, USA), Stem cell antigen-1 (Sca-1, 15 µg/mL, Millipore, USA), Cardiac troponin T (cTnT, Cell Signaling, USA), Nkx-2.5Homeobox protein (NKX-2.5, Santa Cruz, USA), GATA binding protein 4 (GATA-4, Santa Cruz, USA), and cluster of differentiation 34 (CD34, Santa Cruz, USA) antibodies and Cy3 and FITC fluorescent-conjugated secondary antibodies (Abcam, USA; 20 µg/mL) were applied in this study. Olympus BX51 fluorescence microscopy (Olympus, Japan) was used to achieve the fluorescence images.
3-(4,5-Dimethylthiazol-2-yl)-2,5-diphenyltetrazolium bromide (MTT) assay
The MTT assay was used in determining Cell viability. A total of 103 passage 3 CDCs were seeded onto a 96-well plate (n=6 for each group) and treated with the isoproterenol (10−6–10−10 M, designated as I-6–I−10 groups, respectively) or without drug treatment (control group). For colorimetric measurement, 20 µL MTT solution (0.01 M, pH 7.4, Sigma) was added to each well and the cells were incubated for an additional 4 h. After incubation with 150 µL dimethyl sulfoxide, a microplate reader was employed to detect viable cells by measuring absorption at 490 nm (Labsystems Dragon Wellscan MK-3, Finland).
EdU staining
Passage 3 CDCs were seeded onto a 96-well plate (5×103 per well) and cultured with or without isoproterenol (10−6 M) containing medium. EdU (20 µmol/L, Cell-Light™EdU Apollo®567 Cell Tracking Kit, RiboBio, China)-containing medium (100 µL) was added to each well and incubate at 37 ℃ for 72 h, followed by being washed with PBS for 5 min twice. The cells were fixed with 4% paraformaldehyde at room temperature for 30 min, followed by being incubated with glycine (2 mg/mL) at room temperature for 5 min and 0.5% Triton X-100 at room temperature for 10 min. The cells were then stained with Apollo staining solution at room temperature for 30 min in the dark, followed by sequentially incubated with 0.5% Triton X-100 and methanol. The cells were stained with Hoechst 33342 at room temperature for 30 min in the dark. The cells were visualized under a fluorescence microscope (BX51, OLYMPUS, Japan). Edu-positive cell nuclei was light purple.
TUNEL staining
A terminal deoxynucleotidyl transferase dUTP nick-end labeling (TUNEL) assay was used to assess the apoptosis with an apoptosis detection kit (Boster Biological Technology, Ltd., Wuhan, China) according to the manufacturer’s instructions. Passage 3 CDCs (5×103) were seeded onto a coverslip and cultured with or without isoproterenol (10−6 M) containing medium for 72 h. The cells were fixed with 4% paraformaldehyde at room temperature for 30 min and incubated with cold (2–8 ℃) 0.5%TritonX-100 for 2 min. The cells were incubated with 50 µL of TdT enzyme working solution at 37 ℃ for 60 min in the dark. After washing with PBS for 5 min ×3 times, 50 µL of Streptavidin-HRP working solution was added to each slide at 37 ℃ for 60 min in the dark. The slide was incubated with 50~100 µL DAB working solution at room temperature for 10 min. The slide was visualized under a microscope (BX51, OLYMPUS, Japan). Positively stained cell nuclei were dark brown.
Western blot analysis
For protein extraction from myocardial tissue block, tissue block was weighed and treated with ice-cold lysis buffer (60 µL per mg of tissue) with protease inhibitor, followed by being homogenized with the homogenizer at low speed (30 sec each cycle with a 1-min break on ice) until complete lysis. The protein lysate was centrifuged at 12,000 rpm at 4 ℃ for 20 min, and the supernatant was collected and stored at −80 ℃ for further use.
BCA Protein Assay Kit was used in determining Protein concentration (Thermo Scientific, USA). The protein extract underwent electrophoresis using 10% SDS/PAGE gels and later transferred onto PVDF membrane (Merck Millipore, Germany). Antibodies including vimentin (Santa Cruz, USA), cardiac troponin T (cTnT, Santa Cruz, USA), connexin 43 (Cell Signaling, USA), c-Kit (Santa Cruz, USA), CD31 (Santa Cruz, USA), α-smooth muscle actin (α-SMA, Abcam, USA), α-sarcomeric actin (Abcam, USA), rabbit anti-p38α (Santa Cruz, USA), mouse anti p-p38 (Santa Cruz, USA), GAPDH (Proteintech, USA) were all utilized for Western blot analyses. Western blot bands were quantitated by Quantity One software (BioRad, USA).
Establishment of the rat model of MI
In this open-label study, thirty healthy male SD rats were randomly divided into MI group [ligation of the left anterior descending artery (LAD)+PBS injection], MI + CDC group (LAD ligation+CDCs injection) and MI + ISO-CDC group (LAD ligation+isoproterenol pre-treated CDCs injection), (n=8 for each group); and six normal healthy male SD rats in control group (no LAD ligation), and were kept three per cage in a temperature range of 20–24 ℃ room. The randomization sequence of these rats were generated by using a random-number table. The rats were anesthetized by intraperitoneal injection of xylazine (5 mg/kg) and ketamine (100 mg/kg). Then, the animals were intubated and ventilated with an 18-gauge intravenous catheter. Left-sided thoracotomy was performed through a small incision between the third and fourth intercostal spaces. The pericardial sac surrounding the heart was cut to expose the heart. A 6–0 prolene suture was passed left anterior descending coronary artery at about 4 mm away from the origin of the aorta, the needle depth of about 0.5–1.0 mm. Then the left coronary artery together with the myocardium was ligated. After ligation, the myocardial infarct area became purple, and the mobility was significantly reduced. The thorax was closed with the suture until the lungs were inflated completely. At 3 consecutive days after operation, 200,000 U/d of penicillin was intramuscularly injected for anti-infection.
Echocardiography monitoring was performed preoperatively and postoperatively using the echocardiography system (GE VIVID7 10S, USA) in a blinded manner. Both upper limbs and left lower limb of the rat were subcutaneously punctured electrode probe to monitor the limb leads electrocardiogram (20 mm =1 mV, speed =50 mm/sec). An ST elevation in leads II indicated a successful ligation.
CDCs injection treatment
For EdU labeling, the P3 CDCs were cultured with medium containing 10 µM EdU on 100 mm Petri dishes in an incubator (5% CO2, 37 ℃) for 72 h. For isoproterenol pre-treatment, the P3 EdU-labeled CDCs at 80% confluence were treated with 10−6 M of isoproterenol and then cultured for an additional 72 h. The cell suspension (5×107 cells/mL) was prepared before injection.
During establishing the rat model of MI, immediately after ligation of the coronary artery, the CDCs suspension or PBS (MI group) was injected by a sterile microinjector at the four injection points around the infarct area (5 µL each point, total 106 CDCs) in the CDC-treated rats.
Echocardiography
At 3 weeks after operation, rats received an echocardiographic assessment to evaluate the cardiac function. For echocardiographic assessment, the rats were anesthetized by intraperitoneal injection of xylazine (5 mg/kg) and ketamine (100 mg/kg). Then, their upper limbs and left lower limb were subcutaneously punctured with electrode probes. The probe was operated at 11.5 MHz with an image depth of 2.0–4.0 cm. The probe was placed on the left chest of the rat, collecting data from the left ventricular long axis, short axis, and apical four-chamber view, and the echocardiographic data was simultaneously recorded. The following echocardiographic parameters were collected: end-systolic left ventricular internal diameter (LVIDs), end-diastolic left ventricular internal diameter (LVIDd), left ventricular end-systolic volume (LV Vol;s), left ventricular end-diastolic volume (LV Vol;d). fractional shortening of left ventricle (LVFS), and ejection fraction of left ventricle (LVEF). The value for each parameter was obtained by averaging measurements from three successive cardiac cycles.
Measurement of hemodynamic parameters in rats
After the echocardiographic assessment, hemodynamic assessment was performed to evaluate the cardiac function using a BL-420 biological functional system (Chengdu. Thai League Science and Technology Co., Ltd., China). Both upper limbs and left lower limb of the rat were subcutaneously punctured electrode probe to monitor the limb leads electrocardiogram. A 2-cm incision was longitudinally made along the midline of the right side of the neck, and the carotid artery was dissociated about 1 cm. Two #4 silk threads were put at both ends of the dissociated carotid artery. The distal end (craniofacial) thread was ligated, while the proximal thread was tied a slip knot.
A small Incision was made by a 1-mL syringe needle below the ligation site at the distal end of the common carotid artery, and a catheter was inserted into the carotid artery. The slip knot of the proximal thread was loosened to let the catheter pass through and then was tied up to avoid bleeding. The aortic pressure waveform was observed, and the data were recorded after the waveform became stable. The values of the following hemodynamic parameters were recorded: left ventricular end-systolic pressure (LVESP), left ventricular end-diastolic pressure (LVEDP), the maximum rate of left ventricular pressure rise (+LV dP/dt max) and fall (−LV dP/dt max).
Preparation of rat cardiac specimens
After the hemodynamic measurement, 10 mL of 4 ℃ cold cardioplegic solution was injected into the catheter to stop the heartbeat so as to sacrifice the rats and rinse away remaining blood, and the thorax was then open to remove the heart. The heart was placed in ice-cold saline with heparin, followed by taking pictures to record the situation of MI. Each group of specimens from the infarct area was divided into two parts: one was kept immediately into liquid nitrogen for Western Blot. The other one was fixed with 4% paraformaldehyde and cut into 4 equal tissue blocks along the long axis from the apical to the bottom of the heart. After dehydration with sucrose solution, the tissue block was used for frozen section.
Masso’s trichrome staining in the infarct area
For Masso’s trichrome staining, the sections underwent oxidation in a 1% solution of potassium permanganate for 5 min, followed by rinsing in tap water. After 1 min of bleaching involved oxalic acid, the sections were washed in distilled water, stained in Celestine blue and in Maye’s hematoxylin solution for 5 min each, followed by rinsing in running tap water. The sections were then stained with Ponceau-picric acid saturated solution for 5 min, followed by rinsing in 1% acetic acid-water for 2 min, and differentiated in 1% phosphomolybdic acid for 5 min, rinsed in distilled water for 2 min, and stained with either 1% light green or toluidine blue for 30 s. After 1% acetic acid-water treatment, the sections were differentiated in 95% alcohol, hydrated using absolute alcohol, cleared using xylene, and mounted in neutral resin. The slide was visualized by a microscope (BX51, OLYMPUS, Japan). The blue area stained in the sections were measured as fibrosis area using Image J software (version No. 1.53c). Fibrosis size was calculated as percentage of fibrosis area in the left ventricular area, and shown as the average of three section images.
Immunofluorescence staining in the infarct area
Immunofluorescence staining was performed to evaluate the survival, proliferation, and differentiation of EdU-labeled CDCs cells in the infarct area. The tissue section was immunostained for EdU using the Cell-Light™ EdU Apollo®567 Cell Tracking Kit according to the manufacturer’s protocol. Meanwhile, for immunostaining for α-sarcomeric actin, the tissue section was incubated with the primary antibody against α-sarcomeric actin (1:150, Abcam, USA), followed by Cy3-conjugated goat anti-rat IgG (1:100, Abcam, USA). Finally, 4’,6-diamidino-2-phenylindole (DAPI) (10236276001, Sigma) was used as a nuclear dye added in the immunofluorescence staining slide. A fluorescence was used to visualize the slide (BX51, OLYMPUS).
Statistical analysis
All quantitative data were given as mean ± standard deviation (SD). The group with data normality is compared with the group of homogeneity of variance by independent samples t-test. The Mann-Whitney U test was applied to non-normally distributed data. Analysis of variance (ANOVA) with the Bonferroni posthoc test was applied to compare among groups. P value <0.05 as the statistical significant level was set for all comparisons. GraphPad Prism 5 was used in all analysis (GraphPad Software, USA).
Results
Isolation and expansion of CDCs
Rat myocardial tissue was cultured for 7–10 days, and there were some bright round cells growing around the tissue block. A small number of bright, round cells were detached from the tissue block and suspended in the medium. Some cells adhering to the culture dish exhibited spindle-shaped and proliferative. The cells can be passaged in about 5 to 7 days, and the cell still grew well after passage 13 (Figure 1A-1C). Immunofluorescence staining for P3 CDCs showed that some cells were positive for surface markers c-Kit, Sca-1, cTnT, Nkx-2.5, GATA4, and CD34 (Figure 1D).
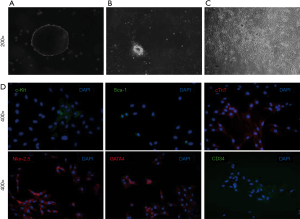
A high concentration of isoproterenol inhibited the proliferation of CDCs
To determine the effect of isoproterenol on the proliferation of CDCs, different concentrations of isoproterenol (10−6–10−10M) were used for the test. As shown in Figure 2A, at 96 h after treatment, a high concentration of isoproterenol (10−6 M) significantly inhibited proliferation of CDCs, whereas low concentrations of isoproterenol (10−8–10−10 M) significantly enhanced proliferation of CDCs (compared to control group, all P<0.05). The concentration of 10−6 M was chosen for isoproterenol treatment in the following experiments.
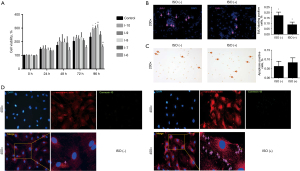
Edu assay and TUNEL staining were performed for isoproterenol-treated CDCs. The results showed that compared to the untreated control, isoproterenol (10−6 M) treatment significantly reduced the Edu-positive CDCs (Figure 2B, P=0.001). TUNEL test showed that the apoptosis rate of CDCs induced by isoproterenol (10−6 M) treatment was not significantly higher than that of untreated controls (Figure 2C, P=0.052). Immunofluorescent staining between control group and 10−6 M isoproterenol group at the 72 h. It showed the CDCs have a better differentiation ability under the 10−6 M isoproterenol pretreatment (Figure 2D). These results suggested that isoproterenol (10−6 M) treatment simultaneously inhibited proliferation of CDCs.
Effects of isoproterenol on the differentiation of CDCs
Next, we evaluate the effect of isoproterenol on the differentiation of CDCs. Western blot showed that compared to the untreated CDCs group, isoproterenol treatment significantly up-regulated the protein levels of vimentin, cTnT, α-sarcomeric actin and connexin 43 (all P<0.05, Figure 3A), but down-regulated c-Kit proteins in CDCs (P<0.05, Figure 3A). However, isoproterenol treatment had no effect on the protein levels of CD31and α-SMA (P>0.05). The results suggested that isoproterenol (10−6 M) treatment promoted the differentiation of CDCs into the myocardium.
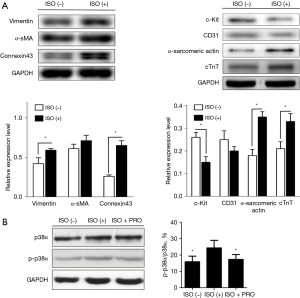
We also conducted a comparative analysis of the activation status of p38 across all groups. In Figure 3B, as indicated, ISO can significantly increase the ratio of p-p38/p38α as compared to the ISO (−) group, however, there was a significant decrease on the ratio of p-p38/p38α in the PRO group (propranolol, 1 µmol/L, Sigma, USA, P<0.05).
Postoperative echocardiography in the rat model of MI
To evaluate the effect of isoproterenol pre-treatment on the therapeutic efficacy of CDCs, a rat model of MI was adopted. The MI rats were treated with PBS (MI group), CDCs (MI + CDC group) or isoproterenol pre-treated CDCs (MI + ISO-CDC group). At 3 weeks after operation, the echocardiographic assessment showed that compared to MI group, MI + CDC group and MI + ISO-CDC group had significantly lower LVIDs, LVIDd (Figure 4A), LV Vol;s, LV Vol;d (Figure 4B), and higher LVFS and LVEF (Figure 4C, all P<0.05). The results indicated that the recovery of cardiac function was better in the two CDCs transplantation groups than in the MI group. However, there was no difference in all the echocardiographic parameters between the MI + CDC group and MI + ISO-CDC group (all P>0.05, Figure 4A).
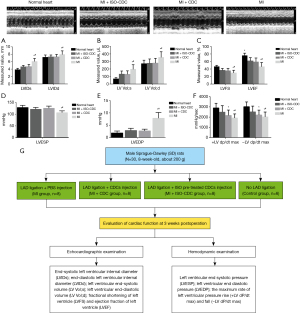
Hemodynamic measurement
Next, the hemodynamic measurement was performed to evaluate cardiac function. As shown in Figure 4D,4E, the two CDCs transplantation groups had a significantly higher LVESP and lower LVEDP than the MI group (all P<0.05). However, there was no difference between MI + CDC and MI + ISO-CDC groups. The +LV dp/dt max and −LV dp/dt max were significantly higher in the MI + ISO-CDC group than in the MI group (Figure 4F, both P<0.05). The experimental schema panel of this study was showed in Figure 4G. These observations consistently indicated that the recovery of cardiac function was better in the two CDCs transplantation groups than in the MI group.
Masson staining
The severity of the myocardial injury was evaluated by Masson staining (Figure 5). Masson staining showed that the residual myocardial tissue and the myocardial thickness in the ischemic region were higher in the MI + ISO-CDC group than in the MI + CDC or MI groups (Figure 5). In addition, the fibrotic area was significantly smaller in the MI + CDC and MI + ISO-CDC groups as compared with the MI group (both P≤0.001, Figure 5).
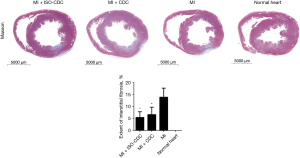
Evaluation of myocardial infarct area by immunofluorescence staining
The severity of the myocardial injury was further evaluated by immunofluorescence staining in the myocardial infarct area. For all the groups, there were abundant non-cardiomyocytes and microvessels in the infarct area (Figure 6). In the MI + ISO-CDC group and MI + CDC group, EdU-positive (proliferating) cells could be observed among the tissues, especially around the small blood vessels. Both the MI + ISO-CDC group and MI + CDC group had significantly more EdU-positive cells as compared with the control group (Figure 6B, P<0.05). The MI + ISO-CDC group had more EdU-positive cells than the MI + CDC group, but the difference did not reach significance. A small number of vascular endothelial cells were also positive for EdU staining, which may be either differentiated from the transplanted CDCs in vivo or the endogenous vascular endothelial cells. The precise cell source remains to be further investigated.
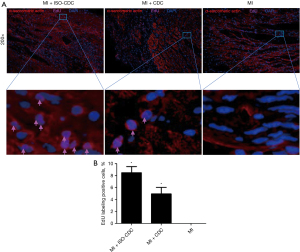
Protein expressions of in the myocardial infarct area
Protein expressions in the infarct area were assessed by Western blot. As shown in Figure 7, the protein levels of connexin 43, cTnT, α-sarcomeric actin and α-SMA were significantly higher in the MI + CDC and MI + ISO-CDC group than in the MI group (P<0.05), while the levels of c-Kit, CD31 and vimentin were not significantly different among the three groups (all P>0.05). In addition, MI + ISO-CDC group had significantly higher levels of α-sarcomeric actin and α-SMA than the MI + CDC group (both P<0.05). The results suggested that the two CDCs transplantation groups had higher myocardium and vascular density than MI group in the myocardial infarct area.
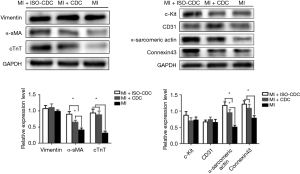
Discussion
In this study, we investigated the effect of isoproterenol pre-treatment on the therapeutic efficacy of CDCs transplantation for MI. The in vitro data showed that isoproterenol treatment (10−6 M) simultaneously inhibited proliferation and induced apoptosis of CDCs, as well as simultaneously up-regulated proteins of vimentin, cTnT, α-sarcomeric actin and connexin 43, and down-regulated c-Kit proteins in CDCs. Echocardiographic assessment in the MI rats showed that the two CDC transplantation groups had significantly smaller LVIDs, LVIDd, LV Vol;s, LV Vol;d and higher LVFS and LVEF than the MI group (all P<0.05) at 3 weeks after operation. Hemodynamic measurement demonstrated that both CDC transplantation groups had a significantly higher LVESP and lower LVEDP than the MI group (both P<0.05). The +LV dp/dt max and −LV dp/dt max were significantly higher in the MI + ISO-CDC group than in the MI group. However, the differences in echocardiographic and hemodynamic data between the two CDC transplantation groups did not reach significant. Masson staining showed MI group had a higher degree of fibrosis in the infarcted myocardium than the two CDC transplantation groups. The MI + ISO-CDC group had more myocardial tissues among the fibrous region than the MI + CDC group. Immunofluorescence staining showed that MI + ISO-CDC group had more EdU-positive cells in the infarcted myocardium than the MI + CDC group. MI + ISO-CDC group had significantly higher protein levels of c-Kit, CD31, cTnT, α-sarcomeric actin, and α-SMA than the MI + CDC group. Taken together, these results suggested that isoproterenol pre-treatment can enhance the therapeutic efficacy of CDCs in MI rats.
The human cardiomyocytes mainly express β1-AR (60–70%), followed by β2-AR (20–30%) and α1-AR (15%) (37). Study have found that in the process of ESCs differentiation into cardiomyocytes, β2-ARs are the main subtype at the early differentiation phase, while β1-AR is the main subtype at late differentiation (35). Isoproterenol treatment can promote the pulsation of human ESC-derived cardiomyocytes (hESC-CM) and human cardiomyocyte progenitor cells (CMPCs), enhancing the function of transplanted cells (38,39). Isoproterenol treatment can enhance mouse embryonic stem differentiation into cardiomyocytes through ERK1/2 and p38 signaling pathways (35).
Isoproterenol promotes hair-follicle-associated pluripotent (HAP) stem cells differentiation into cardiomyocytes. Isoproterenol enhances HAP stem cells to differentiation into cardiac muscle cells (40). Therefore, we chose isoproterenol to pretreat CDCs. Yan et al. have used the concentration range (10−6–10−9 M) of isoproterenol to induce cardiac differentiation in mouse ESCs (35). Therefore, we chose the concentration range (10−6–10−9 M) of isoproterenol for the pre-treatment of CDCs. In our study, it was found that 10−6 M isoproterenol can significantly promote the differentiation of CDCs and inhibited proliferation. Nevertheless, isoproterenol did not completely inhibit the proliferation of CDCs within 72 h of treatment (Figures 2,3). Therefore, we chose to use 72-h isoproterenol pretreated CDCs as a source of transplanted cells.
Our result showed that isoproterenol up-regulated the expression of connexin 43 during differentiation of CDCs, which is in agreement with Salameh et al.’s findings that isoproterenol treatment up-regulate the expression of connexin 43 in cultured neonatal rat cardiomyocytes by βAR-mediated activation of PKA and MAPK (p38, ERK1/2, and JNK) signaling pathways (41,42). Zhang et al. explored the effect of β-AR and p38MAPK signaling pathways on the proliferation and differentiation of CDCs (36). Besides, p38MAPK is one of the downstream molecules of β2-AR and play a negative regulatory role for CDCs differentiation (36).
Their results show that the β1-AR signaling pathway is involved in the differentiation of CDCs, while the β2-AR signaling pathway is involved in the proliferation of CDCs. Isoproterenol can promote the differentiation of CDCs into cardiomyocytes (36). Our study also demonstrates that ISO can activate p38MAPK signaling pathways, while PRO can antagonize this effect of ISO (Figure 3B).
Connexin 43 is the most important gap junction protein in mammalian heart and plays an important role in the intercellular signaling and the maintenance of normal cardiac electrophysiological function (43). It has been shown that in patients with congestive heart failure or dilated cardiomyopathy, the expression of connexin 43 in cardiomyocytes was significantly reduced, while in patients with hypertrophic obstructive cardiomyopathy, the expression of connexin 43 in cardiomyocytes was significantly increased (44). Our results showed that isoproterenol pre-treatment promoted the maturation and differentiation of CDCs and increased the expression of connexin43, cTnT, and α-sarcomeric actin. Connexin43 plays a crucial role in intercellular communication (45), and can promote colonization of transplanted cells (46). Our MTT assay showed that CDCs still retain proliferative capacity within 72 h of isoproterenol treatment. We found that isoproterenol-pretreated CDCs transplantation resulted in more EdU-positive cells in the MI region, which may be attributed to the notion that isoproterenol pre-treatment enhanced the maturation, differentiation, and colonization of CDCs.
Isoproterenol is a broad-spectrum β-AR (β1-AR, β2-AR) agonist. Therefore, the biological effects of isoproterenol on CDCs are complicated and may be related to the concentration of isoproterenol or subtypes of β-AR expression in the local region.
We observed that the low dose of isoproterenol enhanced the proliferation of CDCs, and the high concentration (10−6 M) isoproterenol could significantly inhibit the proliferation of CDCs after 96 h, but the inhibitory effect was not completed within the first 72 h. Meanwhile, isoproterenol promoted the differentiation of CDCs into cardiomyocytes and up-regulates the expression of cTnT and connexin43. It has been shown that Connexin43 plays a crucial role in intercellular communication (45), and can promote colonization of transplanted cells (46). At present, transplanted cells are difficult to colonization in the focal injured region, resulting in the limited effect of stem cell transplantation on cardiac function (47). Combined with the literature and our findings, it is suggested that the use of appropriate concentrations of ISO pre-treatment CDCs can promote the colonization ability of transplanted CDCs, improving the survival rate of transplanted CDCs, and the local microenvironment of damaged myocardium.
Our histological and immunofluorescence findings revealed that at three weeks after operation, MI + ISO-CDC group had the thickest myocardial wall thickness and the most residual myocardium and EdU-positive cells in the infarct area, while MI group had the thinnest myocardial wall thickness, severe myocardial fibrosis, only a few myocardia in the fibrous endocardium. The severity of the injury of the MI + CDC group was between the other two groups. In addition, Western blot showed that MI + ISO-CDC group had higher α-sarcomeric actin protein levels than MI + CDC group and the MI group, which is consistent with morphological observations. Our results showed that isoproterenol pre-treatment promoted CDCs differentiation into the myocardium, but had little effect on the endothelial differentiation and smooth muscle cells, and the expression of connexin43 was significantly increased. The expression of connexin43 protein promotes colonization of transplanted cells (46). It has been found that in addition to the potential for direct differentiation, CDCs have a paracrine effect that promotes the recovery of injured myocardial tissue (1). Our study showed that after MI + ISO-CDC treatment in the MI area, the expression of α-SMA was increased. One possible explanation is that after isoproterenol pre-treatment, the transplanted CDCs increased local colonization, improved the local internal environment, and promoted the proliferation of smooth muscle cells.
The results suggest that isoproterenol pre-treated CDCs can provide a better protective effect against MI than the untreated CDCs. However, the underlying molecular mechanism of the beneficial effect of isoproterenol pre-treatment in CDCs therapeutic efficacy remains to be further investigated.
In the current study, echocardiographic and hemodynamic findings showed that CDCs transplantation significantly improved the cardiac function of MI rats. Although a trend could be observed that MI + ISO-CDC group seemed to have a higher degree of improvement in cardiac function as compared with the MI + CDC group based on the echocardiographic and hemodynamic data, however, the differences between the two CDC transplantation groups did not reach significant. Since the histological data showed that MI + ISO-CDC group had lower severity of injury in the infarct area than the MI + CDC group, the myocardial diastolic and contraction functions were expected to be better in MI + ISO-CDC group than in MI + CDC group. One possible explanation for no significance in cardiac function between the two groups may be the small sample size of this study. Another explanation is that the pre-treatment protocol used in the current study still has room for optimization. The possible optimization may include an increased dose of transplanted CDCs or elongate the observation time.
Since we observed a higher concentration of isoproterenol led to growth inhibition on CDCs, increase concentration may not be a good strategy for optimization. We will further optimize the conditions for isoproterenol pre-treatment of CDCs in subsequent studies. In addition to this limitation, we did not investigate the detailed molecular mechanism of isoproterenol-induced enhancement of differentiation. Follow-up study should address all these limitations.
Conclusions
There are two main hypotheses for heart repair and regeneration: (I) direct cardiomyogenic differentiation, and (II) indirect stimulation of the reparative response by paracrine secreted. Isoproterenol treatment plays a crucial role in regulating CDCs apoptosis and inducing CDCs to differentiate into cardiomyocytes. This study demonstrated that isoproterenol pre-treatment can enhance the therapeutic efficacy of CDCs in MI rats. However, the pre-treatment protocol should be further optimized.
Acknowledgments
Funding: This work was supported by the Project of Administration of Traditional Chinese Medicine of Guangdong Province of China (No. 20211275).
Footnote
Reporting Checklist: The authors have completed the ARRIVE reporting checklist. Available at https://jtd.amegroups.com/article/view/10.21037/jtd-22-1593/rc
Data Sharing Statement: Available at https://jtd.amegroups.com/article/view/10.21037/jtd-22-1593/dss
Conflicts of Interest: All authors have completed the ICMJE uniform disclosure form (available at https://jtd.amegroups.com/article/view/10.21037/jtd-22-1593/coif). The authors have no conflicts of interest to declare.
Ethical Statement: The authors are accountable for all aspects of the work in ensuring that questions related to the accuracy or integrity of any part of the work are appropriately investigated and resolved. Experiments were performed under a project license (No. SYSU-IACUC-2020-000509) granted by the Institutional Animal Care and Use Committee (IACUC) of Sun Yat-sen University, in compliance with Chinese national or institutional guidelines for the care and use of animals.
Open Access Statement: This is an Open Access article distributed in accordance with the Creative Commons Attribution-NonCommercial-NoDerivs 4.0 International License (CC BY-NC-ND 4.0), which permits the non-commercial replication and distribution of the article with the strict proviso that no changes or edits are made and the original work is properly cited (including links to both the formal publication through the relevant DOI and the license). See: https://creativecommons.org/licenses/by-nc-nd/4.0/.
References
- Ashur C, Frishman WH. Cardiosphere-Derived Cells and Ischemic Heart Failure. Cardiol Rev 2018;26:8-21. [Crossref] [PubMed]
- Thomas H, Diamond J, Vieco A, et al. Global Atlas of Cardiovascular Disease 2000-2016: The Path to Prevention and Control. Glob Heart 2018;13:143-63. [Crossref] [PubMed]
- Cahill TJ, Choudhury RP, Riley PR. Heart regeneration and repair after myocardial infarction: translational opportunities for novel therapeutics. Nat Rev Drug Discov 2017;16:699-717. [Crossref] [PubMed]
- Kachanova O, Lobov A, Malashicheva A. The Role of the Notch Signaling Pathway in Recovery of Cardiac Function after Myocardial Infarction. Int J Mol Sci 2022;23:12509. [Crossref] [PubMed]
- Konstam MA, Kramer DG, Patel AR, et al. Left ventricular remodeling in heart failure: current concepts in clinical significance and assessment. JACC Cardiovasc Imaging 2011;4:98-108. [Crossref] [PubMed]
- Grigorian-Shamagian L, Liu W, Fereydooni S, et al. Cardiac and systemic rejuvenation after cardiosphere-derived cell therapy in senescent rats. Eur Heart J 2017;38:2957-67. [Crossref] [PubMed]
- Terzic A, Behfar A. Stem cell therapy for heart failure: Ensuring regenerative proficiency. Trends Cardiovasc Med 2016;26:395-404. [Crossref] [PubMed]
- Nana-Leventaki E, Nana M, Poulianitis N, et al. Cardiosphere-Derived Cells Attenuate Inflammation, Preserve Systolic Function, and Prevent Adverse Remodeling in Rat Hearts With Experimental Autoimmune Myocarditis. J Cardiovasc Pharmacol Ther 2019;24:70-7. [Crossref] [PubMed]
- Zhang GW, Gu TX, Guan XY, et al. HGF and IGF-1 promote protective effects of allogeneic BMSC transplantation in rabbit model of acute myocardial infarction. Cell Prolif 2015;48:661-70. [Crossref] [PubMed]
- Matta A, Nader V, Lebrin M, et al. Pre-Conditioning Methods and Novel Approaches with Mesenchymal Stem Cells Therapy in Cardiovascular Disease. Cells 2022;11:1620. [Crossref] [PubMed]
- Shudo Y, Goldstone AB, Cohen JE, et al. Layered smooth muscle cell-endothelial progenitor cell sheets derived from the bone marrow augment postinfarction ventricular function. J Thorac Cardiovasc Surg 2017;154:955-63. [Crossref] [PubMed]
- Shin HS, Thakore A, Tada Y, et al. Angiogenic stem cell delivery platform to augment post-infarction neovasculature and reverse ventricular remodeling. Sci Rep 2022;12:17605. [Crossref] [PubMed]
- Sun J, Wei T, Bai S, et al. Calcium-sensing receptor-mediated mitogen-activated protein kinase pathway improves the status of transplanted mouse embryonic stem cells in rats with acute myocardial infarction. Mol Cell Biochem 2017;431:151-60. [Crossref] [PubMed]
- Terrovitis JV, Smith RR, Marbán E. Assessment and optimization of cell engraftment after transplantation into the heart. Circ Res 2010;106:479-94. [Crossref] [PubMed]
- Choi YS, Dusting GJ, Stubbs S, et al. Differentiation of human adipose-derived stem cells into beating cardiomyocytes. J Cell Mol Med 2010;14:878-89. [Crossref] [PubMed]
- Coppen SR, Fukushima S, Shintani Y, et al. A factor underlying late-phase arrhythmogenicity after cell therapy to the heart: global downregulation of connexin43 in the host myocardium after skeletal myoblast transplantation. Circulation 2008;118:S138-44. [Crossref] [PubMed]
- Fischer KM, Din S, Gude N, et al. Cardiac progenitor cell commitment is inhibited by nuclear Akt expression. Circ Res 2011;108:960-70. [Crossref] [PubMed]
- Kubo H, Jaleel N, Kumarapeli A, et al. Increased cardiac myocyte progenitors in failing human hearts. Circulation 2008;118:649-57. [Crossref] [PubMed]
- Smart N, Risebro CA, Clark JE, et al. Thymosin beta4 facilitates epicardial neovascularization of the injured adult heart. Ann N Y Acad Sci 2010;1194:97-104. [Crossref] [PubMed]
- Messina E, De Angelis L, Frati G, et al. Isolation and expansion of adult cardiac stem cells from human and murine heart. Circ Res 2004;95:911-21. [Crossref] [PubMed]
- Davis DR, Zhang Y, Smith RR, et al. Validation of the cardiosphere method to culture cardiac progenitor cells from myocardial tissue. PLoS One 2009;4:e7195. [Crossref] [PubMed]
- Makkar RR, Kereiakes DJ, Aguirre F, et al. Intracoronary ALLogeneic heart STem cells to Achieve myocardial Regeneration (ALLSTAR): a randomized, placebo-controlled, double-blinded trial. Eur Heart J 2020;41:3451-8. [Crossref] [PubMed]
- Chakravarty T, Makkar RR, Ascheim DD, et al. ALLogeneic Heart STem Cells to Achieve Myocardial Regeneration (ALLSTAR) Trial: Rationale and Design. Cell Transplant 2017;26:205-14. [Crossref] [PubMed]
- Chakravarty T, Henry TD, Kittleson M, et al. Allogeneic cardiosphere-derived cells for the treatment of heart failure with reduced ejection fraction: the Dilated cardiomYopathy iNtervention with Allogeneic MyocardIally-regenerative Cells (DYNAMIC) trial. EuroIntervention 2020;16:e293-300. [Crossref] [PubMed]
- Malliaras K, Makkar RR, Smith RR, et al. Intracoronary cardiosphere-derived cells after myocardial infarction: evidence of therapeutic regeneration in the final 1-year results of the CADUCEUS trial (CArdiosphere-Derived aUtologous stem CElls to reverse ventricUlar dySfunction). J Am Coll Cardiol 2014;63:110-22. [Crossref] [PubMed]
- Makkar RR, Smith RR, Cheng K, et al. Intracoronary cardiosphere-derived cells for heart regeneration after myocardial infarction (CADUCEUS): a prospective, randomised phase 1 trial. Lancet 2012;379:895-904. [Crossref] [PubMed]
- Takehara N, Ogata T, Nakata M, et al. The ALCADIA (Autologous Human Cardiac-Derived Stem Cell To Treat Ischemic Cardiomyopathy) Trial. Circulation 2012;126:2783.
- Kobilka BK. G protein coupled receptor structure and activation. Biochim Biophys Acta 2007;1768:794-807. [Crossref] [PubMed]
- Xiang Y, Kobilka BK. Myocyte adrenoceptor signaling pathways. Science 2003;300:1530-2. [Crossref] [PubMed]
- Moukette B, Kawaguchi S, Sepulveda MN, et al. MiR-150 blunts cardiac dysfunction in mice with cardiomyocyte loss of β(1)-adrenergic receptor/β-arrestin signaling and controls a unique transcriptome. Cell Death Discov 2022;8:504. [Crossref] [PubMed]
- Madamanchi A. Beta-adrenergic receptor signaling in cardiac function and heart failure. Mcgill J Med 2007;10:99-104. [PubMed]
- Ho D, Yan L, Iwatsubo K, et al. Modulation of beta-adrenergic receptor signaling in heart failure and longevity: targeting adenylyl cyclase type 5. Heart Fail Rev 2010;15:495-512. [Crossref] [PubMed]
- Brinks H, Koch WJ. betaARKct: a therapeutic approach for improved adrenergic signaling and function in heart disease. J Cardiovasc Transl Res 2010;3:499-506. [Crossref] [PubMed]
- Rohrer DK, Desai KH, Jasper JR, et al. Targeted disruption of the mouse beta1-adrenergic receptor gene: developmental and cardiovascular effects. Proc Natl Acad Sci U S A 1996;93:7375-80. [Crossref] [PubMed]
- Yan L, Jia Z, Cui J, et al. Beta-adrenergic signals regulate cardiac differentiation of mouse embryonic stem cells via mitogen-activated protein kinase pathways. Dev Growth Differ 2011;53:772-9. [Crossref] [PubMed]
- Zhang WB, Liu YQ, Zhang X, et al. The role of β-adrenergic receptors and p38MAPK signaling pathways in physiological processes of cardiosphere-derived cells. J Cell Biochem 2018;119:1204-14. [Crossref] [PubMed]
- Dolatshad NF, Hellen N, Jabbour RJ, et al. G-protein Coupled Receptor Signaling in Pluripotent Stem Cell-derived Cardiovascular Cells: Implications for Disease Modeling. Front Cell Dev Biol 2015;3:76. [Crossref] [PubMed]
- Pillekamp F, Haustein M, Khalil M, et al. Contractile properties of early human embryonic stem cell-derived cardiomyocytes: beta-adrenergic stimulation induces positive chronotropy and lusitropy but not inotropy. Stem Cells Dev 2012;21:2111-21. [Crossref] [PubMed]
- Végh AMD, den Haan AD, Cócera Ortega L, et al. Cardiomyocyte Progenitor Cells as a Functional Gene Delivery Vehicle for Long-Term Biological Pacing. Molecules 2019;24:181. [Crossref] [PubMed]
- Yamazaki A, Yashiro M, Mii S, et al. Isoproterenol directs hair follicle-associated pluripotent (HAP) stem cells to differentiate in vitro to cardiac muscle cells which can be induced to form beating heart-muscle tissue sheets. Cell Cycle 2016;15:760-5. [Crossref] [PubMed]
- Salameh A, Krautblatter S, Karl S, et al. The signal transduction cascade regulating the expression of the gap junction protein connexin43 by beta-adrenoceptors. Br J Pharmacol 2009;158:198-208. [Crossref] [PubMed]
- Salameh A, Dhein S. Adrenergic control of cardiac gap junction function and expression. Naunyn Schmiedebergs Arch Pharmacol 2011;383:331-46. [Crossref] [PubMed]
- Beardslee MA, Laing JG, Beyer EC, et al. Rapid turnover of connexin43 in the adult rat heart. Circ Res 1998;83:629-35. [Crossref] [PubMed]
- Boengler K. Stimulation of cardiac beta-adrenoceptors targets connexin 43. Br J Pharmacol 2009;158:195-7. [Crossref] [PubMed]
- Ribeiro-Rodrigues TM, Martins-Marques T, Morel S, et al. Role of connexin 43 in different forms of intercellular communication - gap junctions, extracellular vesicles and tunnelling nanotubes. J Cell Sci 2017;130:3619-30. [Crossref] [PubMed]
- Stoletov K, Strnadel J, Zardouzian E, et al. Role of connexins in metastatic breast cancer and melanoma brain colonization. J Cell Sci 2013;126:904-13. [Crossref] [PubMed]
- Yanamandala M, Zhu W, Garry DJ, et al. Overcoming the Roadblocks to Cardiac Cell Therapy Using Tissue Engineering. J Am Coll Cardiol 2017;70:766-75. [Crossref] [PubMed]