Left ventricular strain and myocardial work in apical hypertrophic cardiomyopathy
Highlight box
Key findings
• Apical hypertrophic cardiomyopathy (ApHCM) is associated with preserved left ventricular (LV) ejection fraction but progressively abnormal longitudinal strain and work indices.
• Important clinical and echocardiographic measures are independently predictive of long-term follow-up LV global longitudinal strain (GLS), global work index (GWI), and adverse events.
What is known and what is new?
• ApHCM variant has been poorly represented in prior studies on longitudinal strain mechanics in hypertrophic cardiomyopathy.
• LV strain mechanics and energetics are moderately impaired in ApHCM, with further reduction occurring over long-term follow-up, particularly in the mid and apical segments.
• Atrial fibrillation, average mitral annular e’ velocity, and glomerular filtration rate are predictors of follow-up GLS and GWI.
• Global wasted work >186 mmHg% is a predictor of composite complications.
What is the implication, and what should change now?
• Contemporary studies suggest significant morbidity with ApHCM, and the pre-sent study provides supportive quantifiable data. Larger studies and longer-term follow-up are needed to confirm our findings.
Introduction
Hypertrophic cardiomyopathy is a heterogeneous genetic disorder with a variable phenotypic expression, and an estimated prevalence of 1:200 to 500 individuals (1,2). Apical hypertrophic cardiomyopathy (ApHCM) variant accounts for 10–25% of all cases, is more commonly seen in males, and may be sporadic or inherited due to myofilament gene mutations (3,4). Initially described in 1970’s Japan and thought to have a more indolent clinical course than other HCM morphologies, contemporary studies have attributed a significant morbidity to ApHCM (4-8). Patients presenting with late-onset ApHCM, have co-existing cardiovascular risk factors, and those with left ventricular (LV) apical aneurysms or wall motion abnormalities appear to be at increased risk (6,7).
Global longitudinal strain (GLS) is a sensitive marker of subclinical myocardial dysfunction and an important predictor of adverse outcomes across HCM phenotypes (9-12). Complementary to GLS has been the development of non-invasive myocardial work estimation, which takes into account LV afterload when assessing GLS to produce LV pressure-strain loops, and has itself been shown to correlate with outcomes, diastology, and degree of fibrosis in non-obstructive HCM (13-16). Importantly, ApHCM patients have not been represented in large proportions in the aforementioned studies, and longitudinal data remain limited. The aim of the present study was to assess LV strain and myocardial work over long-term follow-up in ApHCM using 2D speckle-tracking echocardiography, and to assess for associations between these indices and clinical outcomes. We present this article in accordance with the STROBE reporting checklist (available at https://jtd.amegroups.com/article/view/10.21037/jtd-23-202/rc).
Methods
Patient selection and definitions
The study was conducted in accordance with the Declaration of Helsinki (as revised in 2013). The study was approved by the Mount Sinai Medical Center Institutional Review Board (No. FWA00000176) and individual consent for this retrospective analysis was waived. We retrospectively analyzed the institutional Echocardiography digital database and identified 98 consecutive patients with ApHCM referred for echocardiography between January 2005 and January 2021. The database includes both inpatient and outpatient echocardiograms. Review of the institutional electronic health records was performed to assess patient demographics, clinical risk factors and co-morbidities, and laboratory values. Composite complications were defined as the occurrence of all-cause mortality, sudden cardiac death, myocardial infarction and/or stroke at follow-up.
A diagnosis of ApHCM was the main inclusion criteria. ApHCM was defined as left ventricular wall thickness ≥15 mm distal to the insertion points of the papillary muscles, that is not explained solely by loading conditions (17). Two subtypes of ApHCM include those with apical aneurysm or midventricular obstructive cavity obliteration, respectively (18). Apical aneurysm was defined as discrete and thinned apical segments with dyskinetic contraction and a distinct neck (19,20). Midventricular obstructive cavity obliteration was defined as systolic intra-cavitary LV obliteration and obstruction due to hypertrophied myocardium extending from the apex to include mid-segments (18). Obstruction was defined as a peak instantaneous intra-cavitary pressure gradient of ≥30 mmHg at rest or with provocation. Patients who had any of the following conditions were excluded: (I) untreated or uncontrolled hypertension; (II) hypertensive heart disease; (III) infiltrative cardiomyopathy; (IV) phenocopy conditions (i.e., Andersen-Fabry disease, Danon disease, Friedrich’s ataxia).
2D and speckle-tracking echocardiography
All transthoracic echocardiograms were performed using a GE cardiovascular ultrasound system (General Electric Healthcare, Waukesha, WI, USA). The assessment of cardiac geometry and function was performed in accordance with the American Society of Echocardiography chamber quantification guidelines, and LV diastology in accordance with the diastolic function guidelines (21,22). Specifically, the LV internal diastolic and systolic diameters, and maximal septal and posterior wall thickness were assessed in the parasternal long-axis view. Maximal apical wall thickness was measured in the three standard apical views and in a cross-sectional parasternal short-axis view distal to the papillary muscle insertions. In terms of diastolic assessment, the peak transmitral E-wave velocity was measured to reflect the left atrial to LV pressure gradient, the average mitral annular e’ velocity to reflect LV relaxation, and the E/e’ velocity ratio as a surrogate of LV filling pressure. The mitral valve anatomy and function was assessed in a multi-parametric manner according to the native valvular regurgitation guidelines (23).
Cardiac mechanics were analyzed using the two-dimensional speckle tracking technique via the GE EchoPAC Automated Function Imaging and Q-Analysis software (General Electric Healthcare, Waukesha, WI, USA) according to inter-societal consensus statements on cardiac mechanics quantitation (24,25). Peak full-wall GLS measurements were obtained in the apical four, three and two chamber views, and averaged. Peak systolic strain at the basal, mid, and apical levels was measured and averaged separately in assessing segmental LV longitudinal strain in these individual territories. Myocardial work was calculated by integrating longitudinal strain and blood pressure as estimated by the brachial artery cuff pressure, to generate an LV pressure-strain loop with adjusted ejection and isovolumetric periods. The four myocardial work parameters measured were defined as follows: (I) global work index (GWI), the area of the LV pressure-strain loop between mitral valve opening and closure representing the total LV work performed during systolic ejection and isovolumic relaxation; (II) global constructive work, segmental shortening during systole plus lengthening during isovolumic relaxation; (III) global wasted work, segmental lengthening during systole plus shortening during isovolumic relaxation; and (IV) cardiac efficiency, calculated by the equation: constructive work/(constructive work + wasted work) × 100 (Figure 1) (13).
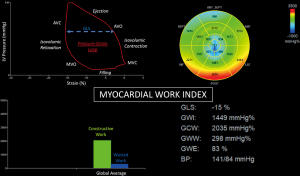
Statistical analysis
Data was analyzed using IBM SPSS Statistics version 21 (IBM Corporation, Armonk, NY). Categorical variables were expressed as number (frequency percentage), while continuous variables were expressed as mean ± standard deviation or median (interquartile range) dependent upon their Gaussian distribution. In the event of missing at random continuous variables, a multiple imputation model using multivariate linear regression was applied (26). Continuous variables with a normal distribution were compared using a paired t-test for repeated measures. Hierarchical multivariable linear regression analysis tested for correlations between baseline clinical and echocardiographic measures and follow-up GLS; step one included the constant and baseline GLS, and step two incorporated exploratory variables whose univariate correlations with follow-up GLS were statistically significant. The same hierarchical multivariable linear regression modeling was performed for GWI. The range estimates and expected treatment effects are expressed as unstandardized and standardized beta coefficients, with a 95% confidence interval. The receiver operating characteristic was applied to GLS and GWI to assess their utility in predicting adverse events at follow-up. A P value <0.05 was considered statistically significant.
Results
Patient demographics and clinical characteristics
The mean age was 64±15 years, 46% were female, and 3% had a family history of HCM. Common cardiovascular co-morbidities included hypertension in 80%, coronary artery disease in 25%, diabetes mellitus in 24%, and congestive heart failure in 13%, of which 54% had New York Heart Association functional class III/IV symptoms. Angina, dyspnea, and syncope were present in 41%, 26%, and 11%, respectively. Electrocardiogram T-wave inversions were observed in 75% (Table 1).
Table 1
Variables | Value [N=98] |
---|---|
Age, years | 64±15 |
Female | 45 [46] |
Heart rate (beats/minute) | 72±15 |
Systolic blood pressure (mmHg) | 130±17 |
Diastolic blood pressure (mmHg) | 74±11 |
Glomerular filtration rate (mL/min/1.73 m2) | 72±25 |
African-American | 17 [17] |
Smoking | 27 [28] |
Family history of HCM | 3 [3] |
HCM signs and symptoms | |
Angina | 40 [41] |
Dyspnea | 25 [26] |
Palpitations | 21 [21] |
Syncope | 11 [11] |
Sudden cardiac death | 0 |
Non-sustained ventricular tachycardia | 9 [9] |
Electrocardiogram T-wave inversions | 73 [75] |
Hypertension | 78 [80] |
Dyslipidemia | 46 [47] |
Diabetes mellitus | 23 [24] |
Coronary artery disease | 24 [25] |
Congestive heart failure | 13 [13] |
New York Heart Association functional class III/IV | 7 [7] |
Cerebrovascular accident | 14 [14] |
Atrial fibrillation/flutter | 30 [31] |
Implantable cardioverter defibrillator | 4 [4] |
History of septal myectomy | 1 [1] |
History of coronary artery bypass graft surgery | 2 [2] |
History of mitral valve replacement | 1 [1] |
Medications | |
Aspirin | 48 [49] |
ACEi/angiotensin receptor blocker | 40 [41] |
Beta-blocker | 58 [59] |
Calcium-channel blocker | 27 [28] |
Direct oral anticoagulant | 19 [19] |
Diuretics | 24 [25] |
Disopyramide | 1 [1] |
P2Y12-inhibitor | 15 [15] |
Warfarin | 3 [3] |
Categorical variables are expressed as n [%], and continuous variables as mean ± standard deviation. ACEi, angiotensin converting enzyme inhibitor; HCM, hypertrophic cardiomyopathy; P2Y12, adenosine diphosphate chemoreceptor.
2D and speckle-tracking echocardiography
Mean LV ejection fraction measured 67%±11%, end-diastolic diameter index was 24±3 mm/m2, maximum apical wall thickness was 19±3 mm, and E/e’ ratio was 13±4. An apical aneurysm was present in 9%. Midventricular obstructive cavity obliteration was present in 3% with provocative maneuvers only. Longitudinal strain and myocardial work parameters were impaired and included an LV GLS measuring −11.7%±3.9%, GWI of 1,073±349 mmHg%, global constructive work of 1,379±449 mmHg%, global wasted work of 233±164 mmHg%, and global work efficiency of 82%±8%. There was a decreasing systolic longitudinal strain gradient from basal (−13.4%±5.1%) to mid (−11.8%±4.8%) and apical LV (−8.5%±7.3%).
Systolic anterior motion of the mitral valve or chordal apparatus was present in 11 (11%) patients, with moderate or greater mitral regurgitation observed in 8 (8%). No patient had evidence of LV outflow tract obstruction. In assessment of the right ventricle, the mean tricuspid annular plane systolic excursion measured 17±4 mm, the basal diameter was 33±5 mm, and the pulmonary artery systolic pressure was 32±12 mmHg (Table 2).
Table 2
Variables | Entire cohort (N=98) | Patients with Follow-up | ||
---|---|---|---|---|
Baseline (N=72) | Follow-up (N=72) | P value | ||
Left ventricular structure and function | ||||
LV ejection fraction (%) | 67±11 | 68±11 | 64±12 | 0.008 |
LV internal diastolic diameter index (mm/m2) | 24±3 | 24±4 | 24±4 | 0.85 |
LV internal systolic diameter index (mm/m2) | 15±4 | 15±4 | 15±4 | 0.26 |
LV mass index (g/m2) | 126±37 | 126±38 | 127±37 | 0.81 |
Septal wall thickness (mm) | 15±4 | 15±4 | 15±4 | 0.5 |
Posterior wall thickness (mm) | 12±2 | 12±2 | 12±2 | 0.66 |
Apical wall thickness (mm) | 19±3 | 18±3 | 19±3 | 0.08 |
Relative wall thickness | 0.53±0.11 | 0.53±0.11 | 0.53±0.13 | 0.91 |
Left ventricular diastology | ||||
Peak transmitral E-wave velocity (m/s) | 0.76±0.21 | 0.78±0.22 | 0.8±0.21 | 0.35 |
Average mitral annular e’ velocity (m/s) | 0.06±0.02 | 0.06±0.02 | 0.05±0.02 | 0.007 |
Average E/e’ ratio | 13±4 | 13±5 | 15±7 | 0.003 |
Right ventricular structure and function | ||||
Right ventricular basal diameter (mm) | 33±5 | 33±5 | 34±6 | 0.26 |
Tricuspid annular plane systole excursion (mm) | 17±4 | 17±3 | 15±4 | <0.001 |
Right ventricular systolic pressure (mmHg) | 32±12 | 35±13 | 36±16 | 0.8 |
Left atrial volume index (mL/m2) | 37±16 | 36±12 | 38±13 | 0.13 |
Data are expressed as mean ± standard deviation.
Follow-up echocardiography
A total of 72 patients had baseline and follow-up echocardiography available for analysis at a median follow-up of 3.9 years [interquartile range (IQR), 1.6–9.2]. There was no difference in characteristics between the follow-up group and the total cohort. When compared with baseline, there was a decrease in the LV ejection fraction (68%±11% vs. 64%±12%, P=0.008), and progressive impairment in GLS (−11.9%±4% vs. −10.7%±3.3%, P=0.006), GWI (1,105±362 vs. 989±343 mmHg%, P=0.02), and global constructive work (1,432±472 vs. 1,312±372 mmHg%, P=0.03), with no change in global wasted work or work efficiency. There was no change in peak systolic longitudinal strain in the LV basal segments, however, significant worsening was observed in the mid (−11.9%±4.9% vs. −10.8%±4.3%, P=0.03) and apical segments (−9.6%±7.1% vs. −6.7%±4.9%, P=0.001). A decrease in the average mitral annular e’ velocity was observed (0.06±0.02 vs. 0.05±0.02 m/s, P=0.007), with a converse increase in the E/e’ ratio (13±5 vs. 15±7, P=0.003). Finally, there was a decrease over follow-up in tricuspid annular plane systolic excursion (TAPSE, 17±3 vs. 15±4, P<0.001), while the right ventricular systolic pressure remained stable (Table 3; Figures 2,3).
Table 3
Left ventricular mechanics | Entire cohort (N=98) | Patients with follow-up | ||
---|---|---|---|---|
Baseline (N=72) | Follow-up (N=72) | P value | ||
Global longitudinal strain (%) | −11.7±3.9 | −11.9±4 | −10.7±3.3 | 0.006 |
Peak systolic basal longitudinal strain (%) | −13.4±5.1 | −13.4±5.2 | −13.4±5.2 | 0.93 |
Peak systolic mid longitudinal strain (%) | −11.8±4.8 | −11.9±4.9 | −10.8±4.3 | 0.03 |
Peak systolic apical longitudinal strain (%) | −8.5±7.3 | −9.6±7.1 | −6.7±4.9 | 0.001 |
Global work index (mmHg%) | 1,073±349 | 1,105±362 | 989±343 | 0.02 |
Global constructive work (mmHg%) | 1,379±449 | 1,432±472 | 1,312±372 | 0.03 |
Global wasted work (mmHg%) | 233±164 | 248±170 | 243±115 | 0.81 |
Global work efficiency (%) | 82±8 | 82±8 | 81±8 | 0.1 |
Basal work efficiency (%) | 88±9 | 88±9 | 88±7 | 0.84 |
Mid work efficiency (%) | 85±10 | 85±10 | 85±8 | 0.68 |
Apical work efficiency (%) | 71±15 | 73±14 | 70±15 | 0.08 |
Data are expressed as mean ± standard deviation.
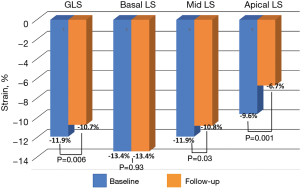
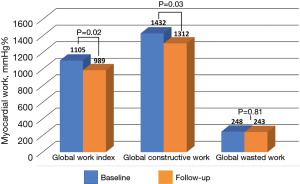
In hierarchical multivariable linear regression analysis, the presence of atrial fibrillation (β=0.37, P<0.001), and measures of average mitral annular e’ velocity (β=−0.32, P=0.001) and glomerular filtration rate (β=−0.2, P=0.03) were significant predictors of follow-up GLS, independent of baseline GLS (β=0.35, P<0.001) (Model fit: r=0.71, P<0.001) (Table 4). Similarly, the presence of atrial fibrillation (β=−0.27, P=0.01) and glomerular filtration rate (β=0.23, P=0.04) were also independent predictors of follow-up GWI, independent of baseline GWI (β=0.27, P=0.02) (Model fit: r=0.51, P<0.001) (Table 5).
Table 4
Variables | Unstandardized B | 95% confidence interval for B | Standardized β | P value | |
---|---|---|---|---|---|
Lower | Upper | ||||
Step 1 | |||||
Constant | −5.8 | −8 | −3.7 | <0.001 | |
GLS (%) | 0.41 | 0.24 | 0.58 | 0.5 | <0.001 |
Step 2 | |||||
Constant | −2.7 | −5.6 | 0.15 | 0.06 | |
GLS (%) | 0.28 | 0.13 | 0.44 | 0.35 | <0.001 |
Atrial fibrillation | 2.6 | 1.3 | 3.9 | 0.37 | <0.001 |
Average mitral annular e’ velocity (m/s) | −58.8 | −92.2 | −25.5 | −0.32 | 0.001 |
Glomerular filtration rate (mL/min/1.73 m2) | −0.03 | −0.05 | −0.003 | −0.2 | 0.03 |
Step 1: initial model including only baseline Global Longitudinal Strain. Step 2: full model with significant variables. Congestive heart failure, septal wall thickness, and left ventricular mass index were univariate correlates that were excluded from the full model due to statistical non-significance. r=0.5, r2=0.24 for step 1; r=0.71, r2=0.5 for step 2 (P<0.001). Negative B/β coefficients indicate negative associations. GLS, global longitudinal strain.
Table 5
Variables | Unstandardized B | 95% confidence interval for B | Standardized β | P value | |
---|---|---|---|---|---|
Lower | Upper | ||||
Step 1 | |||||
Constant | 622 | 376 | 868 | <0.001 | |
Global work index (mmHg%) | 0.33 | 0.12 | 0.54 | 0.35 | 0.003 |
Step 2 | |||||
Constant | 563 | 256 | 870 | <0.001 | |
Global work index (mmHg%) | 0.25 | 0.05 | 0.45 | 0.27 | 0.02 |
Atrial fibrillation | −197.4 | −352.1 | −42.6 | -0.27 | 0.01 |
Glomerular filtration rate (mL/min/1.73 m2) | 3 | 0.17 | 5.8 | 0.23 | 0.04 |
Step 1: initial model including only baseline Global Work Index. Step 2: full model with significant variables. Congestive heart failure was a univariate correlate that was excluded from the full model due to statistical non-significance. r=0.35, r2=0.11 for step 1; r=0.51, r2=0.26 for step 2 (P<0.001). Negative B/β coefficients indicate negative associations.
Clinical outcomes
A total of 5 (5%) all-cause mortality were observed, and 14 (14%) patients had at least one heart failure hospital admission. Of the patients who died, 4 of 5 had a preserved left ventricular ejection fraction (LVEF) ≥55%, while all had an abnormal GLS >−16%. Adverse cardiovascular outcomes included 2 (2%) sudden cardiac deaths, 6 (6%) myocardial infarctions, and 7 (7%) cerebrovascular accidents. Composite complications occurred in 14 (14%) patients. Global wasted work was a fair predictor of composite complications, with a cutoff value of >186 mmHg% having an area under the curve, sensitivity, and specificity of 0.7 (95% CI: 0.53–0.82), 93%, and 41%, respectively. Finally, cardiac surgery was infrequent, and included 1 (1%) coronary artery bypass grafting, 2 (2%) aortic valve replacements, and 1 (1%) septal myectomy in a patient with combined apical and septal hypertrophy.
Discussion
In the present study of patients with ApHCM who underwent 2D and speckle-tracking echocardiography, the following findings are summarized: (I) mean age was 64 years, the majority of patients were male, few patients had a family history of HCM, and the LV ejection fraction and size were normal; (II) LV longitudinal strain mechanics and energetics were moderately impaired, with further reduction in GLS and myocardial work indices over long-term follow-up, particularly in the mid and apical segments; (III) the LV diastolic parameters of mean annular e’ velocity and E/e’ ratio were also impaired and worsened over follow-up; (IV) the presence of atrial fibrillation, average mitral annular velocity, and glomerular filtration rate were significant predictors of follow-up GLS and GWI; and (V) global wasted work >186 mmHg% was a fair predictor of follow-up composite complications.
Despite a normal or hyperdynamic LV ejection fraction in the majority of patients of HCM, it is well established that the myocardial fiber disarray and interstitial fibrosis that characterize the disease significantly impact LV function and energetics (2,27). LV ejection fraction may be overestimated by contribution from mid-wall contraction and in the setting of a small and hypertrophied chamber, and thus, is generally regarded as a suboptimal global marker of LV function. Conversely, GLS is a measure of global longitudinal myocardial shortening and lengthening, and full-wall assessment takes into account subendocardial, mid-wall, and subepicardial function. The inclusion of subendocardial fiber assessment is paramount as they are most at-risk to repetitive injury and may be impaired despite a normal LV ejection fraction. This is particularly useful in subclinical early-stage disease processes or in phenotypes such as HCM (9,10). In the present study, although all regional LV GLS was impaired at baseline, the basal segments were most preserved and did not further deteriorate at follow-up. This LV basal ‘sparing’ pattern may be a useful marker to identify ApHCM patients, however, this hypothesis must be further assessed in additional larger cohorts and in comparison with other cardiomyopathies (Figure 4).
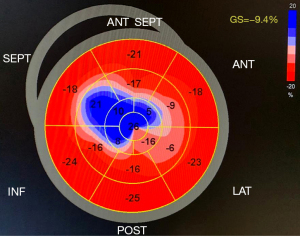
In patients with non-obstructive HCM, GLS has proven useful in risk assessment for adverse cardiovascular events and survival. In a group of 296 patients with non-obstructive HCM and preserved LV ejection fraction, a GLS >−16% was associated with a 5-fold increase in heart failure compared with normal GLS, and increased to 12.5-fold if the LV ejection fraction was preserved at the lower end of normal between 50% to 59% (28). Additionally, a GLS >−10% has been shown to portend a 4-fold increase in ventricular tachyarrhythmias, heart failure, cardiac transplantation, and death (11). Within these studies, ApHCM represented a small proportion of patients and few studies have detailed their LV strain mechanics. In this context, our observations of a markedly decreased GLS at baseline with progressive impairment and worsening LV filling pressure at long-term follow-up is a concerning trend in the natural history of ApHCM.
Assessment of myocardial work indices accounts for not only impaired LV deformation, but also the hemodynamic stress imparted by systemic afterload, for a comprehensive non-invasive analysis of myocardial energetics (13). In a study of 110 patients with non-obstructive HCM, when compared with an age and sex-matched control group, the HCM patients had a significantly lower global work index, constructive work, and work efficiency, with a higher degree of wasted work (29). Of note, only 11 (10%) of patients had ApHCM phenotype. In the present study, ApHCM patients had similarly impaired work indices, with a progressive relative decrease of 11% in global work index and 8% in constructive work at long-term follow-up, respectively. Global wasted work > 186 mmHg% at baseline was identified as a predictor of follow-up composite complications, which is more than double the normal value in healthy hearts, and represents quantification of metabolic burden and myocardial energy waste (13).
The common clinical phenotypic presentation of ApHCM is that of symptomatic heart failure with preserved ejection fraction resulting from chronic microvascular ischemia, myocardial fibrosis, and increased LV filling pressures (18). Patients experience adverse events and recurrent hospitalizations for dyspnea, angina, and tachyarrhythmias, which incur a significant medical and cost burden. Specifically, atrial fibrillation is associated with a 2-fold increase in mortality amongst patients with ApHCM, while an E/e’ ratio >15 has been demonstrated to be an important threshold for decreased event-free survival (6,7). In patients with differing HCM morphologies, chronic kidney disease appears to increase the risk of sudden death and worsens underlying arrhythmic substrates (30). Thus, the present findings of a negative association between atrial fibrillation and decreased mitral annular velocity and glomerular filtration rate with GLS and GWI highlight important co-morbidities that may be therapeutically targeted.
There are limitations to the present study that should be considered. Firstly, this was a retrospective analysis and the sample size is relatively small, which confers a selection bias and limits statistical power. Secondly, ApHCM patients referred for echocardiography are likely to be symptomatic which may affect both imaging findings and clinical outcomes. Nevertheless, the echocardiography database includes inpatient and outpatient referral studies, and is representative of a general community cohort. Thirdly, while clinical long-term follow-up was 100% complete, not all patients had a follow-up echocardiogram available for detailed analysis. Although this is a form of attrition bias and an uncontrollable confounder, it is important to note that no significant differences existed in baseline echocardiographic or clinical characteristics between the entire cohort and those who were analyzed with baseline and follow-up echocardiograms. Fourthly, in certain cases GLS analysis may be difficult to perform in HCM due to the abnormal LV geometry inherent to the disease. This can affect inter- and intra-observer accuracy and reproducibility. Fifthly, adjudication of myocardial infarction did not differentiate between a type I (atherthrombotic coronary artery disease) and type II (oxygen supply and demand mismatch) event. While both are associated with adverse outcomes in the general population, the risk profiles of these patients differ and its translation to the HCM population is not well recognized. Sixthly, cerebrovascular accidents were defined as either ischemic or hemorrhagic, and the distinct prevalence of each subtype was not adjudicated. Although the overall event rate was low, the difference in prognosis is acknowledged as a potential confounder. Seventhly, we observed a reduction in TAPSE at follow-up, which is a surrogate marker for worsening RV systolic function. RV involvement is known to adversely impact clinical outcomes in HCM; however, it is unknown in the present cohort if the observed RV dysfunction was due to deterioration in left-sided function or primary RV myopathy, and requires further investigation (31). Eighthly, contrast echocardiography was not universally performed for all patients, and thus, the prevalence of apical aneurysm may be underestimated. Finally, HCM is a heterogeneous disorder in terms of phenotypic expression, and as such, the current findings are not generalizable to morphologies other than ApHCM, or in those with obstructive physiology. Larger studies and longer-term follow-up are important to confirm our data.
Conclusions
In conclusion, ApHCM is associated with preserved LV ejection fraction but abnormal longitudinal strain mechanics and work indices, with progressive impairment over long-term follow-up. The presence of atrial fibrillation, degree of early LV myocardial relaxation, and glomerular filtration rate are independently associated with follow-up GLS and GWI. A global wasted work >186 mmHg% at baseline is predictive of follow-up composite complications in this group.
Acknowledgments
Funding: None.
Footnote
Reporting Checklist: The authors have completed the STROBE reporting checklist. Available at https://jtd.amegroups.com/article/view/10.21037/jtd-23-202/rc
Data Sharing Statement: Available at https://jtd.amegroups.com/article/view/10.21037/jtd-23-202/dss
Peer Review File: Available at https://jtd.amegroups.com/article/view/10.21037/jtd-23-202/prf
Conflicts of Interest: All authors have completed the ICMJE uniform disclosure form (available at https://jtd.amegroups.com/article/view/10.21037/jtd-23-202/coif). CGM serves as an unpaid editorial board member of Journal of Thoracic Disease from February 2023 to January 2025. The other authors have no conflicts of interest to declare.
Ethical Statement: The authors are accountable for all aspects of the work in ensuring that questions related to the accuracy or integrity of any part of the work are appropriately investigated and resolved. The study was conducted in accordance with the Declaration of Helsinki (as revised in 2013). The study was approved by the Mount Sinai Medical Center Institutional Review Board (No. FWA00000176) and individual consent for this retrospective analysis was waived.
Open Access Statement: This is an Open Access article distributed in accordance with the Creative Commons Attribution-NonCommercial-NoDerivs 4.0 International License (CC BY-NC-ND 4.0), which permits the non-commercial replication and distribution of the article with the strict proviso that no changes or edits are made and the original work is properly cited (including links to both the formal publication through the relevant DOI and the license). See: https://creativecommons.org/licenses/by-nc-nd/4.0/.
References
- Semsarian C, Ingles J, Maron MS, et al. New perspectives on the prevalence of hypertrophic cardiomyopathy. J Am Coll Cardiol 2015;65:1249-54. [Crossref] [PubMed]
- Maron BJ, Desai MY, Nishimura RA, et al. Diagnosis and Evaluation of Hypertrophic Cardiomyopathy: JACC State-of-the-Art Review. J Am Coll Cardiol 2022;79:372-89. [Crossref] [PubMed]
- Jan MF, Todaro MC, Oreto L, et al. Apical hypertrophic cardiomyopathy: Present status. Int J Cardiol 2016;222:745-59. [Crossref] [PubMed]
- Eriksson MJ, Sonnenberg B, Woo A, et al. Long-term outcome in patients with apical hypertrophic cardiomyopathy. J Am Coll Cardiol 2002;39:638-45. [Crossref] [PubMed]
- Sakamoto T, Tei C, Murayama M, et al. Giant T wave inversion as a manifestation of asymmetrical apical hypertrophy (AAH) of the left ventricle. Echocardiographic and ultrasono-cardiotomographic study. Jpn Heart J 1976;17:611-29. [Crossref] [PubMed]
- Moon J, Shim CY, Ha JW, et al. Clinical and echocardiographic predictors of outcomes in patients with apical hypertrophic cardiomyopathy. Am J Cardiol 2011;108:1614-9. [Crossref] [PubMed]
- Klarich KW, Attenhofer Jost CH, Binder J, et al. Risk of death in long-term follow-up of patients with apical hypertrophic cardiomyopathy. Am J Cardiol 2013;111:1784-91. [Crossref] [PubMed]
- Kim EK, Lee SC, Hwang JW, et al. Differences in apical and non-apical types of hypertrophic cardiomyopathy: a prospective analysis of clinical, echocardiographic, and cardiac magnetic resonance findings and outcome from 350 patients. Eur Heart J Cardiovasc Imaging 2016;17:678-86. [Crossref] [PubMed]
- Collier P, Phelan D, Klein A. A Test in Context: Myocardial Strain Measured by Speckle-Tracking Echocardiography. J Am Coll Cardiol 2017;69:1043-56. [Crossref] [PubMed]
- Russo C, Jin Z, Elkind MS, et al. Prevalence and prognostic value of subclinical left ventricular systolic dysfunction by global longitudinal strain in a community-based cohort. Eur J Heart Fail 2014;16:1301-9. [Crossref] [PubMed]
- Liu H, Pozios I, Haileselassie B, et al. Role of Global Longitudinal Strain in Predicting Outcomes in Hypertrophic Cardiomyopathy. Am J Cardiol 2017;120:670-5. [Crossref] [PubMed]
- Yang H, Carasso S, Woo A, et al. Hypertrophy pattern and regional myocardial mechanics are related in septal and apical hypertrophic cardiomyopathy. J Am Soc Echocardiogr 2010;23:1081-9. [Crossref] [PubMed]
- Roemer S, Jaglan A, Santos D, et al. The Utility of Myocardial Work in Clinical Practice. J Am Soc Echocardiogr 2021;34:807-18. [Crossref] [PubMed]
- Russell K, Eriksen M, Aaberge L, et al. A novel clinical method for quantification of regional left ventricular pressure-strain loop area: a non-invasive index of myocardial work. Eur Heart J 2012;33:724-33. [Crossref] [PubMed]
- Hiemstra YL, van der Bijl P, El Mahdiui M, et al. Myocardial Work in Nonobstructive Hypertrophic Cardiomyopathy: Implications for Outcome. J Am Soc Echocardiogr 2020;33:1201-8. [Crossref] [PubMed]
- Gonçalves AV, Rosa SA, Branco L, et al. Myocardial work is associated with significant left ventricular myocardial fibrosis in patients with hypertrophic cardiomyopathy. Int J Cardiovasc Imaging 2021;37:2237-44. [Crossref] [PubMed]
- Ommen SR, Mital S, Burke MA, et al. 2020 AHA/ACC Guideline for the Diagnosis and Treatment of Patients With Hypertrophic Cardiomyopathy: Executive Summary: A Report of the American College of Cardiology/American Heart Association Joint Committee on Clinical Practice Guidelines. J Am Coll Cardiol 2020;76:3022-55. [Crossref] [PubMed]
- Hughes RK, Knott KD, Malcolmson J, et al. Apical Hypertrophic Cardiomyopathy: The Variant Less Known. J Am Heart Assoc 2020;9:e015294. [Crossref] [PubMed]
- Weyman AE, Peskoe SM, Williams ES, et al. Detection of left ventricular aneurysms by cross-sectional echocardiography. Circulation 1976;54:936-44. [Crossref] [PubMed]
- Strachinaru M, Huurman R, Bowen DJ, et al. Relation Between Early Diastolic Mid-Ventricular Flow and Elastic Forces Indicating Aneurysm Formation in Hypertrophic Cardiomyopathy. J Am Soc Echocardiogr 2022;35:846-856.e2. [Crossref] [PubMed]
- Lang RM, Badano LP, Mor-Avi V, et al. Recommendations for cardiac chamber quantification by echocardiography in adults: an update from the American Society of Echocardiography and the European Association of Cardiovascular Imaging. J Am Soc Echocardiogr 2015;28:1-39.e14. [Crossref] [PubMed]
- Nagueh SF, Smiseth OA, Appleton CP, et al. Recommendations for the Evaluation of Left Ventricular Diastolic Function by Echocardiography: An Update from the American Society of Echocardiography and the European Association of Cardiovascular Imaging. J Am Soc Echocardiogr 2016;29:277-314. [Crossref] [PubMed]
- Zoghbi WA, Adams D, Bonow RO, et al. Recommendations for Noninvasive Evaluation of Native Valvular Regurgitation: A Report from the American Society of Echocardiography Developed in Collaboration with the Society for Cardiovascular Magnetic Resonance. J Am Soc Echocardiogr 2017;30:303-71. [Crossref] [PubMed]
- Mor-Avi V, Lang RM, Badano LP, et al. Current and evolving echocardiographic techniques for the quantitative evaluation of cardiac mechanics: ASE/EAE consensus statement on methodology and indications endorsed by the Japanese Society of Echocardiography. J Am Soc Echocardiogr 2011;24:277-313. [Crossref] [PubMed]
- Voigt JU, Pedrizzetti G, Lysyansky P, et al. Definitions for a common standard for 2D speckle tracking echocardiography: consensus document of the EACVI/ASE/Industry Task Force to standardize deformation imaging. J Am Soc Echocardiogr 2015;28:183-93. [Crossref] [PubMed]
- Austin PC, White IR, Lee DS, et al. Missing Data in Clinical Research: A Tutorial on Multiple Imputation. Can J Cardiol 2021;37:1322-31. [Crossref] [PubMed]
- Gonçalves AV, Rosa SA, Branco L, et al. Myocardial work is associated with significant left ventricular myocardial fibrosis in patients with hypertrophic cardiomyopathy. Int J Cardiovasc Imaging 2021;37:2237-44. [Crossref] [PubMed]
- Rowin EJ, Maron BJ, Wells S, et al. Usefulness of Global Longitudinal Strain to Predict Heart Failure Progression in Patients With Nonobstructive Hypertrophic Cardiomyopathy. Am J Cardiol 2021;151:86-92. [Crossref] [PubMed]
- Hiemstra YL, van der Bijl P, El Mahdiui M, et al. Myocardial Work in Nonobstructive Hypertrophic Cardiomyopathy: Implications for Outcome. J Am Soc Echocardiogr 2020;33:1201-8. [Crossref] [PubMed]
- Higuchi S, Minami Y, Shoda M, et al. Effect of Renal Dysfunction on Risk of Sudden Cardiac Death in Patients With Hypertrophic Cardiomyopathy. Am J Cardiol 2021;144:131-6. [Crossref] [PubMed]
- Roşca M, Călin A, Beladan CC, et al. Right ventricular remodeling, its correlates, and its clinical impact in hypertrophic cardiomyopathy. J Am Soc Echocardiogr 2015;28:1329-38. [Crossref] [PubMed]