Myocardial microstructure assessed by T1 mapping after on-pump and off-pump coronary artery bypass grafting
Highlight box
Key findings
• T1 mapping on cardiac magnetic resonance did not find structural myocardial damage after on-pump or off-pump coronary artery bypass grafting, despite the excessive release of cardiac biomarkers, in the absence of procedural myocardial infarction or edema.
What is known and what is new?
• Troponin and CK-MB are released after coronary artery bypass grafting, especially after on-pump procedures. Although this release occurs even in patients with no procedural infarction or edema, it is not associated with cardiac damage assessed by T1 mapping on cardiac magnetic resonance.
What is the implication, and what should change now?
• The isolated release of cardiac biomarkers does not necessarily indicate myocardial tissue damage after coronary artery bypass grafting.
Introduction
Surgical cardiac interventions may impose structural and functional damage to the cardiac tissue, routinely evidenced by the release of myocardial necrosis biomarkers (1,2). This mainly occurs after cardiopulmonary bypass (CPB) surgeries (3). CPB is also associated with the development of a systemic inflammatory response, characterized by a massive release of cytokines and pro-inflammatory mediators, and activation of the complement system (4-7). These reactions may contribute, at least in part, to damage of the myocardial architecture, cellular dysfunction, and tissue necrosis (8).
Cardiac magnetic resonance (CMR) is the gold standard imaging technique for assessing cardiac anatomy and function (9), and late gadolinium enhancement (LGE) is used for non-invasive imaging of myocardial scars and focal fibrosis (10). After myocardial revascularization procedures, CMR and LGE are used to detect myocardial injury, since the isolated use of biomarkers may be insufficient (11). The detection of new LGE in CMR after these procedures has been associated with an excessive release of biomarkers (12). However, in the absence of LGE or myocardial edema, the correlation between the release of biomarkers after revascularization and the development of myocardial tissue damage remains unclear. T1 mapping CMR—which provides a novel, robust, and accurate method of visualizing and quantifying myocardial microstructure in health and disease states, has recently been established (13). The proposed T1-based indices include native T1, which reflects myocardial disease involving the myocyte and interstitium without the need of gadolinium, permitting the noninvasive detection of biologically important processes that may improve the diagnosis and measurement of disease severity (14). Moreover, post-contrast T1 reflects diffuse myocardial abnormalities and quantifies myocardial extracellular volume (ECV), dichotomizes the myocardium into a cellular and an interstitial component, and reflects interstitial changes (15). Additionally, ECV has been shown to be an accurate marker of myocardial tissue remodeling. Therefore, cardiac T1 mapping further extends the ability of CMR to characterize the spectrum of possible myocardial tissue changes associated with coronary revascularization (16). Based on this background, we have hypothesized that coronary artery bypass grafting (CABG) would impose cardiac injury, captured by the release of cardiac biomarkers and changes in T1 mapping on CMR. To test this hypothesis, we aimed to identify whether the release of biomarkers is associated with cardiac tissue damage by assessing myocardial microstructure on T1 mapping after on-pump CABG (ONCAB) and off-pump CABG (OPCAB). We present this article in accordance with the TREND reporting checklist (available at https://jtd.amegroups.com/article/view/10.21037/jtd-23-101/rc).
Methods
Patient selection
This was a pre-specified analysis of the MASS V trial (17), an institutional project of the Heart Institute (InCor), Hospital das Clínicas, University of São Paulo Medical School that was designed to prospectively investigate 202 patients with multivessel coronary artery disease (CAD) with normal left ventricular ejection fraction (LVEF) and a formal indication for CABG or percutaneous coronary intervention. The study was conducted in accordance with the Declaration of Helsinki (as revised in 2013). The Ethics Committee of the Heart Institute of the University of São Paulo, Brazil, approved the study protocol, under the registry SDC 3736/11/154 and informed consent was taken from all the participants. All patients underwent CMR before and after the procedures. From 136 patients who underwent CABG, 69 underwent ONCAB, and 67 with similar arterial and ventricular conditions underwent OPCAB. The inclusion criterion was patients with stable anginal symptoms due to multivessel CAD with preserved LVEF. The exclusion criteria were as follows: recent (≤6 months) myocardial infarction; signs of overt or suspected infections or rheumatologic disease activity; chronic renal failure (creatinine level >2.0 mg/dL); recent (≤6 months) pulmonary embolism or venous thromboembolism; contraindication to CMR, such the presence of a pacemaker or severe claustrophobia; and no provision of consent. For our analysis, we also excluded patients who had LGE or edema on CMR before or after the revascularization procedures and those in whom the T1 map had artifacts. After enrollment, patients were assigned to ONCAB or OPCAB at the discretion of the principal investigator, based on the best strategy to perform a complete anatomical revascularization, and after the evaluation of the cardiac surgeon.
CABG procedures
CABG was performed electively, with or without the use of CPB, by the same team of cardiac surgeons with extensive experience in both ONCAB and OPCAB. Standardized surgical techniques were used, with the same surgical access site, the same aortic and coronary clamping location, antegrade blood cardioplegic solution, normothermic temperature, and anticoagulation protocol. An Octopus stabilizer was used for all OPCAB patients, with the same technique.
CMR and T1 mapping
All patients underwent CMR in a 1.5 Tesla magnetic resonance scanner (Philips Achieva®) 2 days before and 6 days after the surgical interventions during the hospitalization period. The T1 map images were acquired using the modified Look-Locker recovery inversion (MOLLI) technique from the short axis (basal, middle, and apical segments) and comprised the native T1, post-contrast T1, and ECV fraction, which were measured globally and segmentally, but the global values were used (Figure S1) (18). The segmental analysis followed the recommendations of the American Heart Association that established the division of the left ventricle into 16 segments (19). For technical reasons, only one septal region of interest was used for some cases. In these cases, the values of native T1 and global ECV were identical to those of the segments. A careful and standard process was carried out to delimit the myocardial contours and the septal region of interest.
Native T1 was obtained before the gadolinium-based contrast agent (gadoterate meglumine; Gd-DOTA®, Guerbet SA®, France) was administered intravenously (0.1 mmoL/kg body weight). Post-contrast T1 was acquired 15 min after the gadolinium injection. The ECV was calculated using the formula ECV = λ. [1 hematocrit (HCT)]. The partition coefficient (λ) was calculated as λ = ΔR1myocardium/ΔR1blood, where R1 was 1/T1. The end diastolic volumes index (EDVI) of the right ventricle (RV) and left ventricle (LV) EDVI, LV mass, and LVEF were measured using standard methods (20).
All CMR images were analyzed using the cvi42 software (Circle Cardiovascular Imaging Inc. Calgary, Canada) by two experienced observers that were blinded to patients’ biochemical and surgical data. If a consensus was not achieved, the opinion of a third blinded observer was also considered.
Biochemistry
Blood samples were collected for cTnI and CK-MB mass measurements immediately before and at 6, 12, 24, 36, 48, and 72 h after CABG. All samples were centrifuged at 3,000 rpm for 20 min and analyzed within 2 h of collection. Analyses of cTnI and CK-MB were performed using an ADVIA Centaur immunoassay analyzer (Siemens Health Care Diagnostics, Tarrytown, NY, USA). According to the manufacturer, the lower limit of cTnI detection using the high-sensitivity Ultra kit was 0.006 ng/mL, and the 99th percentile upper reference limit (URL) was 0.04 ng/mL. The assay precision represented by the percentage coefficient of variation was 10% at 0.03 ng/mL. The detection limit of the CK-MB mass kit was 0.18 ng/mL. Cutoff values at the 99th percentile was 3.8 ng/mL for women and 4.4 ng/mL for men. These measurements were in accordance with the recommendations of the Study Group on Biomarkers in Cardiology of the European Society of Cardiology Working Group on Acute Cardiac Care (21). For calculating the ECV, HCT values were collected before and after the procedures immediately before CMR.
Electrocardiograms (ECGs)
Twelve-lead ECGs were taken for each patient immediately before and at 6, 12, 24, and 36 h after CABG. To identify new Q waves, the Minnesota code, which is frequently utilized in epidemiological studies and large-scale clinical trials, was used (22).
Statistical analysis
Quantitative variables were presented as means and standard deviations or as medians and interquartile ranges. Qualitative variables were presented as relative frequencies.
Categorical variables were compared using the chi-squared test, Fisher’s exact test, or the likelihood ratio. Quantitative variables were assessed for distribution using the Shapiro-Wilk test. Those with symmetrical distribution were compared using the Student’s t-test, and those with asymmetrical distribution were compared using the Mann-Whitney test. Both CK-MB and troponin peak values were used to assess the association between biomarkers release and T1 mapping parameters. Based on the post-operative CK-MB peak release higher than 10 times the URL, patients were stratified into 2 groups. T1 map and ejection fraction assessed by CMR were compared between these 2 groups by the Mann-Whitney test. This cut-off was chosen because this is the biomarker criteria for considering procedural myocardial infarction related to CABG according to the 3rd Universal Definition of Myocardial Infarction (23). We have chosen CK-MB rather than troponin because in the MASS V trial CK-MB had better accuracy to detect procedural myocardial infarction. In addition, T1 map values after the revascularization procedures were correlated with CK-MB peak release mass using the Spearman method. All hypothesis tests were 2-sided.
The analyses were performed using the statistical package R (version 3.6.2, 12-12-2020; www.R-project.org), and P<0.05 was considered statistically significant.
Results
Between May 2012 and March 2014, 136 prospective patients underwent isolated CABG and pre-and post-CMR. Figure S2 shows the flowchart of the included patients, methodology, and the main results. After excluding 60 patients due to the presence of LGE or edema on CMR before or after the procedures (34 patients) and artifacts in the T1 map images (26 patients), 76 were included in the post-hoc analysis (OPCAB =44 patients, ONCAB =32 patients; Figure S2).
The baseline characteristics of the two groups were similar, except for the SYNTAX score and mean number of grafts, which were significantly higher in the ONCAB group (P=0.002 and P<0.001, respectively). All patients had preserved LVEF. Triple-vessel disease was present in 54 patients (71%), and 74 patients (97.4%) had significant involvement of the left anterior descending artery. The baseline characteristics of the patients are shown in Table 1.
Table 1
Characteristics | OPCAB (n=44) | ONCAB (n=32) | P value |
---|---|---|---|
Demographic profile | |||
Age, mean (SD), year | 60 (9.0) | 61 (7.0) | 0.40 |
Male, n [%] | 30 [68] | 22 [68] | 0.93 |
Medical history | |||
Current or past smoker, n [%] | 13 [29] | 9 [28] | 0.45 |
Hypertension, n [%] | 23 [53] | 17 [52] | 0.56 |
Diabetes mellitus, n [%] | 12 [28] | 10 [31] | 0.61 |
Ejection fraction, mean (SD), % | 0.62 (0.08) | 0.63 (0.06) | 0.58 |
Creatinine, mean (SD), mg/dL | 1.07 (0.28) | 0.99 (0.22) | 0.10 |
Angiographic findings | |||
Triple-vessel disease, n [%] | 27 [61] | 20 [62] | 0.13 |
LAD disease, n [%] | 41 [93] | 29 [91] | 0.46 |
SYNTAX score, median (IQR) | 21 (14.2–24) | 25 (20–30.5) | 0.002 |
Surgery data | |||
Number of grafts, mean (SD) | 2.95 (0.68) | 3.53 (0.51) | <0.001 |
LITA – LAD, n [%] | 42 [95] | 32 [100] | 1.0 |
CPB time, mean (SD), minutes | – | 78.6 (23.2) | |
Anoxia time, mean (SD), minutes | – | 54.7 (18.1) |
OPCAB, off-pump coronary artery bypass; ONCAB, on-pump coronary artery bypass; SD, standard deviation; IQR, interquartile range; LAD, left anterior descending artery; LITA, left internal thoracic artery; CBP, cardiopulmonary bypass.
CMR and T1 mapping analysis
In the OPCAB group, native T1 before and after the procedures was 1,022 ms (995–1,050) and 1,042 ms (991–1,065), respectively (P=0.50; Figure 1A), and ECV was 27.3 (24.4–29.9) and 30.6 (27.1–33.5), respectively (P<0.001; Figure 1B). In the ONCAB group, native T1 before and after the procedure was 1,019 ms (1,004–1,047) and 1,023 ms (1,002–1,056), respectively (P=0.68; Figure 1A), and ECV was 26.1 (23.9–28.4) and 30.7 (28.4–34.9), respectively (P<0.0001; Figure 1B). Native T1 and ECV before and after the procedure showed no significant differences between the two groups.
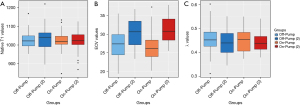
The λ before and after the procedures was 0.45 (0.43–0.48) and 0.44 (0.40–0.48), respectively, in the OPCAB group (P=0.13; Figure 1C), and 0.45 (0.40–0.48) and 0.44 (0.41–0.47), respectively, in the ONCAB group (P=0.79; Figure 1C). There were no significant differences in these values between the two groups.
The LVEF was similar in both groups. Before and after surgery, the mean values were 65% (59–70%) and 67% (60–72%), respectively, in the OPCAB group (P=0.24) and 62% (58–68%) and 67% (62–70%), respectively, in the ONCAB group (P=0.24). Before surgery, LV EDVI and RV EDVI were similar in both groups. Postoperative LV EDVI decreased significantly by 10 mL/m2 (P<0.001) and 6 mL/m2 (P<0.02) in the ONCAB and OPCAB groups, respectively. There were no significant differences in total LV mass or patient weight before and after surgery between the groups (Table 2).
Table 2
CMR findings | OPCAB (n=44) | ONCAB (n=32) | |||||
---|---|---|---|---|---|---|---|
Before | After | P value | Before | After | P value | ||
LVEF, median [IQR], % | 65 [59–70] | 67 [60–72] | 0.24 | 62 [58–68] | 67 [62–70] | 0.24 | |
LV mass, median [IQR], g | 113 [95–137] | 107 [92–119] | 0.27 | 107 [88–123] | 112 [94–135] | 0.27 | |
LVEDVI, median [IQR], mL/m² | 70 [61–79] | 60 [56.5–70] | <0.001 | 67.5 [60–76.5] | 61.5 [56–71] | <0.001 | |
RVEDVI, median [IQR], mL/m² | 62 [52–71] | 57 [52–66] | 0.03 | 64 [56–73] | 56 [50–67] | 0.03 | |
T1 mapping | |||||||
Native T1, median [IQR], ms | 1,022 [995–1,050] | 1,042 [991–1,065] | 0.5 | 1,019 [1,004–1,047] | 1,023 [1,002–1,056] | 0.50 | |
ECV, median [IQR], % | 27.3 [24.4–29.9] | 30.6 [27.1–33.5] | <0.001 | 26.1 [23.9–28.4] | 30.7 [28.4–34.9] | <0.001 | |
Partition coefficient λ, median [IQR] | 0.45 [0.43–0.48] | 0.44 [0.4–0.48] | 0.13 | 0.45 [0.4–0.48] | 0.44 [0.41–0.47] | 0.13 |
CMR, cardiac magnetic resonance; OPCAB, off-pump coronary artery bypass; ONCAB, on-pump coronary artery bypass; IQR, interquartile range; LVEF, left ventricular ejection fraction; IQR, interquartile range; LV, left ventricular; LVEDVI, left ventricular end diastolic volume index; RVEDVI, right ventricular end diastolic volume index; ECV, extracellular volume fraction; λ, partition coefficient.
Biochemistry results
In the OPCAB group, cTnI elevation exceeding 10 times the 99th percentile URL was observed in 40 patients (90.9%). However, CK-MB mass values exceeding 10 times the 99th percentile URL was observed in five patients (11.4%). In the ONCAB group, cTnI elevation values exceeding 10 times the 99th percentile URL were observed in all patients (100%), and CK-MB mass exceeding 10 times the 99th percentile URL was observed in 12 patients (37.5%).
The median peak release of cTnI and CK-MB mass were significantly higher after ONCAB than after OPCAB [3.55 (2.12–4.9) vs. 2.19 (0.69–3.4) ng/mL; P=0.009] and [28.7 (18.2–55.4) vs. 14.3 (9.3–29.2) ng/mL; P=0.009], respectively (Table S1).
All patients were also stratified according to the peak CK-MB values after surgeries in 2 groups: those with peak CK-MB higher than 10 times the URL and those with peak CK-MB lower than 10 times the URL. Of 76 patients, 17 (22.3%) patients had CK-MB values higher than 10 times the URL, and 59 (77.7%) had CK-MB values lower than 10 times the URL. No significant differences in T1 mapping parameters and ejection fraction in CMR were found between these 2 groups (Table 3), with the exception of ECV values.
Table 3
Variables | Peak CK-MB >10xURL (n=17) | Peak CK-MB <10xURL (n=59) | |||||
---|---|---|---|---|---|---|---|
Before | After | P value | Before | After | P value | ||
CK-MB values, median [IQR], ng/mL | 0.92 [0.55–1.45] | 70.2 [54.8–84.1] | <0.001 | 1.10 [0.46–1.50] | 14.5 [10.6–22.9] | <0.001 | |
CMR findings | |||||||
LVEF, median [IQR], % | 63 [61–70] | 66 [61–71] | 0.58 | 64 [58–70] | 67 [60–70] | 0.17 | |
T1 mapping | |||||||
Native T1, median [IQR], ms | 1,009 [999–1,041] | 1,025 [986–1,069] | 0.45 | 1,024 [1,000–1,051] | 1,036 [1,002–1,063] | 0.41 | |
ECV, median [IQR], % | 26.6 [24.0–30.4] | 31.2 [26.9–34.8] | 0.004 | 26.7 [24.5–29.6] | 30.6 [28.0–32.6] | <0.001 | |
Partition coefficient λ, median [IQR] | 0.46 [0.44–0.49] | 0.46 [0.42–0.50] | 0.92 | 0.45 [0.41–0.48] | 0.43 [0.40–0.48] | 0.17 |
CK-MB, creatine kinase myocardial band mass; CMR, cardiac magnetic resonance; URL, upper reference limit; IQR, interquartile range; LVEF, left ventricular ejection fraction; ECV, extracellular volume fraction; λ, partition coefficient.
In addition, no significant correlations were observed between peak CK-MB values and native T1, ECV, partition coefficient lambda or ejection fraction after the procedures (Figure S3A-S3D, respectively).
In both groups, there was a significant decrease in median HCT values after the procedures. Preoperative HCT was 42% (40–45%) in the OPCAB group and 43% (41–46.2%) in the ONCAB group, and postoperative HCT significantly decreased to 31% (28.7–34.2%, P<0.001) and 31% (28–33.2, P<0.001), respectively.
ECG results
No patients in either group had new Q waves, ST segment elevation in two contiguous leads, or new left or right bundle branch block on postoperative ECGs compared to the baseline ECG.
Discussion
The main results of this study show that in the absence of new LGE or edema, OPCAB and ONCAB were not associated with myocardial tissue damage. Despite the excessive release of cardiac biomarkers, the T1 mapping parameters were similar before and after both procedures, suggesting that myocardial integrity was preserved after these procedures.
Interestingly, native T1 showed no differences after surgical intervention in either group. T1 has been reported to be significantly increased in acute myocardial edema in animal and human studies (24,25). Cellular edema, increased extracellular space and water, inflammation, and myocyte necrosis may prolong T1 values in the early stage (26). Therefore, the findings of this study do not support the hypothesis of relevant myocardial damage occurring after these procedures.
Additionally, ECV values increased after the procedure in both groups. This elevation might occur due to an increase in native T1, or more specifically, to the expansion of the extracellular matrix secondary to collagen deposition and diffuse interstitial fibrosis (27-31). However, CMRs in this study were performed one week after surgery on average, and collagen deposition might not have occurred by then. In addition, the increase in ECV was accompanied by a decrease in HCT values after the procedures, possibly due to hemodilution and bleeding related to surgery. On the other hand, the values of the lambda partition coefficient, which represents tissue-specific changes, were not significantly different after the surgeries in either group. This was demonstrated by the mathematical formula for ECV calculation, in which the increase in ECV values occurred in the same magnitude as the decrease in HCT values that occurred after the procedures. Thus, these findings may not be associated with myocardial tissue damage.
Other anatomic and functional parameters of the left and right ventricles, such as LVEF, LV EDVI, RV EDVI, and LV mass, were not compromised, which confirmed the stability of the myocardial microstructure findings. In contrast to previous study (12), the present one showed that LVEF was preserved after coronary revascularization. Notably, there was a slight increase in LVEF after both OPCAB and ONCAB, despite the greater release of cardiac biomarkers after ONCAB. In addition, a reduction in ventricular volume was observed. This might have occurred due to hemodynamic changes after surgery, such as decreased volemic status, or may indicate an improvement in ventricular function related to myocardial revascularization. As expected, there was no difference in LV mass after the procedures. Interestingly, these anatomical results support the findings that T1 mapping showed no myocardial tissue damage after the CABG procedures.
As with previous studies (3), the results of this study show that the peak release of cTnI and CK-MB mass were significantly higher after ONCAB than after OPCAB in the absence of myocardial necrosis. As shown in the MASS V trial, most patients had abnormal troponin release after revascularization surgery. However, <20% of patients had a new LGE in CMR after the procedures. Furthermore, in this trial, CK-MB was more accurate than cTnI for diagnosing periprocedural myocardial infarction, using CMR as the gold-standard (17). In our study, after stratifying patients based on peak CK-MB values greater than or less than 10 times the URL, no significant differences were observed in the T1 map or CMR findings after the interventions. Interestingly, the peak CK-MB values in the group with higher biomarker release reached median values of 70.2 (54.8–84.1) ng/mL, as observed in Table 3. Even in this group, no tissue damage was observed by T1 map or changes in ejection fraction by CMR were detected. Only the ECV values showed a significant increase in post interventions values in both groups.
Finally, no significant correlation was observed between the peak values of postoperative CK-MB and the native T1 and ECV values, confirming the absence of myocardial damage in this population.
Thus, even after excluding patients with LGE (which represented myocardial infarction in this context) and patients with myocardial edema, the higher elevation of cardiac biomarkers (especially troponin) after revascularization interventions, with no evidence of cardiac damage assessed by T1 mapping led to questioning of the pathophysiological processes involved in this release. Further studies may reveal new information regarding this theme.
Therefore, the general results of the present study confirm that in the absence of procedural myocardial infarction or edema, myocardial structure and function are not compromised after revascularization surgeries.
Conclusions
In the absence of procedural documented myocardial infarction, T1 mapping did not identify myocardial tissue damage after surgical revascularization with or without CPB, despite the excessive release of cardiac biomarkers.
Acknowledgments
We would like to thank all members of the MASS-V Trial for their hard work in putting together all the forces needed to perform this study. This work has been presented at the Congress of the Brazilian Society of Cardiology and at the American College of Cardiology Congress.
Funding: This work was supported by the Zerbini Foundation; and the Fundação de Amparo a Pesquisa do Estado de São Paulo (FAPESP) [No. 2011/20876-2]. Medical writing support was provided by Ann Conti Morcos during the preparation of this paper, supported by the Zerbini Foundation.
Footnote
Reporting Checklist: The authors have completed the TREND reporting checklist. Available at https://jtd.amegroups.com/article/view/10.21037/jtd-23-101/rc
Data Sharing Statement: Available at https://jtd.amegroups.com/article/view/10.21037/jtd-23-101/dss
Peer Review File: Available at https://jtd.amegroups.com/article/view/10.21037/jtd-23-101/prf
Conflicts of Interest: All authors have completed the ICMJE uniform disclosure form (available at https://jtd.amegroups.com/article/view/10.21037/jtd-23-101/coif). PCR serves as an unpaid editorial board member of Journal of Thoracic Disease from October 2022 to January 2025. All authors report that this work was supported by the Zerbini Foundation, and the Fundação de Amparo a Pesquisa do Estado de São Paulo (FAPESP) [Grant No. 2011/20876-2]. Grants from Zerbini Foundation were destinated to medical writing support and from FAPESP to cardiac magnetic resonance costs. The authors have no other conflicts of interest to declare.
Ethical Statement: The authors are accountable for all aspects of the work in ensuring that questions related to the accuracy or integrity of any part of the work are appropriately investigated and resolved. The study was conducted in accordance with the Declaration of Helsinki (as revised in 2013). The Ethics Committee of the Heart Institute of the University of São Paulo, Brazil, approved the study protocol, under the registry SDC 3736/11/154 and informed consent was taken from all the participants.
Open Access Statement: This is an Open Access article distributed in accordance with the Creative Commons Attribution-NonCommercial-NoDerivs 4.0 International License (CC BY-NC-ND 4.0), which permits the non-commercial replication and distribution of the article with the strict proviso that no changes or edits are made and the original work is properly cited (including links to both the formal publication through the relevant DOI and the license). See: https://creativecommons.org/licenses/by-nc-nd/4.0/.
References
- Freyholdt T, Massoudy P, Zahler S, et al. Beneficial effect of sodium nitroprusside after coronary artery bypass surgery: pump function correlates inversely with cardiac release of proinflammatory cytokines. J Cardiovasc Pharmacol 2003;42:372-8. [Crossref] [PubMed]
- Kirklin JW, Conti VR, Blackstone EH. Prevention of myocardial damage during cardiac operations. N Engl J Med 1979;301:135-41. [Crossref] [PubMed]
- Alwan K, Falcoz PE, Alwan J, et al. Beating versus arrested heart coronary revascularization: evaluation by cardiac troponin I release. Ann Thorac Surg 2004;77:2051-5. [Crossref] [PubMed]
- Levy JH, Tanaka KA. Inflammatory response to cardiopulmonary bypass. Ann Thorac Surg 2003;75:S715-20. [Crossref] [PubMed]
- Warren OJ, Smith AJ, Alexiou C, et al. The inflammatory response to cardiopulmonary bypass: part 1--mechanisms of pathogenesis. J Cardiothorac Vasc Anesth 2009;23:223-31. [Crossref] [PubMed]
- Paparella D, Yau TM, Young E. Cardiopulmonary bypass induced inflammation: pathophysiology and treatment. An update. Eur J Cardiothorac Surg 2002;21:232-44. [Crossref] [PubMed]
- Asimakopoulos G. Systemic inflammation and cardiac surgery: an update. Perfusion 2001;16:353-60. [Crossref] [PubMed]
- Laffey JG, Boylan JF, Cheng DC. The systemic inflammatory response to cardiac surgery: implications for the anesthesiologist. Anesthesiology 2002;97:215-52. [Crossref] [PubMed]
- h-Ici DO, Jeuthe S, Al-Wakeel N, et al. T1 mapping in ischaemic heart disease. Eur Heart J Cardiovasc Imaging 2014;15:597-602.
- Haaf P, Garg P, Messroghli DR, et al. Cardiac T1 Mapping and Extracellular Volume (ECV) in clinical practice: a comprehensive review. J Cardiovasc Magn Reson 2016;18:89. [Crossref] [PubMed]
- Testa L, Van Gaal WJ, Biondi Zoccai GG, et al. Myocardial infarction after percutaneous coronary intervention: a meta-analysis of troponin elevation applying the new universal definition. QJM 2009;102:369-78. [Crossref] [PubMed]
- Selvanayagam JB, Petersen SE, Francis JM, et al. Effects of off-pump versus on-pump coronary surgery on reversible and irreversible myocardial injury: a randomized trial using cardiovascular magnetic resonance imaging and biochemical markers. Circulation 2004;109:345-50. [Crossref] [PubMed]
- Puntmann VO, Peker E, Chandrashekhar Y, et al. T1 Mapping in Characterizing Myocardial Disease: A Comprehensive Review. Circ Res 2016;119:277-99. [Crossref] [PubMed]
- Moon JC, Messroghli DR, Kellman P, et al. Myocardial T1 mapping and extracellular volume quantification: a Society for Cardiovascular Magnetic Resonance (SCMR) and CMR Working Group of the European Society of Cardiology consensus statement. J Cardiovasc Magn Reson 2013;15:92. [Crossref] [PubMed]
- Mavrogeni S, Apostolou D, Argyriou P, et al. T1 and T2 mapping in Cardiology: Mapping the Obscure Object of Desire. Cardiology 2017;138:207-17. [Crossref] [PubMed]
- Schelbert EB, Messroghli DR. State of the Art: Clinical Applications of Cardiac T1 Mapping. Radiology 2016;278:658-76. [Crossref] [PubMed]
- Hueb W, Gersh BJ, Alves da Costa LM, et al. Accuracy of Myocardial Biomarkers in the Diagnosis of Myocardial Infarction After Revascularization as Assessed by Cardiac Resonance: The Medicine, Angioplasty, Surgery Study V (MASS-V) Trial. Ann Thorac Surg 2016;101:2202-8. [Crossref] [PubMed]
- Messroghli DR, Radjenovic A, Kozerke S, et al. Modified Look-Locker inversion recovery (MOLLI) for high-resolution T1 mapping of the heart. Magn Reson Med 2004;52:141-6. [Crossref] [PubMed]
- Cerqueira MD, Weissman NJ, Dilsizian V, et al. Standardized myocardial segmentation and nomenclature for tomographic imaging of the heart. A statement for healthcare professionals from the Cardiac Imaging Commitee of the Council on Clinical Cardiology of the American Heart Association. Circulation 2002;105:539-42. [Crossref] [PubMed]
- Schulz-Menger J, Bluemke DA, Bremerich J, et al. Standardized image interpretation and post processing in cardiovascular magnetic resonance: Society for Cardiovascular Magnetic Resonance (SCMR) board of trustees task force on standardized post processing. J Cardiovasc Magn Reson 2013;15:35. [Crossref] [PubMed]
- Thygesen K, Mair J, Giannitsis E, et al. How to use high-sensitivity cardiac troponins in acute cardiac care. Eur Heart J 2012;33:2252-7. [Crossref] [PubMed]
- Crow RS, Prineas RJ, Jacobs DR Jr, et al. A new epidemiologic classification system for interim myocardial infarction from serial electrocardiographic changes. Am J Cardiol 1989;64:454-61. [Crossref] [PubMed]
- Thygesen K, Alpert JS, Jaffe AS, et al. Third universal definition of myocardial infarction. Writing Group on the Joint ESC/ACCF/AHA/WHF Task Force for the Universal Definition of Myocardial Infarction, ESC Committee for Practice Guidelines (CPG). Eur Heart J 2012;33:2551-67. [Crossref] [PubMed]
- Ugander M, Bagi PS, Oki AJ, et al. Myocardial edema as detected by pre-contrast T1 and T2 CMR delineates area at risk associated with acute myocardial infarction. JACC Cardiovasc Imaging 2012;5:596-603. [Crossref] [PubMed]
- Ferreira VM, Piechnik SK, Dall'Armellina E, et al. Non-contrast T1-mapping detects acute myocardial edema with high diagnostic accuracy: a comparison to T2-weighted cardiovascular magnetic resonance. J Cardiovasc Magn Reson 2012;14:42. [Crossref] [PubMed]
- Ferreira VM, Piechnik SK, Dall’Armellina E, et al. T (1) mapping for the diagnosis of acute myocarditis using CMR: comparision to T2-weighted and late gadolinium enhanced imaging. JACC Cardiovasc Imaging 2013;6:1048-58. [Crossref] [PubMed]
- Ferreira VM, Piechnik SK, Robson MD, et al. Myocardial tissue characterization by magnetic resonance imaging: novel applications of T1 and T2 mapping. J Thorac Imaging 2014;29:147-54. [Crossref] [PubMed]
- Liu CY, Liu YC, Wu C, et al. Evaluation of age-related interstitial myocardial fibrosis with cardiac magnetic resonance contrast-enhanced T1 mapping: MESA (Multi-Ethnic Study of Atherosclerosis). J Am Coll Cardiol 2013;62:1280-7. [Crossref] [PubMed]
- Kellman P, Wilson JR, Xue H, et al. Extracellular volume fraction mapping in the myocardium, part 2: initial clinical experience. J Cardiovasc Magn Reson 2012;14:64. [Crossref] [PubMed]
- Fontana M, White SK, Banypersad SM, et al. Comparison of T1 mapping techniques for ECV quantification. Histological validation and reproducibility of ShMOLLI versus multibreath-hold T1 quantification equilibrium contrast CMR. J Cardiovasc Magn Reson 2012;14:88. [Crossref] [PubMed]
- Wong TC, Piehler K, Meier CG, et al. Association between extracellular matrix expansion quantified by cardiovascular magnetic resonance and short-term mortality. Circulation 2012;126:1206-16. [Crossref] [PubMed]