Prevalence of early bacterial co-infection in hospitalized patients with COVID-19 pneumonia: a retrospective study
Highlight box
Key findings
• The prevalence of early bacterial co-infection in patients with COVID-19 pneumonia was low but it was associated with mortality.
What is known and what is new?
• Early bacterial co-infection in patients with COVID-19 pneumonia is rare with variable reported prevalence across the world.
• The present study demonstrated several factors associated with an early bacterial co-infection in patients with COVID-19 pneumonia.
What is the implication, and what should change now?
• COVID-19 pneumonia patients with these associated factors, an early bacterial co-infection should be considered in order to expedite antibiotic prescription.
Introduction
The coronavirus disease 2019 (COVID-19) pandemic has been a global healthcare emergency, causing millions of deaths worldwide. The COVID-19 mortality rate in Thailand was 1.03%. About 64% of these deaths are patients with comorbidities. There are limited reports on well-established risk factors for disease progression and mortality in Thai patients. One of the most anticipated risk factors is a bacterial infection, including early bacterial co-infection and nosocomial bacterial infection, which the latter appears to be more frequent with a prevalence of 45–71% (1-3). The definition of early bacterial co-infection may vary among studies, but it generally refers to bacterial infection occurring within the first 48 hours of hospitalization. Diagnosing early bacterial co-infection in COVID-19 pneumonia patients is challenging because the clinical and radiological features can overlap and cannot be distinguished from viral pneumonia. The appropriate tests might be necessary, including respiratory specimen culture, other laboratory tests, and serum biomarkers. In previous reports on the influenza pandemic, the prevalence of bacterial co-infection was high, ranging from 30–50% in severe influenza which was associated with increased mortality (4-7). Several observational studies have reported the prevalence of early bacterial co-infection in patients with COVID-19 pneumonia ranging from 1.2% to 5.5% which was lower than that in influenza (8-10).
Bacterial co-infection in hospitalized patients with COVID-19 pneumonia may worsen clinical outcomes and increase mortality. However, early studies have demonstrated that early bacterial co-infection is uncommon. The most commonly reported causative pathogens included methicillin-susceptible Staphylococcus aureus (MSSA), Hemophilus influenzae, and Streptococcus pneumoniae, respectively (10-14). The patients with bacterial co-infection also had a 3-time higher risk of mortality and had 13 days longer hospital stay (15).
Identification of bacterial co-infection is crucial in determining patient outcomes and deciding whether to start or discontinue broad-spectrum antibiotics in order to avoid antibiotic overuse. In Thailand, the data on the prevalence of early bacterial co-infection in patients with COVID-19 pneumonia is limited. The present study aimed to evaluate the prevalence of early bacterial co-infection and its associated factors. We present this article in accordance with the STROBE reporting checklist (available at https://jtd.amegroups.com/article/view/10.21037/jtd-22-1681/rc).
Methods
The present study is a single-center, retrospective study conducted at the Division of Respiratory Disease and Tuberculosis, Department of Medicine, Faculty of Medicine Siriraj Hospital, Mahidol University, Thailand. The primary outcome was to evaluate the prevalence of early bacterial co-infection in hospitalized patients with COVID-19 pneumonia at Siriraj hospital. The secondary outcomes included the predictors of early bacterial co-infection, characteristics of the patients and chest X-ray, causative pathogens, and clinical outcomes. The study was conducted in accordance with the Declaration of Helsinki (as revised in 2013) and approved by the Institutional Review Board of the Faculty of Medicine Siriraj Hospital (COA No. SI901/2564). Informed consent was waived due to the retrospective nature of the study.
All patients with COVID-19 pneumonia who were admitted to either intensive care units or in-patient wards between April 1 and August 31, 2021 were reviewed for eligibility. The inclusion criteria included patients at least 18 years of age, who had confirmed severe acute respiratory syndrome coronavirus 2 (SARS-CoV-2) infection from the nasopharyngeal swab, expectorated sputum, or tracheal aspirate using the reverse transcriptase-polymerase chain reaction (RT-PCR) technique within 7 days and were hospitalized within 48 hours at the time of SARS-CoV-2 infection diagnosis. Pneumonia is defined by having compatible signs and symptoms (fever >38 ℃ plus at least 1 symptom of cough, purulent sputum, dyspnea, pleuritic chest pain, respiratory rate >20 times per minute, crackles, rhonchi, or signs of consolidation from physical examination) and having abnormal chest X-ray, including alveolar opacities, consolidation, interstitial opacities, or ground-glass opacities. The exclusion criteria included patients who were admitted for more than 48 hours before the diagnosis of SARS-CoV-2 infection, were admitted to another hospital for more than 48 hours, had a history of hospital admission within 14 days, or had no respiratory symptoms.
Data of eligible patients were obtained from electronic medical records using specific ICD-10 codes (U07.1 COVID-19, virus identified, and J128, Other viral pneumonia). Patients were randomly enrolled using computer-based randomization. The patients were classified as the “Early bacterial co-infection” group if they had microbiological evidence of bacterial co-infection (including positive hemoculture, sputum culture, nasopharyngeal swab PCR, and sputum PCR for respiratory pathogens) obtained within 48 hours after hospitalization or met the criteria for probable bacterial co-infection, defined by having at least 2 systemic inflammatory response syndrome (SIRS) criteria and was treated with antibiotics for at least 5 days (16,17). The diagnosis of probable bacterial co-infection was confirmed by 2 pulmonologists. The remaining patients were classified as the “Unlikely early bacterial co-infection” group. Clinical characteristics, chest X-ray findings, laboratory and microbiological results, hospital course, complications, and patient outcomes were assessed. Chest X-rays were obtained within 48 hours after admission and were assessed by one pulmonologist and one chest radiologist. Radiographic findings were classified as characteristic (bilateral patchy and/or confluent, bandlike ground-glass opacity or consolidation, peripheral and mid to lower lung zone distribution), non-specific (focal, unilateral opacity, ill-defined bibasilar opacity, diffuse opacity, upper lobe predominance, mass-liked opacity, effusion), and negative (normal) according to the previously proposed radiographic classification (18). A subsequent consensus read was performed to address discrepancies between the two readers. The Brixia chest X-ray score was used to grade lung abnormalities and was interpreted by one pulmonologist and one chest radiologist who were blinded to clinical data (19,20). The overall Brixia chest X-ray score ranged from 0 to 18 which higher scores indicating more severe disease. The Brixia chest X-ray score was used to further assess the correlation between the severity of chest X-rays and outcomes (20).
Statistical analyses
Sample size estimation was performed based on the previously reported prevalence of early bacterial co-infection and the sample size equation for the descriptive study (10,21). Type 1 error, confidence interval width and expected prevalence were set at 5%, 3%, and 5.5%, respectively. The percentage of missing data was set at 10%, thus at least 245 subjects were needed for analysis.
Categorical data were presented as frequencies and percentages. Continuous data were presented as mean, range, and standard deviation (SD) if they were normally distributed, or median and interquartile range (IQR) if they were not normally distributed. Categorical data were compared using the Chi-square test or Fisher’s exact test. Continuous data were compared using an independent t-test or Mann-Whitney U test. Interobserver agreement analyses were performed using Cohen’s weighted kappa if the data were categorical data, and intraclass correlation coefficient if the data were continuous data. Multivariate logistic regression analysis was used to assess the factors associated with early bacterial co-infection. A P value of less than 0.05 was considered statistically significant. All statistical analyses were performed using statistical software (SPSS for Windows, version 18.0; SPSS; Chicago, USA).
Results
A total of 1,429 hospitalized COVID-19 pneumonia patients were available for selection, and 245 patients who met the inclusion criteria were randomly selected for the analysis, with the sample size determined based on a calculation to ensure adequate statistical power and generalizability of the results (Figure 1). The prevalence of early bacterial co-infection was 15.5% (38 patients). 48.2% of patients were men with a mean age of 60 years as shown in Table 1. There were more men in the Early bacterial co-infection group compared to the Unlikely early bacterial co-infection group (65.8% and 44.9%, respectively; P=0.018). The mean body mass index (BMI) was 26.6 kg/m2. Patients in the Early bacterial co-infection group had a higher Charlson Comorbidity Index (CCI) compared to the Unlikely early bacterial co-infection group (3.5 and 2, respectively; P=0.012). Patients with early bacterial co-infection had a significantly higher proportion of the comorbidities of chronic heart failure, coronary artery disease, and chronic kidney disease without renal replacement therapy.
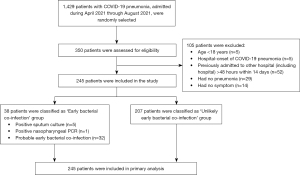
Table 1
Characteristics | Total (N=245) | Early bacterial co-infection (N=38) | Unlikely early bacterial co-infection (N=207) | P value | Odd ratio (95% CI) |
---|---|---|---|---|---|
Demographic data | |||||
Age (years), mean ± SD (range) | 60±17 (18 to 95) | 64±17 (32 to 95) | 60±17 (18 to 93) | 0.136 | 1.02 (0.995–1.04) |
Male, n (%) | 118 (48.2) | 25 (65.8) | 93 (44.9) | 0.018 | 0.42 (0.21–0.88) |
Body mass index, mean ± SD | 26.6±6.6 | 27.4±7.4 | 26.5±6.5 | 0.403 | 1.02 (0.97–1.07) |
Charlson Comorbidity Index, median (IQR) | 2 (1 to 4) | 3.5 (1.8 to 5.3) | 2 (1 to 4) | 0.012 | 1.2 (1.05–1.36) |
Smoking, n (%) | |||||
Non-smoker | 147 (87) | 20 (74.1) | 127 (89.4) | – | 1 |
Current smoker | 7 (4.1) | 2 (7.4) | 5 (3.5) | 0.284 | 2.54 (0.46–13.99) |
Ex-smoker | 15 (8.9) | 5 (18.5) | 10 (7) | 0.053 | 3.18 (0.98–10.26) |
Comorbidities, n (%) | |||||
Diabetes | 81 (33.1) | 14 (36.8) | 67 (32.4) | 0.59 | 1.22 (0.59–2.51) |
Hypertension | 125 (51.0) | 23 (60.5) | 102 (49.3) | 0.202 | 1.58 (0.78–3.20) |
Chronic heart failure | 5 (2.0) | 3 (7.9) | 2 (1.0) | 0.028 | 8.79 (1.42–54.48) |
Coronary artery disease | 22 (9.0) | 9 (23.7) | 13 (6.3) | 0.002 | 4.63 (1.82–11.80) |
Cerebrovascular accident | 12 (4.9) | 3 (7.9) | 9 (4.3) | 0.405 | 1.89 (0.49–7.31) |
Peripheral arterial disease | 2 (0.8) | 1 (2.6) | 1 (0.5) | 0.287 | 5.57 (0.34–90.99) |
Asthma | 5 (2.0) | 0 (0.0) | 5 (2.4) | 1 | – |
COPD | 7 (2.9) | 2 (5.3) | 5 (2.4) | 0.297 | 2.24 (0.42–12.02) |
Chronic kidney disease | |||||
Without RRT | 15 (6.1) | 6 (15.8) | 9 (4.3) | 0.013 | 4.07 (1.25–12.22) |
With RRT | 10 (4.1) | 1 (2.6) | 9 (4.3) | 0.716 | 0.68 (0.08–5.54) |
Cancer | 14 (5.7) | 1 (2.6) | 13 (6.3) | 0.702 | 0.40 (0.05–3.18) |
Hematologic malignancy | 3 (1.2) | 2 (5.3) | 1 (0.5) | 0.064 | 11.44 (1.01–129.53) |
Immunocompromised | 8 (3.3) | 2 (5.3) | 6 (2.9) | 1.86 (0.36–9.59) | |
HIV infection | 3 (1.2) | 1 (2.6) | 2 (1.0) | 0.398 | 2.77 (0.25–31.34) |
Biologic therapy | 1 (0.4) | 1 (2.6) | 0 (0.0) | 0.155 | – |
Chemotherapy within 6 months | 3 (1.2) | 0 (0.0) | 3 (1.4) | 1 | – |
SOT/HSCT | 1 (0.4) | 0 (0.0) | 1 (0.4) | 1 | – |
CI, confidence interval; COPD; chronic obstructive pulmonary disease; HIV, human immunodeficiency virus; HSCT, hematopoietic stem cell transplantation; IQR, interquartile range; RRT, renal replacement therapy; SD, standard deviation; SOT, solid organ transplantation.
The median days from symptom onset (DOS) to admission was 5 days which was no difference between groups. Fever, shortness of breath, and cough were the most presenting symptoms (70.6%, 55.1%, and 45.7%, respectively). In the Early bacterial co-infection group, shortness of breath (78.9%), initial intensive care unit (ICU) admission (28.9%), vasopressor use (10.5%), and a presence of at least 2 SIRS criteria (97.4%) were significantly higher than those in the Unlikely early bacterial co-infection group as shown in Table 2. There were more patients who needed high-flow nasal cannula (HFNC) or invasive mechanical ventilation (IMV) as the initial respiratory support in the Early bacterial co-infection group compared to the Unlikely early bacterial co-infection group (50% and 23.7%, and 16.4% and 2.4%, respectively; P<0.001).
Table 2
Variables | Total (N=245) | Early bacterial co-infection (N=38) | Unlikely early bacterial co-infection (N=207) | P value | Odd ratio (95% CI) |
---|---|---|---|---|---|
Days from symptoms onset to admission | 5 (3 to 7) | 5 (3 to 7.25) | 5 (3 to 7) | 0.716 | 1.04 (0.92–1.17) |
Symptoms at admission | |||||
Fever | 173 (70.6) | 28 (73.7) | 145 (70.0) | 0.651 | 1.20 (0.55–2.61) |
Shortness of breath | 135 (55.1) | 30 (78.9) | 105 (50.7) | 0.001 | 3.64 (1.60–8.32) |
Cough | 112 (45.7) | 18 (47.4) | 94 (45.4) | 0.824 | 1.08 (0.54–2.16) |
Diarrhea | 31 (12.7) | 6 (15.8) | 25 (12.1) | 0.594 | 1.37 (0.52–3.59) |
Mode of respiratory support in the first 48 hours | |||||
No supplement oxygen | 94 (38.4) | 3 (7.9) | 91 (44.0) | – | 1 |
Low-flow oxygen | 83 (33.9) | 7 (18.4) | 76 (36.7) | 0.146 | 2.79 (0.70–11.18) |
HFNC | 54 (21.6) | 19 (50) | 35 (16.4) | <0.001 | 16.47 (4.59–59.14) |
IMV | 14 (5.7) | 9 (23.7) | 5 (2.4) | <0.001 | 54.60 (11.17–266.88) |
Initial ICU admission within 48 hours after admission | 20 (8.2) | 11 (28.9) | 9 (4.3) | <0.001 | 8.96 (3.40–23.61) |
Vasopressor use | 6 (2.4) | 4 (10.5) | 2 (1.0) | 0.006 | 12.06 (2.13–68.41) |
≥2 SIRS criteria | 91 (37.1) | 37 (97.4) | 54 (26.1) | <0.001 | 104.83 (14.04–782.68) |
Fever >38 ℃ or <36 ℃ | 45 (18.4) | 14 (36.8) | 31 (12.7) | 0.001 | 3.31 (1.55–7.09) |
Heart rate >90 bpm | 99 (40.4) | 28 (73.7) | 71 (33.8) | <0.001 | 5.36 (2.47–11.67) |
RR >20/min or PaO2 <38 mmHg | 107 (43.7) | 34 (89.5) | 73 (35.3) | <0.001 | 15.60 (5.33–45.70) |
WBC >12,000 or <4,000×109/L or immature neutrophil >10% | 64 (26.1) | 20 (52.6) | 44 (21.3) | <0.001 | 4.12 (2.01–8.44) |
Radiographic findings | |||||
Findings | |||||
Characteristic | 92 (37.6) | 9 (23.7) | 83 (40.1) | 0.055 | 0.46 (0.21–1.03) |
Non-specific | |||||
Focal, unilateral | 13 (5.3) | 1 (2.6) | 12 (5.8) | 0.698 | 0.44 (0.06–3.48) |
Ill-defined bibasilar opacities | 72 (29.4) | 7 (18.4) | 65 (31.4) | 0.106 | 0.49 (0.21–1.18) |
Diffuse opacities | 51 (20.8) | 17 (44.7) | 34 (16.5) | <0.001 | 4.12 (1.97–8.61) |
Upper lobe predominant opacities | 7 (2.9) | 0 (0.0) | 7 (3.4) | 0.600 | – |
Mass-like opacities | 8 (3.3) | 4 (10.5) | 4 (1.9) | 0.022 | 5.97 (1.43–25.02) |
Effusion | 2 (0.8) | 0 (0.0) | 2 (1.0) | 1.000 | – |
Brixia chest X-ray score | 8 (5 to 11) | 11 (8 to 15) | 7 (4 to 11) | <0.001 | 1.24 (1.13–1.35) |
Laboratory testing | |||||
Hemoglobin (g/dL) | 12.8±2 | 12.6±2 | 12.8±2 | 0.436 | 0.93 (0.79–1.11) |
White blood cell count (×109/L) | 6,524.2±3,010.2 | 8,720.3±5,153.1 | 6,121±2,216 | 0.004 | 1.0002 (1.0001–1.0004) |
Absolute neutrophil count (×109/L) | 4,060 (2,980 to 5,805) | 5,955 (3,980 to 10,212.5) | 3,970 (2,910 to 5,490) | <0.001 | 1.0003 (1.0002–1.0004) |
D-dimer (ng/mL) | 869 (503 to 1,596) | 1,015 (635 to 3,127.5) | 863 (489.3 to 1,539.5) | 0.025 | 1.0001 (1.00001–1.0002) |
C-reactive protein (mg/dL) | 49.7 (15.7 to 88.1) | 83.9 (58 to 126.4) | 41.8 (9.8 to 79.2) | <0.001 | 1.01 (1.005–1.02) |
Procalcitonin (ng/mL) | 0.22 (0.09 to 0.54) | 0.43 (0.19 to 1.58) | 0.17 (0.08 to 0.36) | 0.115 | 1.05 (0.99–1.12) |
Data are presented as median (IQR) or n (%) or mean ± SD. bpm, beats per minute; CI, confidence interval; g/dL, grams per deciliter; HFNC, high-flow nasal cannula; ICU, intensive care unit; IQR, interquartile range; IMV, invasive mechanical ventilation; L, liters; mg/dL, milligrams per deciliter; ng/mL, nanograms per milliliter; PaO2, partial pressure of oxygen in arterial blood; RR, respiratory rate; SIRS, systemic inflammatory response syndrome; SD, standard deviation; WBC, white blood cells.
All patients performed chest X-rays within 48 hours of admission. 92 of 245 patients (37.6%) had characteristic radiographic findings for COVID-19 pneumonia which included bilateral patchy, bandlike ground-glass opacities or consolidation in peripheral and mid to lower lung zone (23.7% in the Early bacterial co-infection group and 40.1% in the Unlikely early bacterial co-infection group, P=0.055) as shown in Table 2. Interobserver agreement for radiographic interpretation was moderate (Kappa coefficient 0.603, P<0.001). Diffuse opacities and mass-liked opacities were found more in the Early bacterial co-infection group compared to the Unlikely early bacterial co-infection group (44.7% and 16.5%, and 10.5% and 1.9%, respectively; P<0.001). The median Brixia chest X-ray scores were 11 and 7 in the Early bacterial co-infection group and the Unlikely early bacterial co-infection group, respectively (P<0.001), with an intraclass correlation coefficient between two readers of 0.77 (95% CI: 0.71–0.82).
Compared to the Unlikely early bacterial co-infection group, patients in the Early bacterial co-infection group had a significantly higher white blood cell count, absolute neutrophil count, D-dimer levels, and C-reactive protein (CRP) levels, whereas the procalcitonin (PCT) level was no difference between groups as shown in Table 2.
There was no difference in the number of patients with worsening respiratory failure, length of hospital stays, length of ICU stays, and days of mechanical ventilation between groups. Higher all-cause mortality was observed in the Early bacterial co-infection group compared to the Unlikely early bacterial co-infection group (31.6% and 12.6%, respectively; P=0.012). Complications including pneumothorax, pneumomediastinum, ventilator-associated pneumonia, hospital-acquired pneumonia, and other nosocomial infection were no significant differences between groups as shown in Table 3.
Table 3
Variables | Total (N=245) | Early bacterial co-infection (N=38) | Unlikely early bacterial co-infection (N=207) | P value | Odd ratio (95% CI) |
---|---|---|---|---|---|
Hospital course | |||||
Worsening respiratory failure, n (%) | 73 (29.8) | 13 (34.2) | 60 (29.0) | 0.517 | 1.27 (0.61–2.66) |
Length of hospital stays (days), median (IQR) | 9 (5 to 14) | 12.5 (8 to 16) | 9 (5 to 14) | 0.078 | 1.03 (0.997–1.07) |
Length of ICU stays (days), median (min to max) | 9 (5 to 15) | 9 (5 to 17) | 9 (5 to 15) | 0.697 | 1.02 (0.96–1.09) |
Days of mechanical ventilation (days), median (range) | 9 (7 to 19.5) | 13 (7.5 to 26.5) | 8.5 (5.5 to 18.8) | 0.472 | 1.02 (0.97–1.08) |
Outcome, n (%) | |||||
Discharge | 170 (69.4) | 24 (63.2) | 146 (70.5) | – | 1 |
Refer to other hospital | 37 (15.1) | 2 (5.3) | 35 (16.9) | 0.164 | 0.35 (0.08–1.54) |
Death | 38 (15.5) | 12 (31.6) | 26 (12.6) | 0.012 | 2.81 (1.25–6.30) |
Respiratory cause | 35 (14.3) | 11 (28.9) | 24 (11.6) | ||
Other cause | 3 (1.2) | 1 (2.6) | 2 (1.0) | ||
Complications, n (%) | |||||
Pneumothorax | 5 (2.0) | 1 (2.6) | 4 (1.9) | 0.573 | 1.37 (0.15–12.62) |
Pneumomediastinum | 5 (2.0) | 1 (2.6) | 4 (1.9) | 0.573 | 1.37 (0.15–12.62) |
VAP/HAP | 24 (9.8) | 4 (10.5) | 20 (9.7) | 0.773 | 1.10 (0.35–3.42) |
Nosocomial infection other than LRTI | 0.918 | ||||
Urinary tract infection | 5 (2.0) | 1 (2.6) | 4 (1.9) | ||
Catheter-related blood stream infection | 2 (0.8) | 0 (0.0) | 2 (0.8) | ||
Bacteremia | 7 (2.9) | 2 (5.3) | 5 (2.4) | ||
CMV colitis | 1 (0.4) | 0 (0.0) | 1 (0.5) |
CI, confidence interval; CMV, cytomegalovirus, HAP, hospital-acquired pneumonia; ICU, intensive care unit; IQR, interquartile range; LRTI, lower respiratory tract infection; VAP, ventilator-associated pneumonia.
The microbiological data were available in 62 of 245 patients (25.3%) including 62 hemocultures (25.3%), 27 sputum or tracheal aspirate cultures (11%), 5 nasopharyngeal PCR (2%), and 4 sputum PCR (1.6%). In the Early bacterial co-infection group, 6 patients (15.8%) had microbiological confirmation, and 32 patients (84.2%) met the criteria for probable bacterial co-infection. The initial empirical antibiotics were prescribed in 25.3% (97.4% in the Early bacterial co-infection group and 12.1% in the Unlikely early bacterial co-infection group; P<0.001). The causative pathogens included methicillin-susceptible Staphylococcus aureus (n=2), Pseudomonas aeruginosa (n=2), and Stenotrophomonas maltophilia (n=2), Haemophilus influenza (n=1), Klebsiella pneumoniae (n=1), and Enterobacter cloacae (n=1).
When using the Brixia chest X-ray score cut-off at 8, patients with scores of more than 8 had more IMV use (20.2% and 5.1%, P<0.001) and ICU admission (24.8% and 6.6%, P<0.001). This group also had higher mortality when compared to the group with a lower Brixia chest X-ray score (22% and 10.3%, respectively; P=0.049) (Table 4).
Table 4
Variables | Brixia chest X-ray score | CRP level (mg/dL) | PCT level (ng/mL) | |||||||||||
---|---|---|---|---|---|---|---|---|---|---|---|---|---|---|
>8 (N=109) | <8 (N=136) | P value | Odd ratio (95% CI) | ≥60 mg/dL (N=107) | <60 mg/dL (N=138) | P value | Odd ratio (95% CI) | >0.5 ng/mL (N=37) | <0.5 ng/mL (N=109) | P value | Odd ratio (95% CI) | |||
Worsening respiratory failure | 31 (28.4) | 42 (30.9) | 0.678 | 0.89 (0.51–1.55) | 34 (31.8) | 39 (28.3) | 0.551 | 1.18 (0.68–2.05) | 11 (29.7) | 31 (28.4) | 0.881 | 1.07 (0.47–2.41) | ||
Length of hospital stays (days) | 10 (5 to 14) | 9 (5 to 3) | 0.211 | 1.02 (0.99–1.05) | 10 (6 to 14) | 8 (5 to 14) | 0.07 | 1.02 (0.99–1.05) | 12 (7 to 15) | 9 (6 to 14) | 0.257 | 1.01 (0.97–1.05) | ||
Any invasive mechanical ventilator use during hospital course | 22 (20.2) | 7 (5.1) | <0.001 | 4.66 (1.91–11.38) | 19 (17.8) | 10 (7.2) | 0.012 | 2.76 (1.23–6.23) | 5 (13.5) | 15 (13.8) | 0.97 | 0.98 (0.33–2.91) | ||
Any ICU admission during hospital course | 27 (24.8) | 9 (6.6) | <0.001 | 4.65 (2.08–10.38) | 20 (18.7) | 16 (11.6) | 0.12 | 1.75 (0.86–3.58) | 8 (21.6) | 16 (14.7) | 0.325 | 1.6 (0.62–4.13) | ||
Outcome | ||||||||||||||
Discharge | 77 (70.6) | 93 (68.4) | – | 1 | 76 (71.0) | 94 (68.1) | – | 1 | 27 (73.0) | 85 (78.0) | – | 1 | ||
Refer to other hospital | 8 (7.3) | 29 (21.3) | 0.01 | 0.33 (0.14–0.77) | 7 (6.5) | 30 (21.7) | 0.005 | 0.29 (0.12–0.69) | 0 (0.0) | 5 (4.6) | 1 | – | ||
Death | 24 (22.0) | 14 (10.3) | 0.049 | 2.07 (1.003–4.28) | 24 (22.4) | 14 (10.1) | 0.042 | 2.12 (1.03–4.38) | 10 (27.0) | 19 (17.5) | 0.261 | 1.66 (0.69–4.00) |
Data are presented as median (IQR) or n (%). CI, confidence interval; CRP, C-reactive protein; ICU, intensive care unit; IQR, interquartile range; mg/dL, milligrams per deciliter; ng/mL, nanograms per milliliter; PCT, procalcitonin.
When using the CRP level cut-off at 60 mg/dL, patients with CRP levels of at least 60 mg/dL had more IMV use (17.8% and 7.2%, P=0.012). Higher mortality was also observed in this group compared to those with lower CRP levels as shown in Table 4.
When using the PCT level cut-off at 0.5 ng/mL, there was no significant difference in the number of patients with worsening respiratory failure, length of hospital stays, invasive mechanical ventilator use, ICU admission, and all-cause mortality.
Univariate and multivariate analyses
All of the potential risk factors for early bacterial co-infection were assessed using univariate and multivariate analyses as shown in Table 5. The analysis demonstrated that the CCI >4 (OR 2.99, 95% CI: 1.24–7.19, P=0.014), the use of HFNC (OR 22.21, 95% CI: 4.69–105.13, P<0.001), the use of IMV (OR 63.69, 95% CI: 9.9–413.06, P=0.002), diffuse opacities on chest X-ray (OR 3.23, 95% CI: 1.31–7.97, P=0.011), and mass-like opacities on chest X-ray (OR 21.31, 95% CI: 2.47–183.85, P=0.005) were independent factors associated with early bacterial co-infection.
Table 5
Factors | Univariate analysis | Multivariate analysis | |||
---|---|---|---|---|---|
Odd ratio (95% CI) | P value | Odd ratio (95% CI) | P value | ||
Male | 0.42 (0.21–0.88) | 0.018 | |||
Charlson Comorbidity Index ≥4 | 2.39 (1.19–4.83) | 0.013 | 2.99 (1.24–7.19) | 0.014 | |
Coronary artery disease | 4.63 (1.82–11.80) | 0.002 | |||
Chronic kidney disease, without RRT | 4.07 (1.25–12.22) | 0.013 | |||
Shortness of breath | 3.64 (1.60–8.32) | 0.001 | |||
Mode of respiratory support in the first 48 hours | |||||
HFNC | 16.47 (4.59–59.14) | <0.001 | 22.21 (4.69–105.13) | <0.001 | |
IMV | 54.60 (11.17–266.88) | <0.001 | 63.96 (9.9–413.06) | 0.002 | |
Initial ICU admission | 8.96 (3.40–23.61) | <0.001 | |||
Vasopressors use | 12.06 (2.13–68.41) | 0.006 | |||
Chest X-ray findings, n (%) | |||||
Diffuse opacities | 4.12 (1.97–8.61) | <0.001 | 3.23 (1.31–7.97) | 0.011 | |
Mass-like opacities | 5.97 (1.43–25.02) | 0.022 | 21.31 (2.47–183.85) | 0.005 | |
Brixia chest X-ray score >8 | 4.36 (2.01–9.40) | <0.001 | |||
CRP level ≥60 mg/dL | 5.30 (2.4–11.85) | <0.001 |
CI, confidence interval; CRP, C-reactive protein; HFNC, high-flow nasal cannula; ICU, intensive care unit; IMV, invasive mechanical ventilation; mg/dL, milligrams per deciliter; RRT, renal replacement therapy.
Discussion
The prevalence of early bacterial co-infection in COVID-19 pneumonia widely varies depending on the patient population and diagnostic criteria, ranging from 0–46% (2,22-25). The microbiological tests in the present study were infrequently performed which was similar to the previous report that might reflect the real-world situation (1). The present study reported a prevalence of 15.5% which included the patients who had a microbiological confirmation and patients with probable early bacterial co-infection. In the pandemic situation, the collection of respiratory specimens cannot be performed in all patients because of the risk of the virus spreading to healthcare providers and other patients. Therefore, the use of clinical data combining with chest X-ray features and other laboratory tests might be helpful. The present study demonstrated that higher CCI, the use of a high level of respiratory support, diffuse opacities, and mass-liked opacities on chest X-rays were independent factors associated with early bacterial co-infection consistently with previous reports (8,9,22,26,27). A large multicenter study identified the characteristics of patients at risk for developing early bacterial co-infection, comprising of higher targeted real-time early warning score, higher CRP level, and higher ferritin level. Moreover, it also showed that the high-grade fever, purulent sputum, leukocytosis, need for supplementary oxygen, and specific chest radiographic findings including consolidation, infiltration, and interstitial opacities, were found more in patients with early bacterial co-infection (8). Another observation cohort study found that combining the white blood cell count of more than 8.8×109 cells/L, absolute neutrophil count of more than 6.9×109 cells/L, and CRP level of more than 119.8 mg/dL can predict the early bacterial co-infection with very high negative predictive values (9).
Chest X-ray is commonly used and plays an important role in monitoring COVID-19 pneumonia patients to assess the severity and extent of lung involvement, identify complications, and treatment guidance (28). The characteristic chest X-ray finding, including bilateral patchy and/or confluent, bandlike ground-glass opacity or consolidation, peripheral and mid to lower lung zone distribution, was more likely to be found in the Unlikely early bacterial co-infection group. The present study confirmed the usefulness of chest X-ray in determining the severity and monitoring the disease. The presence of early bacterial co-infection should be considered in patients who had diffuse or mass-liked opacities on chest X-ray and Brixia chest X-ray score of more than 8. However, these radiological features can overlap and cannot be totally distinguished from COVID-19 pneumonia, combining with the clinical data and other laboratory tests would be necessary to consider microbiological tests to confirm the presence of early bacterial co-infection. The previous study has reported the severity scoring system of chest X-ray, the Brixia chest X-ray score, which the higher score was associated with mortality in COVID-19 pneumonia patients (20). Consistently, we found that the Brixia chest X-ray score of more than 8 was associated with more severe disease and higher mortality.
Several studies found that the elevation of CRP and PCT levels were associated with worse clinical outcomes (29-34). Data on the use of these biomarkers to predict early bacterial co-infection in COVID-19 pneumonia is limited. The present study found that early bacterial co-infection was associated with higher CRP levels but there was no correlation with PCT levels. Consistently with previous studies, baseline PCT level should be interpreted with caution to exclude early bacterial co-infection but a rising of PCT level might be useful in the detection of acquired bacterial infection during hospitalization (35).
We did not perform microbiological tests in all patients because of the followings: (I) The present study was a retrospective study. We did not have a routine protocol for collecting respiratory specimens in all patients with COVID-19 pneumonia, and (II) there was a limitation in respiratory specimen collection due to the risk of spreading the virus to healthcare providers and other patients. These appear to be real-life situations in the pandemic era in which the rate of respiratory specimen collection was low (1). The most common causative pathogens included S.aureus, H.influenzae, and S.pneumoniae while gram-negative organisms were more reported in nosocomial infection (3,11,36). However, gram-negative organisms have also been reported as the causative pathogens in early bacterial co-infection with variable prevalence, including K.pneumoniae (3.4%), P.aeruginosa (9.3%), and E.coli (7.6%) (1,26). In the present study, two patients had S.maltophilia mixed with K.pneumoniae and MSSA on their sputum cultures. Therefore, the authors included these patients in the early bacterial co-infection group.
In the present study, the initial empirical antibiotics were prescribed in 25.3% which was lower than that in the previous studies (37,38). However, the role of empirical antibiotics in COVID-19 pneumonia patients is controversial. There have been studies demonstrating that inappropriate use of antibiotics may lead to increased morbidity and mortality in patients with COVID-19 (37-39). Furthermore, overuse of antibiotics results in antibiotic-related side effects and the development of resistant nosocomial bacterial and fungal pathogens. Despite the low reported prevalence of bacterial co-infection, one study showed that more than half of hospitalized COVID-19-infected patients were empirically treated with antibiotics at hospital admission. Most of these patients were elderly, had severe disease symptoms, had lobar infiltrates from chest X-rays, or were admitted to the for-profit hospital (37). The National Institutes of Health (NIH) guideline stated that there was insufficient evidence to give any recommendation either for or against empirical antibiotics in the absence of another indication. The recent surviving sepsis campaign guideline recommended prescribing empirical antibiotics only in patients with respiratory failure requiring mechanical ventilation (40). According to the results of the present study, the initial empirical antibiotics may be considered in COVID-19 pneumonia patients with higher comorbidities, diffuse or mass-liked opacities on chest X-ray, and receiving a high level of respiratory support while waiting for confirmation from microbiological tests.
The present study has some limitations. Firstly, it is a single-center, retrospective cross-sectional study. Some data collection might be limited. Secondly, we did not routinely perform microbiological tests in all patients. These might result in underdiagnosis of bacterial co-infection if microbiological confirmation is the only criteria for diagnosis. Therefore, we included the patient with probable bacterial co-infection by carefully reviewing the criteria of categorization to minimize bias.
Conclusions
The prevalence of early bacterial co-infection in hospitalized patients with COVID-19 pneumonia was low. There is insufficient evidence to support the empirical use of antibiotics in patients with COVID-19 pneumonia. A further prospective study is required to confirm the results of the present study.
Acknowledgments
Funding: None.
Footnote
Reporting Checklist: The authors have completed the STROBE reporting checklist. Available at https://jtd.amegroups.com/article/view/10.21037/jtd-22-1681/rc
Data Sharing Statement: Available at https://jtd.amegroups.com/article/view/10.21037/jtd-22-1681/dss
Peer Review File: Available at https://jtd.amegroups.com/article/view/10.21037/jtd-22-1681/prf
Conflicts of Interest: All authors have completed the ICMJE uniform disclosure form (available at https://jtd.amegroups.com/article/view/10.21037/jtd-22-1681/coif). The authors have no conflicts of interest to declare.
Ethical Statement: The authors are accountable for all aspects of the work in ensuring that questions related to the accuracy or integrity of any part of the work are appropriately investigated and resolved. The study was conducted in accordance with the Declaration of Helsinki (as revised in 2013) and approved by the Institutional Review Board of the Faculty of Medicine Siriraj Hospital (COA No. SI901/2564). Informed consent was waived due to the retrospective nature of the study.
Open Access Statement: This is an Open Access article distributed in accordance with the Creative Commons Attribution-NonCommercial-NoDerivs 4.0 International License (CC BY-NC-ND 4.0), which permits the non-commercial replication and distribution of the article with the strict proviso that no changes or edits are made and the original work is properly cited (including links to both the formal publication through the relevant DOI and the license). See: https://creativecommons.org/licenses/by-nc-nd/4.0/.
References
- Russell CD, Fairfield CJ, Drake TM, et al. Co-infections, secondary infections, and antimicrobial use in patients hospitalised with COVID-19 during the first pandemic wave from the ISARIC WHO CCP-UK study: a multicentre, prospective cohort study. Lancet Microbe 2021;2:e354-65. [Crossref] [PubMed]
- Lansbury L, Lim B, Baskaran V, et al. Co-infections in people with COVID-19: a systematic review and meta-analysis. J Infect 2020;81:266-75. [Crossref] [PubMed]
- Paparoupa M, Aldemyati R, Roggenkamp H, et al. The prevalence of early- and late-onset bacterial, viral, and fungal respiratory superinfections in invasively ventilated COVID-19 patients. J Med Virol 2022;94:1920-5. [Crossref] [PubMed]
- Klein EY, Monteforte B, Gupta A, et al. The frequency of influenza and bacterial coinfection: a systematic review and meta-analysis. Influenza Other Respir Viruses 2016;10:394-403. [Crossref] [PubMed]
- Johansson N, Kalin M, Tiveljung-Lindell A, et al. Etiology of community-acquired pneumonia: increased microbiological yield with new diagnostic methods. Clin Infect Dis 2010;50:202-9. [Crossref] [PubMed]
- Choi SH, Hong SB, Ko GB, et al. Viral infection in patients with severe pneumonia requiring intensive care unit admission. Am J Respir Crit Care Med 2012;186:325-32. [Crossref] [PubMed]
- Cuquemelle E, Soulis F, Villers D, et al. Can procalcitonin help identify associated bacterial infection in patients with severe influenza pneumonia? A multicentre study. Intensive Care Med 2011;37:796-800. [Crossref] [PubMed]
- Karaba SM, Jones G, Helsel T, et al. Prevalence of Co-infection at the Time of Hospital Admission in COVID-19 Patients, A Multicenter Study. Open Forum Infect Dis 2021;8:ofaa578. [Crossref] [PubMed]
- Wang L, Amin AK, Khanna P, et al. An observational cohort study of bacterial co-infection and implications for empirical antibiotic therapy in patients presenting with COVID-19 to hospitals in North West London. J Antimicrob Chemother 2021;76:796-803. [Crossref] [PubMed]
- Baskaran V, Lawrence H, Lansbury LE, et al. Co-infection in critically ill patients with COVID-19: an observational cohort study from England. J Med Microbiol 2021;70:001350. [Crossref] [PubMed]
- Contou D, Claudinon A, Pajot O, et al. Bacterial and viral co-infections in patients with severe SARS-CoV-2 pneumonia admitted to a French ICU. Ann Intensive Care 2020;10:119. [Crossref] [PubMed]
- Garcia-Vidal C, Sanjuan G, Moreno-García E, et al. Incidence of co-infections and superinfections in hospitalized patients with COVID-19: a retrospective cohort study. Clin Microbiol Infect 2021;27:83-8. [Crossref] [PubMed]
- Chen S, Zhu Q, Xiao Y, et al. Clinical and etiological analysis of co-infections and secondary infections in COVID-19 patients: An observational study. Clin Respir J 2021;15:815-25. [Crossref] [PubMed]
- Hedberg P, Johansson N, Ternhag A, et al. Bacterial co-infections in community-acquired pneumonia caused by SARS-CoV-2, influenza virus and respiratory syncytial virus. BMC Infect Dis 2022;22:108. [Crossref] [PubMed]
- Musuuza JS, Watson L, Parmasad V, et al. Prevalence and outcomes of co-infection and superinfection with SARS-CoV-2 and other pathogens: A systematic review and meta-analysis. PLoS One 2021;16:e0251170. [Crossref] [PubMed]
- He S, Liu W, Jiang M, et al. Clinical characteristics of COVID-19 patients with clinically diagnosed bacterial co-infection: A multi-center study. PLoS One 2021;16:e0249668. [Crossref] [PubMed]
- Coenen S, de la Court JR, Buis DTP, et al. Low frequency of community-acquired bacterial co-infection in patients hospitalized for COVID-19 based on clinical, radiological and microbiological criteria: a retrospective cohort study. Antimicrob Resist Infect Control 2021;10:155. [Crossref] [PubMed]
- Smith DL, Grenier JP, Batte C, et al. A Characteristic Chest Radiographic Pattern in the Setting of the COVID-19 Pandemic. Radiol Cardiothorac Imaging 2020;2:e200280. [Crossref] [PubMed]
- Wong HYF, Lam HYS, Fong AH, et al. Frequency and Distribution of Chest Radiographic Findings in Patients Positive for COVID-19. Radiology 2020;296:E72-8. [Crossref] [PubMed]
- Borghesi A, Maroldi R. COVID-19 outbreak in Italy: experimental chest X-ray scoring system for quantifying and monitoring disease progression. Radiol Med 2020;125:509-13. [Crossref] [PubMed]
- Sharma N, Putman MS, Vij R, et al. Myositis-associated Interstitial Lung Disease: Predictors of Failure of Conventional Treatment and Response to Tacrolimus in a US Cohort. J Rheumatol 2017;44:1612-8. [Crossref] [PubMed]
- Langford BJ, So M, Raybardhan S, et al. Bacterial co-infection and secondary infection in patients with COVID-19: a living rapid review and meta-analysis. Clin Microbiol Infect 2020;26:1622-9. [Crossref] [PubMed]
- Santos AP, Gonçalves LC, Oliveira ACC, et al. Bacterial Co-Infection in Patients with COVID-19 Hospitalized (ICU and Not ICU): Review and Meta-Analysis. Antibiotics (Basel) 2022;11:894. [Crossref] [PubMed]
- Soltani S, Faramarzi S, Zandi M, et al. Bacterial coinfection among coronavirus disease 2019 patient groups: an updated systematic review and meta-analysis. New Microbes New Infect 2021;43:100910. [Crossref] [PubMed]
- Adler H, Ball R, Fisher M, et al. Low rate of bacterial co-infection in patients with COVID-19. Lancet Microbe 2020;1:e62. [Crossref] [PubMed]
- Elabbadi A, Turpin M, Gerotziafas GT, et al. Bacterial coinfection in critically ill COVID-19 patients with severe pneumonia. Infection 2021;49:559-62. [Crossref] [PubMed]
- Shafran N, Shafran I, Ben-Zvi H, et al. Secondary bacterial infection in COVID-19 patients is a stronger predictor for death compared to influenza patients. Sci Rep 2021;11:12703. [Crossref] [PubMed]
- Brandi N, Ciccarese F, Rimondi MR, et al. An Imaging Overview of COVID-19 ARDS in ICU Patients and Its Complications: A Pictorial Review. Diagnostics (Basel) 2022;12:846. [Crossref] [PubMed]
- Heesom L, Rehnberg L, Nasim-Mohi M, et al. Procalcitonin as an antibiotic stewardship tool in COVID-19 patients in the intensive care unit. J Glob Antimicrob Resist 2020;22:782-4. [Crossref] [PubMed]
- Aon M, Alsaeedi A, Alzafiri A, et al. The Association between Admission Procalcitonin Level and The Severity of COVID-19 Pneumonia: A Retrospective Cohort Study. Medicina (Kaunas) 2022;58:1389. [Crossref] [PubMed]
- Lippi G, Plebani M. Procalcitonin in patients with severe coronavirus disease 2019 (COVID-19): A meta-analysis. Clin Chim Acta 2020;505:190-1. [Crossref] [PubMed]
- Villoteau A, Asfar M, Otekpo M, et al. Elevated C-reactive protein in early COVID-19 predicts worse survival among hospitalized geriatric patients. PLoS One 2021;16:e0256931. [Crossref] [PubMed]
- Potempa LA, Rajab IM, Hart PC, et al. Insights into the Use of C-Reactive Protein as a Diagnostic Index of Disease Severity in COVID-19 Infections. Am J Trop Med Hyg 2020;103:561-3. [Crossref] [PubMed]
- Alzahrani SH, Al-Rabia MW. Cardiac Injury Biomarkers and the Risk of Death in Patients with COVID-19: A Systematic Review and Meta-Analysis. Cardiol Res Pract 2021;2021:9363569. [Crossref] [PubMed]
- Atallah NJ, Warren HM, Roberts MB, et al. Baseline procalcitonin as a predictor of bacterial infection and clinical outcomes in COVID-19: A case-control study. PLoS One 2022;17:e0262342. [Crossref] [PubMed]
- Zhang G, Hu C, Luo L, et al. Clinical features and short-term outcomes of 221 patients with COVID-19 in Wuhan, China. J Clin Virol 2020;127:104364. [Crossref] [PubMed]
- Vaughn VM, Gandhi TN, Petty LA, et al. Empiric Antibacterial Therapy and Community-onset Bacterial Coinfection in Patients Hospitalized With Coronavirus Disease 2019 (COVID-19): A Multi-hospital Cohort Study. Clin Infect Dis 2021;72:e533-41. [Crossref] [PubMed]
- Langford BJ, So M, Raybardhan S, et al. Antibiotic prescribing in patients with COVID-19: rapid review and meta-analysis. Clin Microbiol Infect 2021;27:520-31. [Crossref] [PubMed]
- Rawson TM, Moore LSP, Zhu N, et al. Bacterial and Fungal Coinfection in Individuals With Coronavirus: A Rapid Review To Support COVID-19 Antimicrobial Prescribing. Clin Infect Dis 2020;71:2459-68. [Crossref] [PubMed]
- Alhazzani W, Møller MH, Arabi YM, et al. Surviving Sepsis Campaign: Guidelines on the Management of Critically Ill Adults with Coronavirus Disease 2019 (COVID-19). Crit Care Med 2020;48:e440-69. [Crossref] [PubMed]