Fusu mixture alleviates inflammatory reactions in lipopolysaccharide-induced acute respiratory distress syndrome mice via regulation of surfactant-associated protein C, aquaporin 5, and Notch1
Highlight box
Key findings
• It is suggested that Fusu mixture (FSM) alleviates inflammatory reactions and promotes the proliferation of alveolar epithelial cells in LPS-induced ARDS mice via regulation of SP-C, AQP-5, and Notch1 in lung tissues.
What is known and what is new?
• FSM can improve the mechanical ventilation in ARDS patients.
• Our findings contribute the detailed pharmacological mechanisms and active substances of FSM in LPS-induced ARDS mice.
What is the implication, and what should change now?
• It provides evidence that FSM is an effective treatment for treating ARDS and other respiratory diseases, providing guidance for clinical medication. Future works might be devoted to the investigation of the activities of these monomers in FSM on ARDS and the related mechanisms.
Introduction
Acute respiratory distress syndrome (ARDS), characterized by intractable hypoxia and expiratory dyspnea, is a common life-threatening critical illness in with high mortality (1,2). Importantly, new emerging evidences have revealed that ARDS has a very high morbidity in coronavirus disease of 2019 (COVID-19)-induced respiratory tract infection, which is also a predominant cause for the poor prognosis of patients with severe COVID-19 pneumonia (3-5). The detailed pathogenesis for ARDS is extremely complex and remains uncovered, and the results of previous research showed that ARDS might be closely correlated to lung injury induced by inflammatory cytokines released from several inflammatory cells (6,7). Currently, the available treatment for ARDS predominantly comprises supportive treatment including lung protective ventilation, restricted liquid management, and extracorporeal membrane oxygenation (ECMO). Taraxasterol (8), vitamin D (9), and fraxin (10) may help alleviate ARDS by downregulating inflammatory responses. However, there is a lack of evidence or effective drugs to ameliorate ARDS in the clinical setting. Consequently, there is an urgent need to discover more reliable candidate drugs for the treatment of ARDS (11,12). Natural herbal medicines, particularly traditional Chinese medicines (TCMs), are irrefutably precious resources for the exploration of new drugs for treating various intractable diseases (13,14). Increasing evidences have shown that TCMs could be used to improve COVID-19 pneumonia, and could be also effective to treat ARDS induced by various viruses or bacteria (15-17).
Fusu mixture (FSM) is an effective TCM formula for treating ARDS or septic shock in the clinic that is derived from the ancient prescriptions of Qian-yang-dan recorded in a TCM monograph of Yilizhenchuan written in the Qing dynasty (18,19). FSM comprises 7 herbal medicines, including Aconiti Lateralis Radix Praeparata (Fuzi; 30 g), Amomi Fructus (Sha Ren; 15 g), Testudinis Carapax et Plastrum (Gui Jia; 30 g), Ephedrae Herba (Ma Huang; 10 g), Zingiberis Rhizoma (Gan Jiang; 10 g), and Glycyrrhizae Radix et Rhizoma (Gan Cao; 12 g). Our previous clinical research results suggested that FSM can improve the mechanical ventilation in ARDS patients via increase of the arterial partial pressure of oxygen (PaO2) and oxygenation index [PaO2/fraction of inspired oxygen (FiO2)] (18,19). In the previous clinical practice, we found that the TCM FSM could supplement the basic treatment of Western medicine, clinically reduce the extravascular lung water index of patients with sepsis-induced ARDS, and improve the prognosis of patients (19). However, the detailed pharmacological mechanisms and active substances of FSM are still unclear. So, in our present study, we established an ARDS animal model to explore the potential pharmacological mechanisms of FSM for treating ARDS, and we also further explored the chemical compositions of FSM analyzed by high performance liquid chromatography (HPLC). We present this article in accordance with the ARRIVE reporting checklist (available at https://jtd.amegroups.com/article/view/10.21037/jtd-23-367/rc).
Methods
Chemicals and reagents
Lipopolysaccharide (LPS), hematoxylin and eosin (H&E) staining, and pentobarbital sodium were purchased from Sigma-Aldrich (Shanghai, China); enzyme-linked immunosorbent assay (ELISA) kits for interleukin-6 (IL-6) and tumor necrosis factor-α (TNF-α), and horseradish peroxidase (HRP)-conjugated secondary antibodies were purchased from Boster Biotech (Wuhan, China); primary antibodies of surfactant-associated protein C (SP-C), aquaporin 5 (AQP-5), and Notch1 were purchased from Thermo Fisher Inc. (Shanghai, China).
Preparation of water extracts of FSM
All the 7 herbal medicines, obtained from the Chinese Pharmacy of our hospital, were powdered and soaked in purified water for 30 minutes. Then, all the herbal medicines were decocted for 1.5 hours. The extracts were subsequently filtrated and concentrated at 50 ℃ using a rotary evaporator in vacuum to afford the water extracts of FSM.
Animal model and grouping
A total of 30 mice (20±2 g), purchased from the Dossy Experimental Animals Co. Ltd. (Chengdu, China), were used to establish the ARDS model. All mice were male in order to avoid interference with the female estrus cycle and obtain more stable experimental results. All animals were kept in a specific pathogen free (SPF) environment with temperature and humidity of 22–24 ℃ and 50–60%, respectively. The animals had free access to food and water. Sterilized and residue-free wood shavings were used for animal bedding. Animal experiments were performed under a project license (No. 2021DL-003) granted by the Experimental Animal Ethics Committee of the Hospital of Chengdu University of Traditional Chinese Medicine, in compliance with institutional guidelines for the care and use of animals. A protocol was prepared before the study without registration. After 1 week of adaptive feeding, the research began.
In the specific experimental mouse modeling and grouping process, 20 mice were anesthetized by pentobarbital sodium at the dose of 50 mg/kg (i.p.), and subsequently received the LPS via the respiratory tract by nasal drip. Another 10 mice received the same operation with normal saline instead of LPS, and were used as the Normal control mice. Then, the ARDS mice were divided into 2 groups (n=10), including a Model (LPS) and a Treatment group (LPS+FSM). The selection of the above experimental animals is random.
The Control and Model (LPS) group were administered orally with normal saline (10 mL/kg), and the Treatment group (LPS+FSM) were received FSM at the dose of 50 mg/kg orally. All the mice received a 5 days’ treatment. At the end of the treatment, blood samples were collected from the abdominal aorta under anaesthetization by pentobarbital sodium at the dose of 50 mg/kg (i.p.). After blood sampling, mice were sacrificed via decapitation and the lung tissues were collected for further determination.
ELISA assays
Serum samples were prepared by centrifugation at 10,000 rpm for 10 minutes, then levels of TNF-α and IL-6 in serum were determined by commercial ELISA kits following the standard protocols of instruction.
Histopathology examination
Histopathology examinations were applied to analyze the inflammatory response of lung tissues in ARDS mice following the reported method with minor modifications (20). Lung tissues were collected and fixed in 4% paraformaldehyde for 24 hours, and then embedded in paraffin. After a series of standard protocols for tissue section preparation, the lung tissue section was cut into 5 µm and stained with H&E. The histopathological changes of lung tissues were analyzed and the representative figures were captured using an optical microscope (Olympus, Tokyo, Japan).
Immunohistochemical (IHC) examination
Conduct IHC experiments on mice from the three groups mentioned above. After the lung tissue section (5 µm) was prepared as described in previous method, protein expressions of AQP-5, SP-C, and Notch1 were detected by IHC examination. Then, the IHC determination was carried out with primary antibodies of AQP-5, SP-C, and Notch1. Primary antibodies included: AQP-5 (1:200, PA5-36529, Thermo Fisher), SP-C (1:200, PA5-76631, Thermo Fisher), and Notch1 (1:200, PA5-32522, Thermo Fisher), β-actin (1:5,000, AC026, ABclonal, USA). Briefly, the deparaffinized tissue sections were treated with citric acid and antigenic unmasked at 98 ℃ for 10 minutes and subsequently incubated with primary antibodies overnight at 4 ℃, followed by incubation with the secondary antibody at room temperature for 1 hour. Finally, the tissue sections were further stained with 3,3'-diaminobenzidine (DAB) solution. The target protein positive expressions of lung tissues were analyzed and the representative figures were captured using an optical microscope (Olympus, Japan).
Western blot assay
Total proteins of the lung tissues were extracted using radioimmunoprecipitation assay (RIPA) solution and the protein concentration was quantified by bicinchoninic acid (BCA) reagents. After separation of the protein bands by sodium dodecyl sulfate polyacrylamide gel electrophoresis (SDS-PAGE), the target protein bands were blotted on a polyvinylidene fluoride (PVDF) membrane, and subsequently incubated with primary antibodies of AQP-5, SP-C, and Notch1, respectively. Then, the PVDF membrane was incubated with HRP-conjugated secondary antibodies, and followed visualized by chemiluminescence with the enhanced chemiluminescence (ECL) chemicals.
HPLC analysis
The main compositions in FSM were determined by HPLC extracts (21,22). Separation was carried out using the Agilent 1200 High-Performance Liquid Chromatograph (Agilent, Santa Clara, CA, USA) with a Shimadsu InertSustain C18 column (250 mm × 4.6 mm, 5 µm; Shimadsu, Kyoto, Japan) at 30 ℃ using a gradient elution at a flow rate of 1.0 mL/min. The acetonitrile (B) and 0.1% formic acid-water (A) was used as the mobile phase and the gradient program was as following: 0–15 min, 5–20% A; 15–40 min, 20–25% A; 40–50 min, 25–35% A; 50–80 min, 35–65% A; 80–90 min, 65–65% A. The volume of injected sample was 10 µL and the detection wavelength was set at 230 nm.
Statistical analysis
All values were described as the mean ± standard deviation. The software SPSS 22.0 (IBM Corp., Armonk, NY, USA) was used for statistical testing, and significance was determined by one-way analysis of variance (ANOVA) with the least significant difference (LSD) test. Statistical significance was defined as P<0.05.
Results
FSM decreased pro-inflammatory cytokines in serum of ARDS mice
As shown in Figure 1, after LPS induction, the serum levels of IL-6 and TNF-α in ARDS mice were significantly increased (P<0.01, vs. Control). Interestingly, FSM treatment significantly reduced these 2 pro-inflammatory cytokines compared to the model mice (P<0.01).
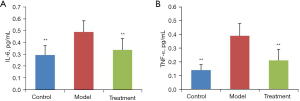
FSM improved pro-inflammatory responses in lung tissues of ARDS mice
As shown in Figure 2, after LPS induction, the alveolar septum was significantly broadened and obvious inflammatory reactions and pink homogenous edema fluids were observed in the alveolar cavity. In addition, the results also showed focal pulmonary dilatation, alveoli collapse, and multifocal hemorrhage. Interestingly, FSM significantly attenuated the inflammatory responses in lung tissues; no obvious alveoli collapse and multifocal hemorrhage were observed in the treatment group mice.
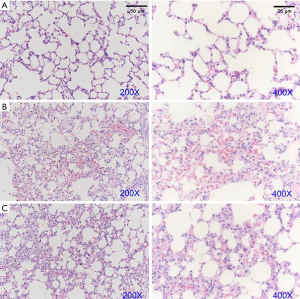
FSM increased AQP-5, SP-C, and Notch1 in lung tissues of ARDS mice
Subsequently, we explored the potential mechanisms of FSM for treating ARDS via western blot assays and IHC examinations. We determined the protein expressions of AQP-5, SP-C, and Notch1 (Figure 3) in the lung tissues of ARDS mice. Our results showed that after FSM treatment, the SP-C and AQP-5 were significantly increased, compared to the Model mice (P<0.01). Besides, FSM treatment also up-regulated the expression of Notch1 in the lung tissues of ARDS mice (P<0.001, vs. Model). In addition, similar results with the western blot assays were also observed from the IHC examinations on AQP-5 (Figure 4), SP-C (Figure 5), and Notch1 (Figure 6) in lung tissues of ARDS mice.
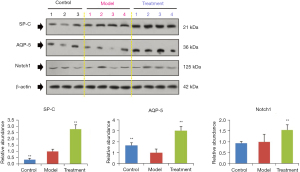
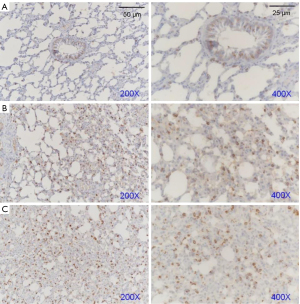
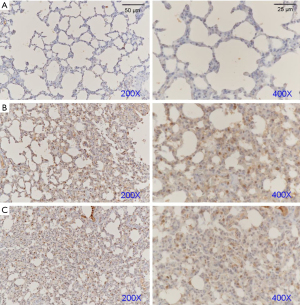
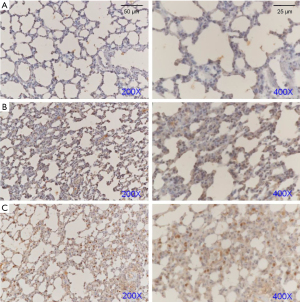
Chemical compositions of FSM
As shown in Figure 7, over 20 chromatography peaks were obtained from the HPLC chromatogram, and 8 constituents of FSM were identified by using standard reference agents, including (I) liquiritin, (II) isoliquiritin, (III) benzoylmesaconine, (IV) mesaconitine, (V) liquiritigenin, (VI) hypaconitine, (VII) glycyrrhizic acid, and (VIII) 6-gingerol, respectively.
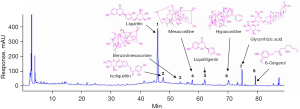
Discussion
FSM is a clinical empirical TCM formula for treating ARDS and other respiratory diseases. In the present study, we reported experimental animal evidences for FSM against ARDS for the first time, in addition, we also explored the related potential mechanisms.
The mouse model of LPS-induced ARDS is a consistent and reproducible ARDS animal model which can mimic the pathophysiology of ARDS in humans (23,24). In the real-world study, the proinflammatory cytokines TNF-α, IL-6, and IL-8 are among the most promising as biomarkers for predicting morbidity and mortality (25,26). In our present study, we established the LPS-induced ARDS mouse model to evaluate the curative effects of FSM against ARDS, and the results showed that FSM could significantly attenuate the ARDS symptoms of mice. The pathological characteristics of ARDS include increased pulmonary capillary permeability, uncontrolled local inflammatory responses, and inflammatory cytokines release, finally leading to systemic inflammatory response (23,27). Currently, it is generally recognized that uncontrolled inflammatory reactions are closely related to the development of ARDS, and the over-produced inflammatory cytokines of IL-1, IL-6, and TNF-α further exacerbated the injury of alveolus (26,27). Recently, a study showed that the inhibition of miR-129-5p may induce autophagy and inhibit the inflammatory response by promoting the expression of the PPAR-γ, thereby relieving ARDS (28). Our present results showed that FSM treatment could remarkably attenuate the inflammatory responses and injury of lung tissues induced by LPS challenge, including reduction of inflammatory exudation, decrease of alveoli collapse and multifocal hemorrhage, and so on. In addition, FSM treatment could also reduce the serum levels of IL-6 and TNF-α in ARDS mice.
SP-C, one of the pulmonary surfactants of the lung tissues, is important for maintaining the normal functions of lung tissues, such as regulation of alveolar surface tension and local defense system of lung (29). Importantly, recent studies have suggested that pulmonary surfactants could attenuate the ARDS both in children and adults (30,31). Aquaporins (AQP) are water channels with the function of regulating the balance of intracellular and intercellular water fluid (32). Current findings have shown that AQPs are closely correlated to the development of ARDS, and upregulation of AQPs are beneficial for improving ARDS (33,34). Notch/DLK1 is a key signaling pathway for the development of type II alveolar epithelial cells, which could be beneficial for the repair of lung function after ARDS (35-38). In our present study, we found that FSM treatment significantly up-regulated the protein expressions of SP-C, AQP-5, and Notch1 in lung tissues of ARDS mice. In addition, we also analyzed the main composition of FSM, and 8 potential active constituents were identified including (I) liquiritin, (II) isoliquiritin, (III) benzoylmesaconine, (IV) mesaconitine, (V) liquiritigenin, (VI) hypaconitine, (VII) glycyrrhizic acid, and (VIII) 6-gingerol. Consequently, future works might be devoted to investigating the activities of these monomers on ARDS and the related mechanisms.
In our study, we found that SP-C proteins were significantly elevated in the model group, either as a result of compensatory expression of the SP-C gene in the model construct or as a consequence of pulmonary epithelial cell damage in the model construct, which activates the repair mechanism of the body. Notably, the tissue expression of SP-C was further increased after pharmacological intervention, indicating that the intervention induced pulmonary epithelial cell proliferation accompanied by an up-regulation of SP-C protein expression. To determine the specific mechanisms of SP-C protein expression in the intervention arm, it is necessary to confirm this by histological analysis and specific staining in subsequent studies.
In the model group, AQP-5 was significantly reduced, indicating that the inhibition of the protein was significant in the modeling process, whereas in the intervention group, we found that AQP-5 was significantly elevated, even higher than in the control group. This suggests that the intervention not only affects the protein by improving the tissue status but is likely to have a regulatory effect on the AQP-5 gene. In subsequent studies, we need to validate the mechanisms by which the AQP-5 gene is regulated in primary cells.
Notch signaling pathway has a critical role in regulating cell fate determination, proliferation, and differentiation during development and tissue regeneration (38). In the adult, Notch is involved in repair and regeneration of several airway cells types. Finn reported that Notch1 expression was affected in type II pulmonary epithelial cells (39). Similarly, in the present study, FSM up-regulated the Notch expressions in lung tissues of ARDS mice, attenuating the inflammatory responses in lung tissues.
There are several limitations in this study. Firstly, there are too few inflammatory cytokine detections. Secondly, the research of signaling pathway is limited, due to the limited funds and conditions. In the future, we will continue to explore the relationship between FSM and the proliferation of alveolar epithelial cells.
Conclusions
In our study, FSM alleviates inflammatory reactions and promotes the proliferation of alveolar epithelial cells in LPS-induced ARDS mice via regulation of SP-C, AQP-5, and Notch1 in lung tissues, providing basic evidence for the clinical treatment.
Acknowledgments
We are grateful to the Experimental Animal Ethics Committee of the Hospital of Chengdu University of Traditional Chinese Medicine for checking this study.
Funding: This study was financially supported by the Traditional Chinese Medicine Science Foundation of Sichuan Provincial Administration of Traditional Chinese Medicine (No. 2023zd005).
Footnote
Reporting Checklist: The authors have completed the ARRIVE reporting checklist. Available at https://jtd.amegroups.com/article/view/10.21037/jtd-23-367/rc
Data Sharing Statement: Available at https://jtd.amegroups.com/article/view/10.21037/jtd-23-367/dss
Peer Review File: Available at https://jtd.amegroups.com/article/view/10.21037/jtd-23-367/prf
Conflicts of Interest: All authors have completed the ICMJE uniform disclosure form (available at https://jtd.amegroups.com/article/view/10.21037/jtd-23-367/coif). The authors have no conflicts of interest to declare.
Ethical Statement:
Open Access Statement: This is an Open Access article distributed in accordance with the Creative Commons Attribution-NonCommercial-NoDerivs 4.0 International License (CC BY-NC-ND 4.0), which permits the non-commercial replication and distribution of the article with the strict proviso that no changes or edits are made and the original work is properly cited (including links to both the formal publication through the relevant DOI and the license). See: https://creativecommons.org/licenses/by-nc-nd/4.0/.
References
- Bos LDJ, Ware LB. Acute respiratory distress syndrome: causes, pathophysiology, and phenotypes. Lancet 2022;400:1145-56. [Crossref] [PubMed]
- Gorman EA, O'Kane CM, McAuley DF. Acute respiratory distress syndrome in adults: diagnosis, outcomes, long-term sequelae, and management. Lancet 2022;400:1157-70. [Crossref] [PubMed]
- Fan E, Beitler JR, Brochard L, et al. COVID-19-associated acute respiratory distress syndrome: is a different approach to management warranted? Lancet Respir Med 2020;8:816-21. [Crossref] [PubMed]
- Toniati P, Piva S, Cattalini M, et al. Tocilizumab for the treatment of severe COVID-19 pneumonia with hyperinflammatory syndrome and acute respiratory failure: A single center study of 100 patients in Brescia, Italy. Autoimmun Rev 2020;19:102568. [Crossref] [PubMed]
- Tsai YT, Ku HC, Maithreepala SD, et al. Higher Risk of Acute Respiratory Distress Syndrome and Risk Factors among Patients with COVID-19: A Systematic Review, Meta-Analysis and Meta-Regression. Int J Environ Res Public Health 2022;19:15125. [Crossref] [PubMed]
- Reiss LK, Schuppert A, Uhlig S. Inflammatory processes during acute respiratory distress syndrome: a complex system. Curr Opin Crit Care 2018;24:1-9. [Crossref] [PubMed]
- Huppert LA, Matthay MA, Ware LB. Pathogenesis of Acute Respiratory Distress Syndrome. Semin Respir Crit Care Med 2019;40:31-9. [Crossref] [PubMed]
- Bu C, Wang R, Wang Y, et al. Taraxasterol Inhibits Hyperactivation of Macrophages to Alleviate the Sepsis-induced Inflammatory Response of ARDS Rats. Cell Biochem Biophys 2022;80:763-70. [Crossref] [PubMed]
- Kloc M, Ghobrial RM, Lipińska-Opałka A, et al. Effects of vitamin D on macrophages and myeloid-derived suppressor cells (MDSCs) hyperinflammatory response in the lungs of COVID-19 patients. Cell Immunol 2021;360:104259. [Crossref] [PubMed]
- Ma X, Liu X, Feng J, et al. Fraxin Alleviates LPS-Induced ARDS by Downregulating Inflammatory Responses and Oxidative Damages and Reducing Pulmonary Vascular Permeability. Inflammation 2019;42:1901-12. [Crossref] [PubMed]
- Yıldırım F, Karaman İ, Kaya A. Current situation in ARDS in the light of recent studies: Classification, epidemiology and pharmacotherapeutics. Tuberk Toraks 2021;69:535-46. [Crossref] [PubMed]
- Fielding-Singh V, Matthay MA, Calfee CS. Beyond Low Tidal Volume Ventilation: Treatment Adjuncts for Severe Respiratory Failure in Acute Respiratory Distress Syndrome. Crit Care Med 2018;46:1820-31. [Crossref] [PubMed]
- Long Y, Yang Q, Xiang Y, et al. Nose to brain drug delivery - A promising strategy for active components from herbal medicine for treating cerebral ischemia reperfusion. Pharmacol Res 2020;159:104795. [Crossref] [PubMed]
- Wang J, Wong YK, Liao F. What has traditional Chinese medicine delivered for modern medicine? Expert Rev Mol Med 2018;20:e4. [Crossref] [PubMed]
- Filardo S, Di Pietro M, Mastromarino P, et al. Therapeutic potential of resveratrol against emerging respiratory viral infections. Pharmacol Ther 2020;214:107613. [Crossref] [PubMed]
- Ren JL, Zhang AH, Wang XJ. Traditional Chinese medicine for COVID-19 treatment. Pharmacol Res 2020;155:104743. [Crossref] [PubMed]
- Diao Y, Ding Q, Xu G, et al. Qingfei Litan Decoction Against Acute Lung Injury/Acute Respiratory Distress Syndrome: The Potential Roles of Anti-Inflammatory and Anti-Oxidative Effects. Front Pharmacol 2022;13:857502. [Crossref] [PubMed]
- Zhang S, Ding P, Xu MX, et al. Effect of Fusu Mixture on mechanical ventilation in patients with acute respiratory distress syndrome. J North Sichuan Med Coll 2020;35:220-3.
- Gao PY, Wang CX, Zheng XH. A study on effect of Fusu mixture on haemodynamics in patients with septic shock. Chin J TCM WM Crit Care 2010;17:337-9.
- Zhang Q, Peng W, Wei S, et al. Guizhi-Shaoyao-Zhimu decoction possesses anti-arthritic effects on type II collagen-induced arthritis in rats via suppression of inflammatory reactions, inhibition of invasion & migration and induction of apoptosis in synovial fibroblasts. Biomed Pharmacother 2019;118:109367. [Crossref] [PubMed]
- Gao C, Zhu M, Xu W, et al. Chemical constituents from the stems and leaves of Amomum villosum Lour. and their anti-inflammatory and antioxidant activities. Bioorg Chem 2023;131:106281. [Crossref] [PubMed]
- Li Y, Lin Z, Wang Y, et al. Unraveling the mystery of efficacy in Chinese medicine formula: New approaches and technologies for research on pharmacodynamic substances. Arab J Chem 2022;15:104302. [Crossref] [PubMed]
- Wu X, Yu L, Long D, et al. Study on the protective effects and mechanism of berberine hydrochloride on sepsis induced acute respiratory distress syndrome in mice. J Pract Med 2019;35:3452-6.
- Jiang L, Yao M, Yang M, et al. Study on rats with acute respiratory distress syndrome induced by intratracheal instillation of lipopolysaccharide in different ways. Clin J Crit Care Med 2019;12:80-4.
- Xu BQ, Zhou Y. The effects of blood purification combined with antibiotics on extravascular lung water index, inflammatory factors, and prognosis of patients with severe acute pancreatitis complicated with acute respiratory distress syndrome. Ann Palliat Med 2021;10:9792-9. [Crossref] [PubMed]
- Qin M, Qiu Z. Changes in TNF-α, IL-6, IL-10 and VEGF in rats with ARDS and the effects of dexamethasone. Exp Ther Med 2019;17:383-7. [PubMed]
- Dang W, Tao Y, Xu X, et al. The role of lung macrophages in acute respiratory distress syndrome. Inflamm Res 2022;71:1417-32. [Crossref] [PubMed]
- Zhu D, Zhou M, Wang K, et al. The downregulation of miR-129-5p relieves the inflammatory response in acute respiratory distress syndrome by regulating PPAR-γ-mediated autophagy. Ann Transl Med 2022;10:345. [Crossref] [PubMed]
- Korolainen H, Lolicato F, Enkavi G, et al. Dimerization of the pulmonary surfactant protein C in a membrane environment. PLoS One 2022;17:e0267155. [Crossref] [PubMed]
- Li KC, Qian SY, Zeng JS. Current status of pulmonary surfactant in the treatment of acute respiratory distress syndrome. Chin J Pract Pediatr 2018;33:4311-434.
- Li S, Huang S, Cai Y. Curative effects of pulmonary surfactant in treatment of neonatal acute respiratory distress syndrome. Chin Med Pharm 2020;10:166-8.
- Tran E, Shi T, Li X, et al. Development of human alveolar epithelial cell models to study distal lung biology and disease. iScience 2022;25:103780. [Crossref] [PubMed]
- Li C, Wang W. Molecular Biology of Aquaporins. Adv Exp Med Biol 2017;969:1-34. [Crossref] [PubMed]
- Xiao M, Zhu T, Wang T, et al. Diammonium glycyrrhizinate promotes aquaporin-5 in LPS induced acute lung injury via inactivation of NF-κB. Sichuan Da Xue Xue Bao Yi Xue Ban 2014;45:376-9. [PubMed]
- Gao J, Zeng LX. The effects of Notch signaling in acute injury of Lung. J Clin Exp Pathol 2014;30:544-6.
- Aspal M, Zemans RL. Mechanisms of ATII-to-ATI Cell Differentiation during Lung Regeneration. Int J Mol Sci 2020;21:3188. [Crossref] [PubMed]
- Liu X, Zhu X, Zhu G, et al. Effects of Different Ligands in the Notch Signaling Pathway on the Proliferation and Transdifferentiation of Primary Type II Alveolar Epithelial Cells. Front Pediatr 2020;8:452. [Crossref] [PubMed]
- Liu J, Sato C, Cerletti M, et al. Notch signaling in the regulation of stem cell self-renewal and differentiation. Curr Top Dev Biol 2010;92:367-409. [Crossref] [PubMed]
- Finn J, Sottoriva K, Pajcini KV, et al. Dlk1-Mediated Temporal Regulation of Notch Signaling Is Required for Differentiation of Alveolar Type II to Type I Cells during Repair. Cell Rep 2019;26:2942-2954.e5. [Crossref] [PubMed]