Target genes of N6-methyladenosine regulatory protein ALKBH5 are associated with prognosis of patients with lung adenocarcinoma
Highlight box
Key findings
• ALKBH5 was highly expressed in LUAD tissues and was significantly associated with a poor prognosis. Upregulation of ZNF777, TCOF1, CPLX2, and ABL1 was associated with a poor prognosis, whereas upregulation of ZER1, VPS53, and RRBP1 was associated with a good prognosis.
What is known and what is new?
• The expression of the N6-methyladenosine regulatory protein ALKBH5 is associated with lung cancer. ALKBH5 was highly expressed in LUAD tissues and was significantly associated with a poor prognosis.
What is the implication, and what should change now?
• We screened target genes of ALKBH5 in LUAD, some of which were associated with the prognosis of patients with LUAD. This study provides targets and a basis for the development of novel treatments for LUAD.
Introduction
Lung cancer has a higher annual fatality rate than pancreatic, prostate, or breast cancer (1). Lung adenocarcinoma (LUAD) is the most common subtype of lung cancer (2), with 5-year survival rate of only 17% (3). Patients with early-stage disease and without metastatic tumors care candidates for standard surgical resection. However, most patients with LUAD are diagnosed at an advanced stage and can only be treated with chemoradiotherapy, which is associated with a high mortality rate (4). Therefore, in-depth studies of the molecular mechanisms underlying LUAD prognosis are of great value for the diagnosis and treatment of this cancer.
N6-methyladenosine (m6A), an adenosine derivative from RNA, plays a major role in regulating gene expression and is the most common mRNA modification in mammals (5). Li et al. (6) found that m6A modification contributes to the formation of the tumor microenvironment in patients with LUAD and consequently is associated with its prognosis. The m6A family consists of regulatory proteins that can “write”, such as methyltransferase-like protein 3 (METTL3), METTL14, and Wilms’ tumor 1-associating protein; “read”, including YTH domain-containing family proteins and insulin-like growth factor 2 mRNA-binding protein family; and “erase”, including α-ketoglutarate-dependent dioxygenase and α-ketoglutarate-dependent dioxygenase alkB homolog 5 (ALKBH5) (7). ALKBH5 has been identified as the primary demethylase of the m6A family (8). ALKBH5 is a target of hypoxia-inducible factor and is upregulated during hypoxia (9). ALKBH5 demethylase activity can promote the progression of various cancers, including liver cancer and glioblastoma (10,11), but its overexpression has been reported to inhibit cancer cell growth in bladder and pancreatic cancers (12,13). The conflicting roles of ALKBH5 in different cancers warrant further investigation. In LUAD, the upregulation of METTL3 and downregulation of ALKBH5 together lead to an increase in m6A levels and are often associated with a poor prognosis. Furthermore, knockdown of METTL3 and overexpression of ALKBH5 can inhibit lung tumor formation in a mouse model (14). Therefore, studies of the regulatory mechanisms of ALKBH5 are crucial to understand its influence on LUAD formation, prognosis, and treatment.
A549 cell lines with and without ALKBH5 knockdown were sequenced by methylated RNA immunoprecipitation sequencing (MeRIP-seq). We combined these data with mRNA sequencing data and clinical information for LUAD samples from The Cancer Genome Atlas (TCGA). The goal was to identify target genes of ALKBH5 and their association with LUAD prognosis. The newly identified target genes provide insights into the mechanism of action of ALKBH5 and are candidate targets for the treatment of LUAD. We present this article in accordance with the STREGA reporting checklist (available at https://jtd.amegroups.com/article/view/10.21037/jtd-22-1464/rc).
Methods
Expression of ALKBH5 in LUAD and paracarcinoma tissues
TCGA-LUAD expression and clinical profile data were downloaded using the Xena online platform of the University of Santa Cruz [https://xenabrowser.net/datapages/?cohort=GDC%20TCGA%20Lung%20Adenocarcinoma%20(LUAD)&removeHub=https%3A%2F%2Fxena.treehouse.gi.ucsc.edu%3A443]. The relative expression levels of ALKBH5 in 50 pairs of LUAD and paracancerous tissues were compared using paired-sample t-tests.
In addition, using 478 LUAD samples from TCGA, the relative expression levels of ALKBH5 were compared among patients at different pathological stages by t-tests. The ggpubr R package was used to visualize the results, and the significance threshold was set to P<0.05.
Relationship between ALKBH5 expression and LUAD prognosis
To analyze the relationship between the expression of ALKBH5 and the prognosis of LUAD, prognostic information and ALKBH5 expression data for 478 patients with LUAD were obtained from TCGA. The cutoff R package was used to calculate the optimal cutoff value for ALKBH5 expression based on the smallest P value. The survival package in R (15) was used for survival analysis, and the Survminer R package was used to draw the Kaplan-Meier (KM) survival curve for ALKBH5.
Screening of genes related to ALKBH5 expression
Based on the expression profiles for 478 LUAD samples, Pearson correlation coefficients between the expression levels of ALKBH5 and other genes were calculated. The thresholds for significantly correlated expression were correlation coefficient ǀrǀ >0.2 and P<0.05.
Genes significantly associated with ALKBH5 were further evaluated by Gene Ontology (GO) and Kyoto Encyclopedia of Genes and Genomes (KEGG) enrichment analyses using the clusterProfiler R package (version 4.4.4) (16). Terms with counts greater than or equal to 2 and Bonferroni-corrected P<0.05 were considered significantly enriched.
Analyzing ALKBH5 target genes
The LUAD cell line A549 was transfected with three small hairpin RNAs (shRNAs) and the scramble lentiviral vector pLHO.1 for stable ALKBH5 silencing. Quantitative reverse transcription polymerase chain reaction (RT-qPCR) was used to verify the transfection efficiency. RNA was extracted using TRIzol. m6A RNA immunoprecipitation (IP) was performed using the GenSeqTM m6A-MeRIP Kit (GenSeq Inc., Nanjing, China) following the manufacturer’s instructions. Both input samples without IP and m6A IP samples were used for RNA-seq library generation using the NEBNext® Ultra II Directional RNA Library Prep Kit (New England Biolabs, Inc., Ipswich, MA, USA). The library quality was evaluated with the BioAnalyzer 2100 system (Agilent Technologies, Inc., Santa Clara, CA, USA). Library sequencing was performed using an Illumina HiSeq instrument to obtain 150-bp paired-end reads. These reads were subjected to quality control with a threshold score of Q30. Subsequently, 3' adaptor trimming and the removal of low-quality reads were performed using cutadapt (v1.9.3). Clean reads from all libraries were aligned to the reference genome (HG38) using Hisat2 (v2.0.4). Methylated sites on RNAs (peaks) were identified using magnetic cell sorting (MACS). Differentially methylated sites were identified using diffReps. Peaks identified by both MACS and diffReps overlapping with exons were identified using homemade scripts.
Given that ALKBH5 is a demethylation gene, the methylation level of genes related to ALKBH5 will increase after knockdown of ALKBH5 (17). Therefore, genes with elevated methylation levels in ALKBH5-knockdown cells compared with normal LUAD cells with P<0.00001 and logFC <2 were selected for further analyses. GO and KEGG analyses of these genes were performed using clusterProfiler. Counts greater than or equal to 2 and Bonferroni-corrected P<0.05 were set as the significance thresholds for the enrichment analysis.
To further narrow down the number of target genes, the intersection between genes with significantly upregulated methylation levels in ALKBH5-knockdown cells and ALKBH5-related genes based on correlated expression patterns were identified as candidate targets of ALKBH5. The VennDiagram package in R (version 1.7.3) (18) was used to draw Venn plots. The functions of the target genes were assessed by GO and KEGG analyses using clusterProfiler. The significance threshold for the enrichment analyses was set as previously described.
In addition, interactions between target genes were assessed using the protein-protein interaction (PPI) database STRING (version 11.5) (19). Cytoscape (version 3.9.1) (20) was used to visualize the PPI network with a score of 0.15.
Prognostic analysis of ALKBH5 target genes
Pearson’s correlation coefficients between the expression of ALKBH5 and its target genes were calculated to assess the correlation between the genes. The R package ggplot2 (21) was used to visualize the correlation results. The cutoff package was used to calculate the optimal cutoff value of ALKBH5 target gene expression. The Survminer package was used to draw the KM survival curve for ALKBH5. The study was conducted in accordance with the Declaration of Helsinki (as revised in 2013).
Statistical analysis
We compared standardized differences for all covariates between LUAD and paracarcinoma tissues. Continuous data are presented as the mean ± standard deviation (SD) and were analyzed with Student’s t-tests for independent data. Categoric variables are presented as a count and percentage of patients and compared using the χ2 or Fisher’s exact test. Pearson correlation coefficients between the expression levels of different genes were calculated. All P values less than 0.05 were considered statistically significant. All tests were two-sided, with an alpha level of 0.05. For all statistical evaluations, IBM SPSS Statistics 22.0 (IBM Corp, Armonk, NY, USA) and R 2.15.3 (2013; The R Foundation for Statistical Computing, Vienna, Austria) were used.
Results
High expression of ALKBH5 in LUAD tissues
The expression levels of ALKBH5 were compared between LUAD and paired paracancerous tissues. ALKBH5 expression was higher in LUAD tissues than in paracancerous tissues (P<0.05, Figure 1).
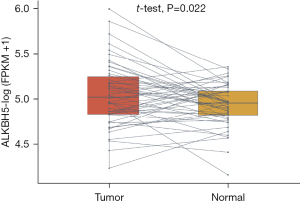
ALKBH5 expression levels in patients with LUAD at different pathological stages are presented in Figure S1. No obvious association between ALKBH5 expression and different tumor node metastasis stages or stages of LUAD was identified.
High expression of ALKBH5 is a poor prognostic factor
According to the optimal cutoff value, patients with LUAD were divided into ALKBH5 high- and low-expression groups. The KM curve exhibited a significant difference in prognosis between the two groups of patients; in particular, the prognosis of patients in the high ALKBH5 expression group was worse than that of patients in the low expression group (P<0.05, Figure 2).
Correlated expression analysis
Based on Pearson correlation coefficients, we identified 774 genes that were significantly related to ALKBH5 expression, including 459 and 315 genes with positively and negatively correlated expression, respectively. For functional assessment of these related genes, GO and KEGG enrichment analyses were performed, and only the top 20 terms were retained (Figure 3). These terms were all classified within the biological process category in GO analysis. The genes were primarily enriched in proteasomal protein catabolic processes, cellular component disassembly, negative regulation of transport, I-kappaB kinase/nuclear factor kappa B (NF-κB) signaling, and response to molecules of bacterial origin. A KEGG pathway analysis revealed that these genes were primarily involved in herpes simplex virus 1 infection, Salmonella infection, lipid and atherosclerosis, and human cytomegalovirus infection.
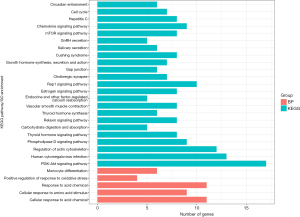
Fifteen genes were screened as targets of ALKBH5
The expression of ALKBH5 in A549 cells decreased significantly after shRNA-mediated ALKBH5 silencing (Figure S2). To obtain the ALKBH5 target genes, we obtained the intersection of genes correlated with ALKBH5 expression and upregulated genes (365 genes) after ALKBH5 knockdown in LUAD cells. Ultimately, 15 genes (ANKRD11, LACTB, VPS53, TCOF1, ABL1, HSP90B1, RRBP1, NCOR2, TFF3, ZER1, CPLX2, NDST1, DHRS7B, ZNF777, and KATNIP) were identified as potential candidate target genes of ALKBH5 (Figure 4A). GO and KEGG enrichment analyses were used to functionally characterize these genes (Figure 4B). The genes were enriched for 21 GO terms (20 biological processes and one molecular function) and one KEGG pathway. These 21 terms included protein processing in the endoplasmic reticulum, transcriptional coregulator activity, regulation of small molecule metabolic processes, and cell activation involved in the immune response. In addition, a PPI network constructed using these 15 targets demonstrated that 10 target genes were included (Figure 4C). Nuclear receptor corepressor 2 (NCOR2) was a core gene in this network and interacted with ankyrin repeat domain-containing 11 (ANKRD11), zinc finger protein 777 (ZNF777), and nonreceptor tyrosine kinase (ABL1).
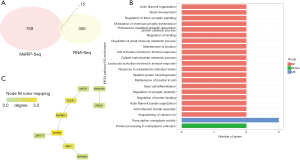
Prognostic value of target genes
Except for β-actin (ACTB), the target genes were positively correlated with the expression of ALKBH5 (Figure S3). In addition, we analyzed the relationship between the 15 target genes and the prognosis of patients with LUAD (only significant results are presented in Figure 5). Patients with high expression of ZNF777, treacle ribosome biogenesis factor 1 (TCOF1), complexin 2 (CPLX2), or ABL1 exhibited worse survival probabilities, whereas patients with high expression of zyg-11 related cell cycle regulator (ZER1), a subunit of the GARP complex (VPS53), or ribosome binding protein 1 (RRBP1) had better prognoses.
Discussion
A large number of patients with LUAD develop drug resistance, and a considerable proportion of patients die from the disease despite rapid therapeutic development (22). Studies have demonstrated that the genesis and development of tumors are regulated by m6A modifications (23). Here, we found that ALKBH5 is differentially expressed between LUAD and paracancerous tissues and is associated with poor prognosis. Furthermore, we synthesized TCGA-LUAD expression profile data and MeRIP-seq data for A549 cell lines with and without ALKBH5 silencing and identified 15 target genes.
We screened 774 genes significantly associated with ALKBH5 expression and investigated their functions in GO and KEGG enrichment analyses. NF-κB is a regulator of inflammation and cell survival and contributes to tumor growth, proliferation, and metastasis (24,25). Abnormal activation of the NF-κB signaling pathway is related to the invasion and migration of LUAD, whereas its downregulation induces apoptosis in non-small cell lung cancer cells (26). In this study, ALKBH5-related genes were enriched in the NF-κB signaling pathway.
ALKBH5-related genes were also enriched in proteasomal protein catabolic processes. The proteasome plays an important role in protein homeostasis, critical for maintaining cellular stress responses (27), and dysfunctional complexes may contribute to cancer development. Proteasome complex-related genes that promote the cell cycle are highly expressed in lung squamous cell carcinomas (28). These genes have been identified as targets of anticancer drugs (29). Zhang et al. (30) reported that proteasome subunits are highly correlated with proteasome 26S subunit (PSMC6) expression and that PSMC6 may promote the progression of LUAD by activating the Wingless-type (WNT) signaling pathway.
The 15 target genes identified in this study were primarily involved in the regulation of metabolic processes of small molecules and cell activation involved in the immune response. The PPI network revealed that NCOR2 may be an important gene that interacts with ZNF777, ABL1, and ANKRD11. NCOR2 modifies chromatin structures and inhibits the basal transcriptional activity of target genes; its abnormal expression is associated with various cancers (31). Downregulation of NCOR2 is associated with poor prognosis in breast cancer and LUAD, as NCOR2 can enhance the invasive ability of breast cancer cells and may contribute to cisplatin resistance in LUAD cells (32,33). Gong et al. (34) reported that chromatin assembly factor 1 subunit B (CHAF1B) can induce cisplatin resistance by increasing the ubiquitination and degradation of NCOR2. In a prognostic analysis, patients with LUAD with high expression of ZNF777, TCOF1, CPLX2, or ABL1 exhibited worse survival probabilities, whereas those with high expression of ZER1, VPS53, or RRBP1 had a better prognosis. Several of these genes implicated in prognosis have been associated with cancer. For instance, the downregulation of family with sequence similarity 129 (FAM129A) mediated by ZNF777 inhibits colorectal cancer cell proliferation (35). TCOF1 may affect the prognosis of multiple cancer types by participating in the cell cycle and cellular senescence pathways (36). RRBP1 is highly expressed in lung cancer tissues; knockout of RRBP1 promotes the endoplasmic reticulum stress response and reduces tumorigenicity (37).
Not all of the target genes were associated with prognosis in LUAD. There are several explanations for this observation. For example, not all targets may contribute to LUAD. Alternatively, these targets may not be involved in LUAD independently but may exert functions via interactions with other genes. Previous studies have demonstrated that not all modified sites are equally important, and only some play a key role in biological processes (10). More research is needed to determine the specific mechanism of action of ALKBH5 and its target genes. Although the identification of 15 target genes that are strongly associated with LUAD provides a good basis for further analyses, this study had some limitations. First, we only screened genes related to the expression of ALKBH5, and the interactions between these genes require further experimental verification. Second, given the heterogeneity of tumors, the amount of retrospective data included in this study was still insufficient. Therefore, it is necessary to expand the sample size in future studies. Finally, more prospective studies are imperative to validate these results.
Conclusions
In conclusion, we screened target genes of ALKBH5 in LUAD, some of which were associated with the prognosis of patients with LUAD. This study provides targets and a basis for the development of novel treatments for LUAD.
Acknowledgments
Funding: This work was supported by the State Key Laboratory of Respiratory Diseases (No. SKLRD-QN-201903) and the Guangzhou Science and Technology Planning Project (No. 202102010342).
Footnote
Reporting Checklist: The authors have completed the STREGA reporting checklist. Available at https://jtd.amegroups.com/article/view/10.21037/jtd-22-1464/rc
Conflicts of Interest: All authors have completed the ICMJE uniform disclosure form (available at https://jtd.amegroups.com/article/view/10.21037/jtd-22-1464/coif). The authors have no conflicts of interest to declare.
Ethical Statement: The authors are accountable for all aspects of the work in ensuring that questions related to the accuracy or integrity of any part of the work are appropriately investigated and resolved. The study was conducted in accordance with the Declaration of Helsinki (as revised in 2013).
Open Access Statement: This is an Open Access article distributed in accordance with the Creative Commons Attribution-NonCommercial-NoDerivs 4.0 International License (CC BY-NC-ND 4.0), which permits the non-commercial replication and distribution of the article with the strict proviso that no changes or edits are made and the original work is properly cited (including links to both the formal publication through the relevant DOI and the license). See: https://creativecommons.org/licenses/by-nc-nd/4.0/.
References
- Siegel RL, Miller KD, Jemal A. Cancer statistics, 2020. CA Cancer J Clin 2020;70:7-30. [Crossref] [PubMed]
- Wang Q, Li M, Yang M, et al. Analysis of immune-related signatures of lung adenocarcinoma identified two distinct subtypes: implications for immune checkpoint blockade therapy. Aging (Albany NY) 2020;12:3312-39. [Crossref] [PubMed]
- Duan J, Lei Y, Lv G, et al. Identification of a novel autophagy signature for predicting survival in patients with lung adenocarcinoma. PeerJ 2021;9:e11074. [Crossref] [PubMed]
- Spella M, Stathopoulos GT. Immune Resistance in Lung Adenocarcinoma. Cancers (Basel) 2021;13:384. [Crossref] [PubMed]
- He PC, He C. m(6) A RNA methylation: from mechanisms to therapeutic potential. EMBO J 2021;40:e105977. [Crossref] [PubMed]
- Li Y, Gu J, Xu F, et al. Molecular characterization, biological function, tumor microenvironment association and clinical significance of m6A regulators in lung adenocarcinoma. Brief Bioinform 2021;22:bbaa225. [Crossref] [PubMed]
- Han H, Fan G, Song S, et al. piRNA-30473 contributes to tumorigenesis and poor prognosis by regulating m6A RNA methylation in DLBCL. Blood 2021;137:1603-14. [Crossref] [PubMed]
- Mauer J, Luo X, Blanjoie A, et al. Reversible methylation of m(6)A(m) in the 5’ cap controls mRNA stability. Nature 2017;541:371-5. [Crossref] [PubMed]
- Kaur S, Tam NY, McDonough MA, et al. Mechanisms of substrate recognition and N6-methyladenosine demethylation revealed by crystal structures of ALKBH5-RNA complexes. Nucleic Acids Res 2022;50:4148-60. [Crossref] [PubMed]
- Zhang S, Zhao BS, Zhou A, et al. m(6)A Demethylase ALKBH5 Maintains Tumorigenicity of Glioblastoma Stem-like Cells by Sustaining FOXM1 Expression and Cell Proliferation Program. Cancer Cell 2017;31:591-606.e6. [Crossref] [PubMed]
- Chen Y, Zhao Y, Chen J, et al. ALKBH5 suppresses malignancy of hepatocellular carcinoma via m(6)A-guided epigenetic inhibition of LYPD1. Mol Cancer 2020;19:123. [Crossref] [PubMed]
- Yu H, Yang X, Tang J, et al. ALKBH5 Inhibited Cell Proliferation and Sensitized Bladder Cancer Cells to Cisplatin by m6A-CK2α-Mediated Glycolysis. Mol Ther Nucleic Acids 2021;23:27-41. [Crossref] [PubMed]
- Guo X, Li K, Jiang W, et al. RNA demethylase ALKBH5 prevents pancreatic cancer progression by posttranscriptional activation of PER1 in an m6A-YTHDF2-dependent manner. Mol Cancer 2020;19:91. [Crossref] [PubMed]
- Ma L, Xue X, Zhang X, et al. The essential roles of m(6)A RNA modification to stimulate ENO1-dependent glycolysis and tumorigenesis in lung adenocarcinoma. J Exp Clin Cancer Res 2022;41:36. [Crossref] [PubMed]
- Therneau TM, Grambsch PM. The Cox Model. In: Modeling Survival Data: Extending the Cox Model. New York, NY, USA: Springer New York, 2000:39-77.
- Yu G, Wang LG, Han Y, et al. clusterProfiler: an R package for comparing biological themes among gene clusters. OMICS 2012;16:284-7. [Crossref] [PubMed]
- Meyer KD, Jaffrey SR. The dynamic epitranscriptome: N6-methyladenosine and gene expression control. Nat Rev Mol Cell Biol 2014;15:313-26. [Crossref] [PubMed]
- Chen H, Boutros PC. VennDiagram: a package for the generation of highly-customizable Venn and Euler diagrams in R. BMC Bioinformatics 2011;12:35. [Crossref] [PubMed]
- Szklarczyk D, Franceschini A, Kuhn M, et al. The STRING database in 2011: functional interaction networks of proteins, globally integrated and scored. Nucleic Acids Res 2011;39:D561-8. [Crossref] [PubMed]
- Shannon P, Markiel A, Ozier O, et al. Cytoscape: a software environment for integrated models of biomolecular interaction networks. Genome Res 2003;13:2498-504. [Crossref] [PubMed]
- Wickham H. Data analysis. In: ggplot2. Cham: Springer, 2016:189-201.
- Xu F, Huang X, Li Y, et al. m(6)A-related lncRNAs are potential biomarkers for predicting prognoses and immune responses in patients with LUAD. Mol Ther Nucleic Acids 2021;24:780-91. [Crossref] [PubMed]
- Sun T, Wu R, Ming L. The role of m6A RNA methylation in cancer. Biomed Pharmacother 2019;112:108613. [Crossref] [PubMed]
- Gaultier A, Arandjelovic S, Niessen S, et al. Regulation of tumor necrosis factor receptor-1 and the IKK-NF-kappaB pathway by LDL receptor-related protein explains the nti-inflammatory activity of this receptor. Blood 2008;111:5316-25. [Crossref] [PubMed]
- Ren S, Wang J, Xu A, et al. Integrin α6 overexpression promotes lymphangiogenesis and lymphatic metastasis via activating the NF-κB signaling pathway in lung adenocarcinoma. Cell Oncol (Dordr) 2022;45:57-67. [Crossref] [PubMed]
- Xu L, Wu Q, Zhou X, et al. TRIM13 inhibited cell proliferation and induced cell apoptosis by regulating NF-κB pathway in non-small-cell lung carcinoma cells. Gene 2019;715:144015. [Crossref] [PubMed]
- Gu Y, Barwick BG, Shanmugam M, et al. Downregulation of PA28α induces proteasome remodeling and results in resistance to proteasome inhibitors in multiple myeloma. Blood Cancer J 2020;10:125. [Crossref] [PubMed]
- Chen M, Liu X, Du J, et al. Differentiated regulation of immune-response related genes between LUAD and LUSC subtypes of lung cancers. Oncotarget 2017;8:133-44. [Crossref] [PubMed]
- Guo X, Dixon JE. The 26S proteasome: A cell cycle regulator regulated by cell cycle. Cell Cycle 2016;15:875-6. [Crossref] [PubMed]
- Zhang JY, Shi KZ, Liao XY, et al. The Silence of PSMC6 Inhibits Cell Growth and Metastasis in Lung Adenocarcinoma. Biomed Res Int 2021;2021:9922185. [Crossref] [PubMed]
- Cui J, Yang Y, Zhang C, et al. FBI-1 functions as a novel AR co-repressor in prostate cancer cells. Cell Mol Life Sci 2011;68:1091-103. [Crossref] [PubMed]
- Alam H, Li N, Dhar SS, et al. HP1γ Promotes Lung Adenocarcinoma by Downregulating the Transcription-Repressive Regulators NCOR2 and ZBTB7A. Cancer Res 2018;78:3834-48. [Crossref] [PubMed]
- Martínez-Iglesias OA, Alonso-Merino E, Gómez-Rey S, et al. Autoregulatory loop of nuclear corepressor 1 expression controls invasion, tumor growth, and metastasis. Proc Natl Acad Sci U S A 2016;113:E328-37. [Crossref] [PubMed]
- Gong L, Hu Y, He D, et al. Ubiquitin ligase CHAF1B induces cisplatin resistance in lung adenocarcinoma by promoting NCOR2 degradation. Cancer Cell Int 2020;20:194. [Crossref] [PubMed]
- Yuki R, Aoyama K, Kubota S, et al. Overexpression of zinc-finger protein 777 (ZNF777) inhibits proliferation at low cell density through down-regulation of FAM129A. J Cell Biochem 2015;116:954-68. [Crossref] [PubMed]
- Gu W, Sun L, Wang J, et al. The oncogenic role of treacle ribosome biogenesis factor 1 (TCOF1) in human tumors: a pan-cancer analysis. Aging (Albany NY) 2022;14:943-60. [Crossref] [PubMed]
- Zhou H, Zhu Y, Qi H, et al. Evaluation of the prognostic values of solute carrier (SLC) family 39 genes for patients with lung adenocarcinoma. Aging (Albany NY) 2021;13:5312-31. [Crossref] [PubMed]