Epithelial-mesenchymal transition is associated with osteopontin-induced EGFR‑TKI resistance in EGFR mutant non-small cell lung cancer
Highlight box
Key findings
• The present study showed that the osteopontin (OPN)-phosphatidylinositol-3 kinase (PI3K)/protein kinase B (AKT)-epithelial-mesenchymal transition (EMT) pathway plays a critical role in epidermal growth factor receptor-tyrosine kinase inhibitor (EGFR-TKI) resistance in non-small cell lung cancer (NSCLC).
What is known and what is new?
• EGFR-TKIs exert a favorable therapeutic effect in advanced NSCLC patients with positive EGFR mutations. Previous research has examined the mechanisms of acquired EGFR-TKI resistance via the EGFR-T790M secondary mutation, human epidermal growth factor receptor 2 amplification, mesenchymal-epithelial transition factor (MET) amplification, and EMT.
• This study showed that the OPN-PI3K/AKT-EMT pathway is involved in EGFR-TKI resistance.
What is the implication, and what should change now?
• Our findings may lead to the use of newly designed targeted treatments in clinical trials that can circumvent this resistance pathway and have potential in laboratory investigations, which may provide a possible therapeutic target for overcoming EGFR-TKI resistance.
Introduction
Lung cancer has become one of the most prevalent cancers and is the main cause of cancer-related mortality worldwide (1,2). Despite great advances in our understanding of the molecular mechanisms underlying non-small cell lung cancer (NSCLC) and the development of more effective treatments, the overall 5-year survival rate for lung cancer patients remains low (3). Unfortunately, individuals with advanced or metastatic NSCLC have a poor prognosis and are resistant to traditional chemotherapy (4). However, the development of epidermal growth factor receptor-tyrosine kinase inhibitors (EGFR-TKIs) has provided substantial benefits for patients with advanced NSCLC that harbor activating EGFR mutations (e.g., the L858R mutation, which occurs due to deletions in both exon 19 and exon 21). First-line treatments with EGFR-TKIs, such as gefitinib, erlotinib, afatinib, dacomitinib, and Osimertinib, are effective for these patients (5-9). However, despite their initial efficacy, most patients develop resistance to EGFR-TKIs over time (10,11). Most acquired resistance results from the EGFR-T790M secondary mutation, human epidermal growth factor receptor 2 amplification, mesenchymal-epithelial transition factor (MET) amplification, and histological alterations, including epithelial-mesenchymal transition (EMT) (12-16). Unfortunately, a few resistive mechanisms remain elusive. Ongoing clinical studies and future perspective studies need to be conducted to extend understandings of the mechanisms of resistance in the specific genetic and clinical characteristics of NSCLC. Effective medicines for individuals who have developed resistance to EGFR-TKIs need to be urgently developed.
Osteopontin (OPN) is a glycosylated phosphoprotein found in the extracellular matrix that is expressed in a wide range of human tissues and is also overexpressed in numerous malignancies (17). There is considerable evidence that OPN contributes to bone resorption, immune function, wound repairing, angiogenesis, and cancer biology (18,19). OPN was found to be upregulated in NSCLC and involved in the metastasis of NSCLC (20,21). Further, research has shown that OPN knockdown suppresses NSCLC cell invasion and metastasis (22). OPN overexpression has been linked to advanced disease, poor prognosis, lymph node metastases, and frequent recurrence (23,24). The results of our earlier research showed that OPN has the potential to serve as a metastasis-associated or particular biomarker for lung cancer and a possible therapeutic target for the treatment of metastatic disease (21). Additionally, there is accumulating evidence that OPN is involved in chemo-resistance in various cancers (25-27). Further, OPN promotes cell proliferation in NSCLC by engaging the integrin αV3/FAK pathway, which contributes to the acquisition of EGFR-TKI resistance (28). We previously demonstrated that OPN induced EMT formation (21,29), a process that causes epithelial cells to lose their cell polarity and gain mesenchymal cell markers and traits, such as motility, contractility, and invasiveness (30,31), conferring resistance to EGFR-TKIs (32).
Based on the above-mentioned studies, OPN upregulation in NSCLC contributes to drug resistance and tumor recurrence, two factors that contribute to poor outcomes for patients. There is no evidence that OPN-EMT causes acquired resistance to EGFR-TKIs. This study sought to determine how OPN and EMT is associated with the development of EGFR-TKI resistance in NSCLC. We present this article in accordance with the MDAR reporting checklist (available at https://jtd.amegroups.com/article/view/10.21037/jtd-23-818/rc).
Methods
Cell lines and reagents
The PC9 and PC9 gefitinib resistance (PC9GR) human lung cancer cell lines were obtained from the Shanghai Institute of Biological Science (Shanghai, China). To cultivate the cells, Roswell Park Memorial Institute 1640 Medium was supplemented with 10% heat-inactivated fetal bovine serum, streptomycin (100 mg/mL), and penicillin (100 U/mL). The cultured cells were kept in an incubator set at 37 ℃ with a 5% continuous carbon dioxide (CO2) supply. The drug gefitinib was provided by AstraZeneca (London, UK). R&D Systems China Co., Ltd. (Shanghai, China) provided both the human recombinant OPN and the secreted OPN (sOPN) enzyme-linked immunosorbent assay (ELISA) kits. The phosphatidylinositol-3 kinase (PI3K)/protein kinase B (AKT) inhibitor LY294002 was purchased from the Biovision Company (San Francisco, CA, USA). The anti-OPN rabbit polyclonal antibody was acquired from Abcam (Hong Kong, China). The rabbit monoclonal anti-pAKT, p38, MAPK, AKT, p-JNK, ERK, JNK, AKT, and ERK, and E-cadherin antibodies were obtained from Cell Signaling Technology (Danvers, MA, USA). The anti-vimentin mouse monoclonal antibodies were provided by Santa Cruz Biotechnology (CA, USA).
Human tissues and immunohistochemistry (IHC)
In total, 8 pairs of tumor tissue samples from EGFR mutant NSCLC patients were obtained between 2019 and 2021 from the Department of Pulmonary and Critical Medicine, First Affiliated Hospital, Wenzhou Medical University. The IHC analysis was performed following an IHC-established protocol, which we described in detail previously (21). In brief, the formalin-fixed and paraffinized tissues were sliced into 4-µm thick segments and were then treated overnight at 4 ℃ with rabbit anti-human OPN antibody (200 dilutions, Abcam), followed by biotinylated secondary antibodies. All the immunoreactions were independently assessed by 2 pathologists. The strength of a stain was given a score of 0, 1, 2, or 3, with 0 being weak and 3 being very strong. Further, the percentage was given a score according to 4 categories, where a score of 0 represented 0%, 1 represented 1–33%, 2 represented 34–66%, and 3 represented 67–100%.
This study was approved by the Ethics Committee in Clinical Research (ECCR) of The First Affiliated Hospital of Wenzhou Medical University (No. 2022-R126). All the methods were carried out in accordance with the relevant guidelines, regulations and declaration of Helsinki (as revised in 2013). Informed consent was obtained from all subjects or their legal guardians.
RNA extraction and quantitative real‑time polymerase chain reaction (qRT-PCR) evaluation
Cultured cell RNA was isolated using TRIzol reagent (Invitrogen, Carlsbad, CA, USA), and complementary DNA (cDNA) was synthesized using the SuperScript First-Strand Synthesis System (Invitrogen, Carlsbad, CA, USA) in accordance with the instructions of the manufacturer. Starting with 1 µL of cDNA and a Synergy Brands (SYBR) Green Polymerase Chain Reaction (PCR) Master Kit (Toyobo, Japan), a 2-stage PCR reaction was run using ABI 7000 PCR apparatus (Eppendorf, Hamburg, Germany) as follows: 1 min at 95 ℃, followed by 40 cycles of 5 s at 95 ℃ and 30 s at 60 ℃. The glyceraldehyde-3-phosphate dehydrogenase (GAPDH) gene was used as a benchmark for comparison. The data presented represent the mean of 3 separate experiments and were normalized to GAPDH expression. The “raw” cycle threshold (CT) value of each sample was standardized to the housekeeping gene before the analysis. Next, the normalized ΔCT value was adjusted to control the cell samples so that ΔΔCT could be obtained. Table S1 details all of the primer sequences.
Western blot (WB)
Radioimmunoprecipitation assay buffer was used for the intracellular protein extraction (Cell Signalling Technology, Danvers, MA, USA). Protein (50 µg) contents were obtained by employing the sodium dodecyl-sulfate polyacrylamide gel electrophoresis technique. Next, the obtained protein content was loaded onto polyvinylidene difluoride membranes and subjected to the appropriate primary and secondary antibody treatments. The increased chemiluminescence approach was used in the process of developing the membranes (Pierce, Rockford, IL, USA). After being subjected to X-ray film, the bands were observed using Western Lightning Enhanced Chemiluminescence (Thermo Fisher Scientific, MA, USA). Each experiment was repeated 3 times to validate the obtained results. The Phoretix 1D program was then used to analyze the results.
ELISAs
In a 24-well plate, 5×103 cells per well were seeded. The cells were allowed to adhere for 48 h before fresh medium was provided. Next, to determine the amount of sOPN, the supernatants were collected. As per the manufacturer’s instructions, sOPN was measured by ELISAs. After adding the protein samples and standards, the polystyrene microplates containing 96 wells that had been coated with OPN primary antibody were placed in an incubator for 2 h. After washing the plates, they were treated for 2 h with an OPN conjugate antibody and then washed again. After being washed 3 times, 100 µL of substrate solution was placed in each well, and the reaction was ended after 30 min by the addition of the stop solution. The absorbance at 450 nm was measured with a spectrophotometer (Thermo, MA, USA), and the sOPN concentration was determined by comparing the experimental results to the standard curve.
Immunofluorescence staining
Sterile cover-slips were used to seed the cells into 24-well cell culture plates at a concentration of 1×104 cells per ml. The cells were given 48 h to grow. After being washed 3 times in phosphate buffered saline (PBS), the cells were fixed with 4% paraformaldehyde for 20 min at room temp, permeabilized with 1% Triton X-100 for 20 min, and finally washed another 3 times. The cells were then blocked in PBS with 10% goat serum for 30 min. Primary antibodies, rabbit anti-E-cadherin 1:200, and mouse anti-Vimentin 1:200, were added to the cells after they had been washed 3 times in PBS. Each chamber was rinsed 3 times for 5 min with PBS before being incubated with the secondary antibody for 1 h at 25 ℃. After 5 min of counterstaining with 4',6'-diamidino-2-phenylindole and being washed 3 times in PBS, the nuclei were visible. Finally, the cells were put under an immunofluorescence microscope (Olympus/BX51, Tokyo, Japan) to check for fluorescence.
Viability and proliferation assays
As per the instructions from the manufacturer, a Cell Counting Kit-8 (CCK-8) test kit (Dojindo, Kumamoto, Japan) was used to measure cell viability and growth. Each well of a 96-well plate was seeded with 3,000 cells, and the following day, the cells were allowed to proliferate before being treated with various drug dosages for 72 h. Next, 10 µL of CCK-8 reagent was transferred to each well after 2 h of incubation at 37 ℃ with 5% CO2. The fluorescence at 630 and 450 nm was then measured with a microplate reader (Bio-Rad Laboratories Inc., Hercules, CA, USA).
Flow cytometric evaluation
The cells were plated at a density of 5×104 per well in 6-well plates and then exposed either to the test compound or to dimethyl sulfoxide as a negative control. After being washed 3 times with PBS, the cells were collected, re-suspended in binding buffer, and stained for 20 min with propidium iodide and annexin V-fluorescein isothiocyanate (Sigma, St. Louis, MO, USA). Flow cytometry (BD Bioscience, San Diego, CA, USA) and Cell Quest software were used to examine cell apoptosis (Biomedika, Canada).
RNA interference and transfection
The small-interfering RNA (siRNA)-containing lentivirus vector was provided by Ribobio Co. (Guangzhou, Guangdong, China). The transfection was performed using Lipofectamine 2000 transfection reagent (Invitrogen, Carlsbad, NM, USA) as per the manufacturer’s instructions. In short, the cells were inoculated in 12-well plates and were then transfected using 10 µL of optimized lentivirus. Additionally, each experiment had controls that contained the transfection agent and the control vector. Both qRT-PCR and WB were used to examine OPN after 24 h. After being exposed to the lentivirus vector, the stably transduced cells were used in the subsequent experiments after being cultivated with puromycin at a concentration of 5 µg/mL for 2 weeks. Table S1 sets out the sense and antisense strands of the siRNAs.
Statistical analysis
All the data are presented as the mean ± the standard error of the mean and were based on at least 3 independent experiments. After the analysis of variance, the statistical significance was assessed between the groups using the Student’s t-test. A P value <0.05 was considered statistically significant. All the statistical evaluations were conducted using Graph-Pad Prism-version 7.0 and SPSS-version 17.0 software.
Results
OPN was upregulated in human EGFR-TKI resistant NSCLC tissues and cells
To examine the expression levels of OPN in the tumor tissues from the human EGFR-TKI resistant NSCLC patients, 8 pairs of lung adenocarcinoma tumor samples before and after the development of EGFR-TKI resistance were analyzed. IHC revealed positive staining for OPN in the cytoplasm and elevated levels of OPN in the 8 patients with EGFR-TKI resistance (Figure 1A,1B). Further, the messenger RNA (mRNA) (Figure 1C) and protein (Figure 1D,1E) levels of OPN were elevated in the NSCLC tissues following the development of EGFR-TKI resistance. We selected PC9, a TKI-sensitive cell line, and PC9GR, a gefitinib-resistant cell line, to analyze the effect of OPN levels on the regulation of TKI sensitivity. The levels of OPN were determined by qRT-PCR (Figure 1F) and WB (Figure 1G). According to the data obtained from this study, the PC9GR cells expressed OPN at a higher level than the PC9 cells. As a classical secretory protein, OPN is thought to have many functions. The amount of sOPN produced was determined by ELISAs. The result revealed that the PC9GR cells exhibited higher levels of sOPN expression than the PC9 cells (Figure 1H).
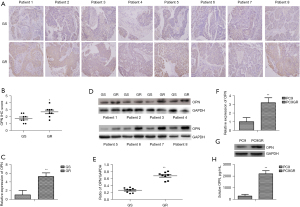
The role of OPN in EGFR-TKI resistance in NSCLC
The mutant EGFR NSCLC cell lines (i.e., PC9GR and PC9) were treated with varying doses of gefitinib to evaluate the role of OPN in the development of acquired EGFR-TKI resistance in NSCLC. According to the findings, the PC9GR cells were much less susceptible to gefitinib than the PC9 cells (Figure 2A). A stable PC9GR cell line in which OPN was downregulated was developed by siRNA sequencing against OPN to further investigate whether OPN was positively linked with acquired resistance to EGFR-TKIs (si-OPN). SiRNA transient transfection in the PC9GR cells significantly downregulated OPN mRNA and protein (Figure 2B,2C). After si-OPN transfection, sOPN was also suppressed (Figure 2D). The CCK-8 assays indicated that the PC9GR cells were highly sensitive to gefitinib after OPN was silenced (Figure 2D). In addition, OPN knockdown enhanced gefitinib-induced apoptosis in the drug-resistant PC9GR (Figure 2E-2G). Based on these findings, OPN may contribute to the formation of gefitinib-resistant NSCLC cells.
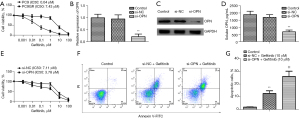
OPN-EMT association conferred resistance to EGFR-TKIs
We also studied whether or not the EGFR-TKI-resistant NSCLC cells had a similar pattern of OPN expression to that of the EMT-related protein. For the duration specified, gefitinib was administered to the PC9 cells. Gefitinib at 0.05 µM significantly elevated OPN mRNA (Figure 3A), inhibited E-cadherin mRNA (Figure 3B), and induced vimentin mRNA (Figure 3C) beginning at 24 h post-stimulus and continuing for 48 h. Similarly, the expression of sOPN (Figure 3D) and EMT-related protein (Figure 3E: OPN, E-cadherin and vimentin) were examined by ELISA and WB. Additionally, the results showed that the knockdown of OPN at least partially averted the EMT process in the gefitinib-treated PC9 cells (Figure 3F-3H). Conversely, the PC9GR cells expressed less E-cadherin mRNA (Figure 3I) and an elevated level of vimentin mRNA (Figure 3J), and similar results were demonstrated by WB (Figure 3K) and immunofluorescence staining (Figure 3L). Consistent with these findings, we found that OPN interference in the PC9GR cells increased E-cadherin expression (Figure 3M) but decreased vimentin expression (Figure 3N,3O). Based on these results, it seems that OPN, through the EMT process, helps cells become resistant to EGFR-TKIs.
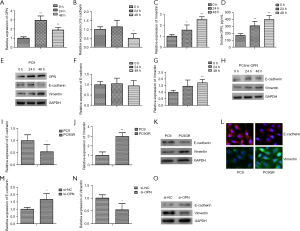
OPN induced the EMT of NSCLC cells by upregulating the PI3K/AKT signaling cascade
The potential mechanisms by which OPN mediated EMT related to EGFR-TKIs in EGFR mutant NSCLC were examined based on the evidence that OPN contributed to the acquisition of EGFR-TKI resistance through EMT (Figure 3). Unlike the PC9 cells, the PC9GR cells had elevated levels of phosphorylated AKT (Figure 4A), and silencing OPN suppressed this activation (Figure 4B). Additionally, the treatment of the PC9 cells with 1,000 ng/mL of recombinant human OPN resulted in a significant increase in AKT phosphorylation as early as 20 min (Figure 4C). Pretreatment with LY294002, a selective inhibitor of PI3K activity, prevented the OPN-induced downregulation or upregulation of E-cadherin mRNA (Figure 4D) or vimentin mRNA (Figure 4E), and similar results were demonstrated by WB (Figure 4F). When the PC9GR cells were treated with LY294002, they became more sensitive to gefitinib, and the combination of OPN silencing and LY294002 inhibited PC9GR cell growth more than either drug alone (Figure 4G).
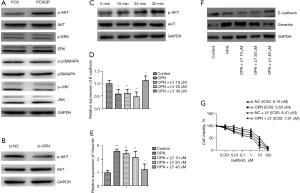
Discussion
Lung cancer is an aggressive malignancy with a high fatality rate worldwide, and NSCLC is responsible for nearly 85% of lung carcinoma cases (33,34). There have been significant advances in the diagnosis and treatment options over the past decade, however, the prognosis of NSCLC patients remains unsatisfactory. Most individuals are diagnosed with NSCLC at an advanced stage when it has already spread and is likely to reoccur (35). EGFR-TKIs have been shown to have a favorable therapeutic effect in advanced NSCLC patients with positive EGFR mutations and have been widely accepted as the treatment of first choice for this population (36,37). However, all patients eventually develop resistance to these medications, which limits their long-term therapeutic efficacy and is a serious clinical concern. After years of research, the majority of the causes of acquired EGFR-TKI resistance have been identified, but the phenomenon behind the remaining ~15% remains elusive. In this study, OPN overexpression was recorded in human EGFR-TKI-resistant NSCLC cells and tissues, and this overexpression contributed to the acquisition of EGFR-TKI resistance through the EMT in NSCLC.
OPN belongs to the family of tiny integrin-binding ligand N-linked glycoproteins, and it can bind to many different types of integrins and cell adhesion molecule 44 (CD44) receptors to modulate signaling cascades that control many different aspects of cell activity (18). It is known that integrin signaling participated in the EGFR-TKIs resistance. For example, Ichihara et al. reported SFK/FAK is activated by integrins to attenuates osimertinib efficacy in both drug-sensitive and drug-resistant models of EGFR-mutant lung cancer (38). In contrast to SFK/FAK downstream of integrin signaling, the present research focus on the upstream ligand of integrin to further elucidate the activate mechanism of integrin signaling. A large body of research has reported that OPN is closely correlated to tumor invasion, metastasis, and progression in different types of cancer (39-41). Consistent with these findings, our previous study also demonstrated that the overexpression of OPN is linked to high metastasis, early recurrence, and a poor prognosis in NSCLC (21). Fu et al. reported that OPN makes gefitinib resistance worse by increasing the expression of integrin αVβ3 (28). Wang et al. also reported that OPN contributes to the development of afatinib resistance in the lung cancer (15). In this study, we also demonstrated that the duration of EGFR-TKIs treatment in NSCLC patients is connected to OPN, and OPN elicits a protective effect against EGFR-TKI-induced apoptosis. Reducing OPN levels reduced resistance to EGFR-TKIs and increased the apoptosis caused by these drugs. Thus, OPN should be investigated as a possible therapeutic target for adjusting EGFR-TKI sensitivity in NSCLC patients.
We found that OPN drives EMT occurrence in lung cancer cells by reducing E-cadherin expression and increasing vimentin levels in response to stimulation, which provides further evidence that OPN has a regulatory function in EGFR-TKI resistance. OPN overexpression is associated with the repression of E-cadherin and the simultaneous induction of vimentin, which is also the case in EGFR-TKI-resistant NSCLC. Conversely, OPN inhibition, suppresses EMT development in cells resistant to EGFR-TKIs. Our findings suggest that OPN may affect patient prognosis through a changed EMT process and contribute to acquired EGFR-TKI resistance. Similar to our findings, previous studies have suggested that PI3K/AKT signaling is critical to the occurrence of EMT in human cancer cells (42-45). We found that the ability of OPN to cause EMT was mediated by PI3K/AKT signaling. This was supported by the fact that blocking PI3K/AKT activity with its specific inhibitor, LY294002, slowed down the ability of OPN to cause EMT. LY294002 also increased the sensitivity of cancer cells to EGFR-TKIs in the PC9GR cells, and silencing OPN in combination with LY294002 increased the sensitivity of the cancer cells to EGFR-TKIs more than any agent alone. These results suggest that OPN-PI3K/AKT-EMT may be a target to stop or slow the development of acquired resistance and lung cancer progression. This could provide a theoretical basis for new treatment options and strategies for patients with EGFR-TKI-acquired resistant NSCLC.
This study had a number of limitations. The 2 EGFR mutant NSCLC cell lines (i.e., PC9 and PC9GR) are frequently used to study the mechanisms of EGFR-TKI resistance. However, there are important distinctions between lung cancer cell lines and tumor tissues, and the association between cancerous cells and the tumor microenvironment is quite complex. Further, we showed that OPN-PI3K/AKT-EMT intervenes in the resistance of EGFR-TKIs in vitro. However, our findings still need to be verified in vivo to provide conclusive evidence for our conclusion. OPN has been implicated in acquired resistance to EGFR-TKIs, but further study in this area is warranted to achieve the ultimate goal of developing viable therapeutics for EGFR mutant NSCLC.
Conclusions
Taken together, the OPN mRNA and proteins were overexpressed in the human NSCLC tissues and cells that were resistant to EGFR-TKIs. This was linked to the presence of EMT. Through the activation of the OPN-PI3K/AKT-EMT pathway, both internal and external OPN played important roles in how the EGFR-TKIs acquired resistance (Figure 5). Our findings may lead to clinical trials employing newly designed targeted treatments that can circumvent these resistance pathways and have demonstrated potential in laboratory investigations.
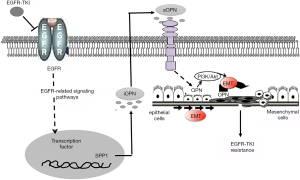
Acknowledgments
Funding: This study was supported by the Natural Science Foundation of Zhejiang Province (No. LQ20H010002 to Junjie Chen), the Health science and Technology Project of Zhejiang Province (No. 2022RC047 to Beibei Wang), the Key Laboratory of Interventional Pulmonology of Zhejiang Province (No. 2019E10014 to Lin Shi), the National Nature Science Foundation of China (No. 81800034 to Lin Shi), the Outstanding Youth Foundation of Zhongshan Hospital (No. 2021ZSYQ25 to Lin Shi), and the Basic Scientific Research Program of Wenzhou (Nos. Y20190207 to Yi Jin, Y20190522 to Junjie Chen, Y20220341 to Junjie Chen, and Y20220038 to Beibei Wang).
Footnote
Reporting Checklist: The authors have completed the MDAR reporting checklist. Available at https://jtd.amegroups.com/article/view/10.21037/jtd-23-818/rc
Data Sharing Statement: Available at https://jtd.amegroups.com/article/view/10.21037/jtd-23-818/dss
Peer Review File: Available at https://jtd.amegroups.com/article/view/10.21037/jtd-23-818/prf
Conflicts of Interest: All authors have completed the ICMJE uniform disclosure form (available at https://jtd.amegroups.com/article/view/10.21037/jtd-23-818/coif). JC reports funding support from the Natural Science Foundation of Zhejiang Province (No. LQ20H010002) and the Basic Scientific Research Program of Wenzhou (Nos. Y20190522 and Y20220341). LS reports funding support from the Key Laboratory of Interventional Pulmonology of Zhejiang Province (No. 2019E10014), the National Nature Science Foundation of China (No. 81800034), and the Outstanding Youth Foundation of Zhongshan Hospital (No. 2021ZSYQ25). YJ reports funding support from the Basic Scientific Research Program of Wenzhou (No. Y20190207). BW reports funding support from the Health Science and Technology Project of Zhejiang Province (No. 2022RC047) and the Basic Scientific Research Program of Wenzhou (No. Y20220038). The other authors have no conflicts of interest to declare.
Ethical Statement: The authors are accountable for all aspects of the work in ensuring that questions related to the accuracy or integrity of any part of the work are appropriately investigated and resolved. This study was approved by the Ethics Committee in Clinical Research (ECCR) of The First Affiliated Hospital of Wenzhou Medical University (No. 2022-R126). All the methods were carried out in accordance with the relevant guidelines, regulations and declaration of Helsinki (as revised in 2013). Informed consent was obtained from all subjects or their legal guardians.
Open Access Statement: This is an Open Access article distributed in accordance with the Creative Commons Attribution-NonCommercial-NoDerivs 4.0 International License (CC BY-NC-ND 4.0), which permits the non-commercial replication and distribution of the article with the strict proviso that no changes or edits are made and the original work is properly cited (including links to both the formal publication through the relevant DOI and the license). See: https://creativecommons.org/licenses/by-nc-nd/4.0/.
References
- Siegel RL, Miller KD, Fuchs HE, et al. Cancer statistics, 2022. CA Cancer J Clin 2022;72:7-33. [Crossref] [PubMed]
- Xia C, Dong X, Li H, et al. Cancer statistics in China and United States, 2022: profiles, trends, and determinants. Chin Med J (Engl) 2022;135:584-90. [Crossref] [PubMed]
- Goldstraw P, Crowley J, Chansky K, et al. The IASLC Lung Cancer Staging Project: proposals for the revision of the TNM stage groupings in the forthcoming (seventh) edition of the TNM Classification of malignant tumours. J Thorac Oncol 2007;2:706-14. [Crossref] [PubMed]
- Scagliotti GV, Parikh P, von Pawel J, et al. Phase III study comparing cisplatin plus gemcitabine with cisplatin plus pemetrexed in chemotherapy-naive patients with advanced-stage non-small-cell lung cancer. J Clin Oncol 2008;26:3543-51. [Crossref] [PubMed]
- Sequist LV, Yang JC, Yamamoto N, et al. Phase III study of afatinib or cisplatin plus pemetrexed in patients with metastatic lung adenocarcinoma with EGFR mutations. J Clin Oncol 2013;31:3327-34. [Crossref] [PubMed]
- Wu YL, Cheng Y, Zhou X, et al. Dacomitinib versus gefitinib as first-line treatment for patients with EGFR-mutation-positive non-small-cell lung cancer (ARCHER 1050): a randomised, open-label, phase 3 trial. Lancet Oncol 2017;18:1454-66. [Crossref] [PubMed]
- Rosell R, Carcereny E, Gervais R, et al. Erlotinib versus standard chemotherapy as first-line treatment for European patients with advanced EGFR mutation-positive non-small-cell lung cancer (EURTAC): a multicentre, open-label, randomised phase 3 trial. Lancet Oncol 2012;13:239-46. [Crossref] [PubMed]
- Jiang Y, Chen W, Yu W, et al. Survival analysis of afatinib versus erlotinib for individuals with advanced del19 lung adenocarcinoma with asymptomatic brain metastasis after pemetrexed-cisplatin chemotherapy: a retrospective study. J Int Med Res 2020;48:300060520937093. [Crossref] [PubMed]
- Wen F, Zheng H, Zhang P, et al. OPTIMAL and ENSURE trials-based combined cost-effectiveness analysis of erlotinib versus chemotherapy for the first-line treatment of Asian patients with non-squamous non-small-cell lung cancer. BMJ Open 2018;8:e020128. [Crossref] [PubMed]
- Cappuzzo F, Ciuleanu T, Stelmakh L, et al. Erlotinib as maintenance treatment in advanced non-small-cell lung cancer: a multicentre, randomised, placebo-controlled phase 3 study. Lancet Oncol 2010;11:521-9. [Crossref] [PubMed]
- Koulouris A, Tsagkaris C, Corriero AC, et al. Resistance to TKIs in EGFR-Mutated Non-Small Cell Lung Cancer: From Mechanisms to New Therapeutic Strategies. Cancers (Basel) 2022;14:3337. [Crossref] [PubMed]
- Ohashi K, Maruvka YE, Michor F, et al. Epidermal growth factor receptor tyrosine kinase inhibitor-resistant disease. J Clin Oncol 2013;31:1070-80. [Crossref] [PubMed]
- John T, Akamatsu H, Delmonte A, et al. EGFR mutation analysis for prospective patient selection in AURA3 phase III trial of osimertinib versus platinum-pemetrexed in patients with EGFR T790M-positive advanced non-small-cell lung cancer. Lung Cancer 2018;126:133-8. [Crossref] [PubMed]
- Onitsuka T, Uramoto H, Nose N, et al. Acquired resistance to gefitinib: the contribution of mechanisms other than the T790M, MET, and HGF status. Lung Cancer 2010;68:198-203. [Crossref] [PubMed]
- Wang M, Zhang D, Wang G, et al. HER2 amplification as a potential mechanism of acquired resistance to afatinib in an advanced non-small-cell lung cancer patient. Lung Cancer 2021;151:106-7. [Crossref] [PubMed]
- Chung JH, Rho JK, Xu X, et al. Clinical and molecular evidences of epithelial to mesenchymal transition in acquired resistance to EGFR-TKIs. Lung Cancer 2011;73:176-82. [Crossref] [PubMed]
- Shi L, Wang X. Role of osteopontin in lung cancer evolution and heterogeneity. Semin Cell Dev Biol 2017;64:40-7. [Crossref] [PubMed]
- Rangaswami H, Bulbule A, Kundu GC. Osteopontin: role in cell signaling and cancer progression. Trends Cell Biol 2006;16:79-87. [Crossref] [PubMed]
- Butler WT. Structural and functional domains of osteopontin. Ann N Y Acad Sci 1995;760:6-11. [Crossref] [PubMed]
- Ouyang X, Huang Y, Jin X, et al. Osteopontin promotes cancer cell drug resistance, invasion, and lactate production and is associated with poor outcome of patients with advanced non-small-cell lung cancer. Onco Targets Ther 2018;11:5933-41. [Crossref] [PubMed]
- Shi L, Hou J, Wang L, et al. Regulatory roles of osteopontin in human lung cancer cell epithelial-to-mesenchymal transitions and responses. Clin Transl Med 2021;11:e486. [Crossref] [PubMed]
- Sun BS, You J, Li Y, et al. Osteopontin knockdown suppresses non-small cell lung cancer cell invasion and metastasis. Chin Med J (Engl) 2013;126:1683-8. [PubMed]
- Zou XL, Wang C, Liu KE, et al. Prognostic significance of osteopontin expression in non-small-cell lung cancer: A meta-analysis. Mol Clin Oncol 2015;3:633-8. [Crossref] [PubMed]
- Chen L, Huan X, Xiao GH, et al. Osteopontin and its downstream carcinogenic molecules: regulatory mechanisms and prognostic value in cancer progression. Neoplasma 2022;69:1253-69. [Crossref] [PubMed]
- Hsieh IS, Huang WH, Liou HC, et al. Upregulation of drug transporter expression by osteopontin in prostate cancer cells. Mol Pharmacol 2013;83:968-77. [Crossref] [PubMed]
- Liu G, Fan X, Tang M, et al. Osteopontin induces autophagy to promote chemo-resistance in human hepatocellular carcinoma cells. Cancer Lett 2016;383:171-82. [Crossref] [PubMed]
- Gu T, Ohashi R, Cui R, et al. Osteopontin is involved in the development of acquired chemo-resistance of cisplatin in small cell lung cancer. Lung Cancer 2009;66:176-83. [Crossref] [PubMed]
- Fu Y, Zhang Y, Lei Z, et al. Abnormally activated OPN/integrin αVβ3/FAK signalling is responsible for EGFR-TKI resistance in EGFR mutant non-small-cell lung cancer. J Hematol Oncol 2020;13:169. [Crossref] [PubMed]
- Ho NT, Lin SW, Lee YR, et al. Osteopontin Splicing Isoforms Contribute to Endometriotic Proliferation, Migration, and Epithelial-Mesenchymal Transition in Endometrial Epithelial Cells. Int J Mol Sci 2022;23:15328. [Crossref] [PubMed]
- Lavin DP, Tiwari VK. Unresolved Complexity in the Gene Regulatory Network Underlying EMT. Front Oncol 2020;10:554. [Crossref] [PubMed]
- Das V, Bhattacharya S, Chikkaputtaiah C, et al. The basics of epithelial-mesenchymal transition (EMT): A study from a structure, dynamics, and functional perspective. J Cell Physiol 2019;234:14535-55. [Crossref] [PubMed]
- Chang TH, Tsai MF, Su KY, et al. Slug confers resistance to the epidermal growth factor receptor tyrosine kinase inhibitor. Am J Respir Crit Care Med 2011;183:1071-9. [Crossref] [PubMed]
- Yang D, Liu Y, Bai C, et al. Epidemiology of lung cancer and lung cancer screening programs in China and the United States. Cancer Lett 2020;468:82-7. [Crossref] [PubMed]
- Siegel RL, Miller KD, Jemal A. Cancer statistics, 2020. CA Cancer J Clin 2020;70:7-30. [Crossref] [PubMed]
- Wei L, Hu N, Ye M, et al. Overexpression of 14-3-3ζ primes disease recurrence, metastasis and resistance to chemotherapy by inducing epithelial-mesenchymal transition in NSCLC. Aging (Albany NY) 2022;14:5838-54. [Crossref] [PubMed]
- Kobayashi S, Boggon TJ, Dayaram T, et al. EGFR mutation and resistance of non-small-cell lung cancer to gefitinib. N Engl J Med 2005;352:786-92. [Crossref] [PubMed]
- Marin-Acevedo JA, Pellini B, Kimbrough EO, et al. Treatment Strategies for Non-Small Cell Lung Cancer with Common EGFR Mutations: A Review of the History of EGFR TKIs Approval and Emerging Data. Cancers (Basel) 2023;15:629. [Crossref] [PubMed]
- Ichihara E, Westover D, Meador CB, et al. SFK/FAK Signaling Attenuates Osimertinib Efficacy in Both Drug-Sensitive and Drug-Resistant Models of EGFR-Mutant Lung Cancer. Cancer Res 2017;77:2990-3000. [Crossref] [PubMed]
- Tan Y, Zhao L, Yang YG, et al. The Role of Osteopontin in Tumor Progression Through Tumor-Associated Macrophages. Front Oncol 2022;12:953283. [Crossref] [PubMed]
- Nakajima T, Uehara T, Iwaya M, et al. Osteopontin expression in the invasive front stroma of colorectal adenocarcinoma is associated with tumor budding and prognosis. Pathol Res Pract 2022;240:154190. [Crossref] [PubMed]
- Jámbor K, Koroknai V, Kiss T, et al. Gene Expression Patterns of Osteopontin Isoforms and Integrins in Malignant Melanoma. Pathol Oncol Res 2022;28:1610608. [Crossref] [PubMed]
- Chen XF, Zhang HJ, Wang HB, et al. Transforming growth factor-β1 induces epithelial-to-mesenchymal transition in human lung cancer cells via PI3K/Akt and MEK/Erk1/2 signaling pathways. Mol Biol Rep 2012;39:3549-56. [Crossref] [PubMed]
- Khan GJ, Gao Y, Gu M, et al. TGF-β1 Causes EMT by Regulating N-Acetyl Glucosaminyl Transferases via Downregulation of Non Muscle Myosin II-A through JNK/P38/PI3K Pathway in Lung Cancer. Curr Cancer Drug Targets 2018;18:209-19. [Crossref] [PubMed]
- Yuan R, Fan Q, Liang X, et al. Cucurbitacin B inhibits TGF-β1-induced epithelial-mesenchymal transition (EMT) in NSCLC through regulating ROS and PI3K/Akt/mTOR pathways. Chin Med 2022;17:24. [Crossref] [PubMed]
- Lin LL, Yang F, Zhang DH, et al. ARHGAP10 inhibits the epithelial-mesenchymal transition of non-small cell lung cancer by inactivating PI3K/Akt/GSK3β signaling pathway. Cancer Cell Int 2021;21:320. [Crossref] [PubMed]
(English Language Editor: L. Huleatt)