Primary graft dysfunction grade correlates with acute kidney injury stage after lung transplantation
Highlight box
Key findings
• Strong association exists between primary graft dysfunction (PGD) and acute kidney injury (AKI) severity, and between AKI and chronic kidney disease (CKD) severity after lung transplantation.
What is known and what is new?
• A few reports have investigated the association between PGD and AKI; however, the correlation between PGD and AKI severity in the entire lung transplantation cohort remained unclear.
• The impact of AKI severity on CKD risk and the temporal evolution of CKD grade has not been well investigated.
What is the implication, and what should change now?
• Our study provides a clinical basis for speculating the potential causal relationship between PGD and AKI.
• Challenges to severe PGD reduction with protection of renal function will improve the life expectancy and quality of life of lung transplant recipients.
Introduction
Primary graft dysfunction (PGD), resulting from ischemia reperfusion injury, affects over 50% of recipients within 24 hours of lung transplantation and has emerged as the most important risk factor for short-term mortality (1). It also has a potential impact on long-term survival, resulting in a higher rate of chronic lung allograft dysfunction (CLAD) (2,3).
Acute kidney injury (AKI), which commonly occurs after lung transplantation, is characterized by a rapid decline in the glomerular filtration rate (GFR) in response to acute stressors, leading to increased short- and long-term morbidity and mortality (4,5). In recent years, it has been reported that 23–69% of lung transplantation patients have AKI, 17–37% have stage 2–3 AKI, and 5–13% require renal replacement therapy (RRT) (6-12). Mortality rates due to AKI after lung transplantation range between 16–50% and are strongly associated with AKI stage, etiology, and comorbidities (13,14). Pooled odds ratios of in-hospital mortality rates in patients following lung transplantation with AKI and AKI requiring RRT were 2.75 (95% CI: 1.18–6.41) and 10.89 (95% CI: 5.03–23.58) (4). In previous studies, the intraoperative risk factors for AKI after lung transplantation included bilateral transplantation (6,8,9), hypoxia (15), hypotension (10), high-dose catecholamine (11), longer ischemic time (7), blood loss (6,11,12), and transfusion (6,12). In addition, volume depletion (16), diuretic use (16), sepsis (17), prolonged mechanical ventilation (10,18) and extracorporeal membrane oxygenation (ECMO) (18), and nephrotoxic drugs (19) were listed as postoperative risk factors (5). Despite advances in transplantation therapy, the incidence of AKI in post-lung transplant patients has not improved for at least a decade (4).
Previous studies have shown that PGD is a strong clinical risk factor of AKI. However, these studies did not classify AKI by severity (20), except for one report related only to cystic fibrosis (21). Therefore, the correlation between PGD and AKI severity in the entire lung transplantation cohort remained unclear. If there is a strong connection between PGD and AKI severity, it would be a good rationale to research the mechanism by which PGD leads AKI.
Lung transplantation patients with AKI are also at high risk of developing chronic kidney disease (CKD) (22). The 2019 annual report of the International Study Groups of Heart and Lung Transplantation (ISHLT) reported that 10 years after lung transplantation, the cumulative incidence of severe renal dysfunction was 24.6%, with 6.3% undergoing chronic dialysis and 3.6% receiving a kidney transplant (23). However, while the impact of the degree of AKI on subsequent CKD risk and CKD grade over time has been studied in patients with kidney disease, it has not been extensively studied in the field of lung transplantation (24).
In this study, we investigated the correlation between PGD grade and AKI stage using a single-center consecutive lung transplant database. We also investigated the impact of AKI stage on the GFR categories of CKD and its transition within one year. We present this article in accordance with the STROBE reporting checklist (available at https://jtd.amegroups.com/article/view/10.21037/jtd-23-256/rc).
Methods
Study design
Patient data were collected retrospectively using electronic medical records and stored in a database at the Northwestern University Medical Center in Chicago, Illinois, USA. Adult patients who underwent lung transplantation at our institution between January 2018 and June 2022 were included in the study. Multiorgan transplant recipients were excluded from the study. Tacrolimus trough level was used as the target of 8–12 ng/mL for all patients. All the patients underwent regular follow-up at our outpatient clinic after discharge. Data on patient demographics, comorbidities, including PGD grade, AKI stage, GFR category, donor characteristics, preoperative laboratory values, and intraoperative and postoperative outcomes were collected. This study was conducted in accordance with the Declaration of Helsinki (as revised in 2013). The study was approved by the Institutional Review Board of Northwestern University (Nos. STU00207250 and STU00213616). The need for patient consent was waived by the institutional review board because this was a retrospective study.
Statistical analysis
Recipient and donor characteristics, preoperative laboratory values, and intra- and postoperative outcomes were compared among the patients at each stage of AKI. One-way analysis of variance with Tukey’s range test and Kruskal-Wallis analysis with the Bonferroni test were used to compare continuous variables among the groups and calculate P values. The chi-square test was used to compare categorical variables, which were reported as numbers and percentages. The univariate logistic regression analysis was used to calculate odds ratios (ORs). Hazard ratios (HRs) for mortality were obtained using a univariate Cox regression analysis. Spearman’s test was used to compare PGD grades and AKI stages, and compare AKI stages and GFR categories of CKD. For sensitivity analysis, a dataset was created in which missing values in the GFR category of CKD were replaced by the Last Observation Carried Forward (LOCF) method, and the same Spearman test as above was performed. The Kaplan-Meier method was used to estimate survival, and the Wilcoxon test was performed to compare survival between the groups. Linear mixed-effects models were used to assess changes in CKD over time. Statistical significance was set at P<0.05. EZR software (Saitama Medical Center, Jichi Medical University, Saitama, Japan), a graphical user interface for R (The R Foundation for Statistical Computing, Vienna, Austria), was used to perform all the analyses (25).
Definition of complication and classification
PGD grade
PGD is defined based on the ISHLT guidelines (1). Patients with no evidence of pulmonary edema on chest X-ray (CXR) are considered grade 0. The absence of invasive mechanical ventilation was graded according to the partial pressure of arterial oxygen (PaO2)/fraction of inspired oxygen (FiO2) ratio, using methods similar to those used for mechanical ventilation. If PaO2 was not available for calculation of the PaO2/FiO2 ratio, then an oxygen saturation/FiO2 ratio was used. Grade 1: PaO2/FiO2 ratio >300; grade 2: PaO2/FiO2 ratio 200–300; grade 3: PaO2/FiO2 ratio <200. The lowest PaO2/FiO2 ratio within 72 hours after lung transplantation was used. The use of ECMO for bilateral pulmonary edema on CXR images was graded grade 3. The continuous use of ECMO without pulmonary edema on CXR imaging was excluded.
AKI stage
AKI stages were classified using the Kidney Disease: Improving Global Outcomes (KDIGO) criteria (26). Baseline serum creatinine (sCr) levels were measured immediately before transplantation. AKI was defined by: (I) a 0.3 mg/dL sCr increase, over any 48-hour period, to a level ≥0.3 mg/dL above baseline; (II) a 50% increase in sCr, over any 7-day period, to a level ≥50% above baseline; or (III) need for acute RRT. In addition, each AKI case was staged (stage 2: sCr ≥200% of baseline; stage 3: sCr ≥300% of baseline, sCr ≥4 mg/dL, or RRT use). Indications for RRT were determined by an experienced transplant nephrologist based on the eGFR, urine output, and electrolyte abnormalities.
GFR categories of CKD
The GFR categories of CKD were classified using the KDIGO criteria (27): Category 1: GFR ≥90 mL/min; Category 2: GFR =60–89 mL/min; Category 3A: GFR ≥45–59 mL/min; Category 3B: GFR ≥30–44 mL/min; Category 4: GFR ≥15–29 mL/min; Category 5: GFR <15 mL/min. The GFR was calculated using the 2009 Chronic Kidney Disease Epidemiology Collaboration equation (28). Albuminuria was not routinely examined and not used for staging CKD in this study.
CLAD
The ISHLT defined CLAD as a persistent decline (≥20%) in the measured forced expiratory volume in 1 second (FEV1) from the post-transplantation baseline that persisted for three months after the first value was taken (29). Patients with ≤3 total FEV1 measurements, precluding CLAD diagnosis, were excluded.
Indication of ECMO
Patients with respiratory failure were considered for veno-venous (VV)-ECMO if they failed to achieve satisfactory gas exchange (PaO2 >55 mmHg, oxygen saturations >88%, pH >7.2, with plateau pressures less than 35 cmH2O) despite lung protective mechanical ventilation and recruitment maneuvers with or without neuromuscular blockade. The decision to cannulate was made by a multidisciplinary ECMO team. All patients were cannulated by thoracic surgeons.
VV-ECMO management
Patients did not receive continuous anticoagulation unless there was a specific indication, such as deep venous thrombosis or pulmonary embolism, and there was no monitoring of bleeding parameters such as the activated clotting time or activated partial thromboplastin time, consistent with our prior study (30). All patients who were not receiving continuous systemic anticoagulation received 5,000 U subcutaneous unfractionated heparin every 8 hours as a prophylaxis dose to prevent deep venous thrombosis. VV-ECMO flow was maintained at a minimum of 3.0–3.5 L/min, consistent with our recent reports, to reduce thrombotic complications in the ECMO circuit (30). Transfusions were administered if any of the following criteria were met: platelets <50,000/mL, hemoglobin <7 g/dL, or hemodynamic instability in the setting of active blood loss. Different cannulation strategies [internal jugular vein-femoral vein cannulation or ProtekDuo® cannulation (CardiacAssist Inc., Pittsburgh, PA, USA)] were used in patients depending on surgeon preference. The VV-ECMO circuit included a Quadrox-iD adult (7.0) oxygenator (MAQUET Holding B.V. & Co. KG, Germany) and Rotaflow pump (MAQUET Holding B.V. & Co. KG, Germany). All components of the ECMO circuit had a heparin coating except for the cannulas.
Results
Patient demographics
A total of 206 patients were included in this study. Seven multi-organ transplant recipients were excluded from the study. There were no missing values for PGD grade and AKI stage. Median follow-up period was 471.0 days (interquartile range, 254.0–802.5 days). The patient data, divided by AKI stage, are summarized in Table 1. The numbers of patients with AKI stages 0, 1, 2, and 3 were 96 (46.6%), 47 (22.8%), 27 (13.1%), and 36 (17.5%), respectively. The use of preoperative hemodialysis and VV-ECMO, bilateral lung transplantation, low lung allocation score (LAS), coronavirus disease 2019 (COVID-19)-associated acute respiratory distress syndrome (ARDS), and low hemoglobin levels were more common in the AKI stage 3 group. Higher AKI severity was also observed in patients with longer operative time, higher transfusion use, and more intraoperative veno-arterial ECMO use (Table 2). Other postoperative complications were also more frequently identified, especially PGD grade 3, which was more common in AKI stage 3 [21 of 36 patients (58.3%)] than in AKI stage 0 [2 of 96 patients (2.1%)]. The length of stay in the intensive care unit, duration of ventilator connection, and length of hospital stay were also prolonged in the group with higher AKI. The use of postoperative hemodialysis and ECMO, as well as hemodialysis after discharge, was also more common in patients with higher AKI severity (Table 2). Comparing the AKI stage 0–2 and AKI stage 3 groups, the univariate logistic analysis showed that preoperative dialysis and ECMO use, high LAS, COVID-19-associated ARDS, high total bilirubin level, and female donors were more common in the AKI stage 3 group [dialysis use, odds ratio (OR) =7.76; ECMO use, OR =5.69; LAS, OR =1.03; COVID-associated ARDS, OR =2.87; high total bilirubin level, OR =1.80; and female donors, OR =2.76] (Table S1). Similarly, patients in the AKI stage 3 group had longer operative times, higher transfusion and intraoperative VA-ECMO use (operative times, OR =1.49; packed red blood cells, OR =1.13; and intraoperative VA-ECMO use, OR =2.96). Furthermore, PGD grades 1 to 3 and PGD grade 3 were significantly higher in AKI stage 3 than in AKI stage 0–2 (PGD grades 1 to 3, OR =5.94; and PGD grade 3, OR =32.6). The ventilator connections, and length of intensive care unit and hospital stays were also longer in the AKI stage 3 group (ventilator connections, OR =1.05; intensive care unit stay, OR =1.05; and hospital stay, OR =1.05) (Table S1).
Table 1
Variable | All cohort (n=206) | AKI stage 0 (n=96) | AKI stage 1 (n=47) | AKI stage 2 (n=27) | AKI stage 3 (n=36) | P value |
---|---|---|---|---|---|---|
Recipient factors | ||||||
Age (years) | 57.4±12.2 | 58.4±12.5 | 57.7±12.6 | 57.7±11.8 | 54.2±11.2 | 0.37 |
Female | 92 (44.7) | 44 (45.8) | 16 (34.0) | 12 (44.4) | 20 (55.6) | 0.27 |
BMI (kg/m2) | 25.8±4.6 | 25.9±4.2 | 25.8±5 | 24.7±4.7 | 26.2±4.9 | 0.62 |
Smoking history | 86 (41.7) | 42 (43.8) | 16 (34.0) | 11 (40.7) | 17 (47.2) | 0.62 |
Hypertension | 101 (49.0) | 41 (42.7) | 28 (59.6) | 13 (48.1) | 19 (52.8) | 0.28 |
Diabetes | 71 (34.5) | 33 (34.4) | 18 (38.3) | 9 (33.3) | 11 (30.6) | 0.90 |
Dialysis | 16 (7.8) | 2 (2.1) | 4 (8.5) | 1 (3.7) | 9 (25.0) | <0.001 |
Pre-ECMO use | 31 (15.0) | 5 (5.2) | 7 (14.9) | 6 (22.2) | 14 (38.9) | <0.001 |
Bilateral | 129 (62.6) | 47 (49.0) | 31 (66.0) | 22 (81.5) | 29 (80.6) | <0.001 |
LAS | 56.4±19.6 | 51.1±16.6 | 56.8±19.3 | 61.3±22.5 | 66.3±20.8 | <0.001 |
Etiology | ||||||
ILD | 78 (37.9) | 41 (42.7) | 16 (34.0) | 7 (25.9) | 14 (38.9) | 0.41 |
ARDS | 41 (19.9) | 10 (10.4) | 11 (23.4) | 7 (25.9) | 13 (36.1) | 0.006 |
COPD | 43 (20.9) | 21 (21.9) | 12 (25.5) | 5 (18.5) | 5 (13.9) | 0.61 |
PAH | 25 (12.1) | 14 (14.6) | 4 (8.5) | 5 (18.5) | 2 (5.6) | 0.31 |
Laboratory | ||||||
Hemoglobin (g/dL) | 11.1±2.6 | 11.8±2.4 | 10.9±2.5 | 10.0±2.6 | 10.4±2.8 | 0.002 |
WBC (1,000/mm3) | 9.9±3.9 | 9.5±3.9 | 10.1±3.3 | 10.4±4.6 | 10.5±4.2 | 0.53 |
Platelets (1,000/mm3) | 242.1±103.4 | 247.3±98.0 | 258.1±118.1 | 233.2±103.3 | 214.1±95.0 | 0.24 |
Sodium (mEq/L) | 139.9±3.2 | 139.6±3.0 | 139.4±3.0 | 140.7±3.2 | 140.9±3.8 | 0.07 |
BUN (mg/dL) | 16.7±8.3 | 16.3±8.0 | 16.1±6.3 | 19.0±9.6 | 16.9±10.4 | 0.49 |
Creatinine (mg/dL) | 0.70±0.20 | 0.78±0.22 | 0.73±0.22 | 0.67±0.25 | 0.74±0.25 | 0.15 |
eGFR (mL/min/1.73 m2) | 102.9±48.5 | 93.6±38.4 | 106.1±55.5 | 123.1±58.4 | 108.2±51.3 | 0.03 |
Total bilirubin (mg/dL) | 0.7±0.6 | 0.6±0.4 | 0.7±0.5 | 0.6±0.4 | 0.9±1.1 | 0.04 |
PRA | 92 (44.7) | 46 (47.9) | 15 (31.9) | 11 (40.7) | 20 (55.6) | 0.15 |
Arterial blood gas | ||||||
pH | 7.40±0.10 | 7.36±0.07 | 7.38±0.08 | 7.39±0.06 | 7.38±0.07 | 0.31 |
PaCO2 (mmHg) | 50.1±12.1 | 49.4±10.3 | 52.6±15.6 | 51.1±12.6 | 47.6±9.8 | 0.29 |
PaO2 (mmHg) | 268.5±121.4 | 295.8±118.0 | 240.2±127.8 | 266.7±113.2 | 243.0±117.2 | 0.049 |
Donor | ||||||
Age (years) | 33.0±12.0 | 32.8±11.8 | 31.1±12.2 | 35.0±11.5 | 34.4±12.6 | 0.50 |
Female | 68 (33.0) | 31 (32.3) | 12 (25.5) | 6 (22.2) | 19 (52.8) | 0.03 |
Cause of death | ||||||
Anoxia | 89 (43.2) | 47 (49.0) | 15 (31.9) | 8 (29.6) | 11 (30.6) | 0.07 |
Head trauma | 84 (40.8) | 30 (31.3) | 25 (53.2) | 12 (44.4) | 17 (47.2) | 0.06 |
Continuous data are shown as mean ± standard deviation and categorical data are shown as n (%). AKI, acute kidney injury; BMI, body mass index; ECMO, extracorporeal membrane oxygenation; LAS, lung allocation score; ILD, interstitial lung disease; ARDS, acute respiratory distress syndrome; COPD, chronic obstructive pulmonary disease; PAH, pulmonary arterial hypertension; WBC, white blood cell; BUN, blood urea nitrogen; eGFR, estimated glomerular filtration rate; PRA, panel reactive antibody; PaCO2, pressure of arterial carbon dioxide; PaO2, partial pressure of arterial oxygen.
Table 2
Variable | All cohort (n=206) | AKI stage 0 (n=96) | AKI stage 1 (n=47) | AKI stage 2 (n=27) | AKI stage 3 (n=36) | P value |
---|---|---|---|---|---|---|
Intraoperative outcomes | ||||||
Operative time (hours) | 7.3 (5.3–8.3) | 6.2 (5.0–7.9) | 7.5 (6.1–8.3) | 7.5 (6.5–9.6) | 8.4 (7.3–9.4) | <0.001 |
Intraoperative blood transfusion; PRBC (packs) | 1 (0–3.0) | 0 (0–2.0) | 1 (0–3.0) | 2 (1–5.5) | 3 (0–10.0) | <0.001 |
Intraoperative blood transfusion; FFP (packs) | 0 (0–1.0) | 0 (0–0) | 0 (0–1) | 0 (0–2.0) | 0 (0–2.5) | <0.001 |
Intraoperative blood transfusion; PLT (packs) | 0 (0–0) | 0 (0–0) | 0 (0–0) | 0 (0–1.5) | 0 (0–3.0) | <0.001 |
Ischemic time (hours) | 4.9 (4.1–5.8) | 4.5 (3.9–5.5) | 5.3 (4.2–5.8) | 5.3 (4.6–6.5) | 5.3 (4.5–5.8) | 0.01 |
VA-ECMO use | 127 (61.7) | 44 (45.8) | 31 (66.0) | 23 (85.2) | 29 (80.6) | <0.001 |
VA-ECMO time (hours) | 3.1 (2.5–3.7) | 3.4 (3.0–5.0) | 3.3 (3.0–6.7) | 4.3 (3.9–11.9) | 4.1 (3.1–5.8) | 0.048 |
Postoperative outcomes | ||||||
PGD grade 1 to 3 | 91 (44.2) | 29 (30.2) | 22 (46.8) | 12 (44.4) | 28 (77.8) | <0.001 |
PGD grade 3 | 28 (13.6) | 2 (2.1) | 5 (10.6) | 0 (0.0) | 21 (58.3) | <0.001 |
Dialysis | 29 (14.1) | 0 (0.0) | 0 (0.0) | 0 (0.0) | 7 (19.4) | <0.001 |
Stroke | 6 (2.9) | 2 (2.1) | 1 (2.1) | 1 (3.7) | 2 (5.6) | 0.73 |
Bowel ischemia | 2 (1.0) | 0 (0.0) | 0 (0.0) | 0 (0.0) | 2 (5.6) | 0.02 |
Digital ischemia | 6 (2.9) | 0 (0.0) | 0 (0.0) | 0 (0.0) | 6 (16.7) | <0.001 |
ICU stay (days) | 9.0 (6.0–19.3) | 7.0 (5.0–10.3) | 9.0 (5.0–15.0) | 13.0 (8.0–24.0) | 19.5 (14.0–33.8) | <0.001 |
Posttransplant ventilator (days) | 2.0 (1.0–4.3) | 2.0 (1.0–3.0) | 2.0 (1.0–5.0) | 2.0 (1.0–3.5) | 8.0 (2.0–17.3) | <0.001 |
Hospital stay (days) | 17.0 (12.0–29.5) | 12.0 (10.0–17.5) | 19.0 (15.0–27.0) | 23.0 (15.5–34.0) | 38.0 (27.0–54.3) | <0.001 |
Post-ECMO use | 34 (16.5) | 4 (4.2) | 6 (12.8) | 3 (11.1) | 22 (61.1) | <0.001 |
CLAD | 15 (7.3) | 9 (9.4) | 2 (4.3) | 0 (0.0) | 4 (11.1) | 0.15 |
HD after discharge | 37 (18.0) | 9 (9.4) | 9 (19.1) | 4 (14.8) | 15 (41.7) | <0.001 |
Continuous data are shown as medians (interquartile ranges) and categorical data are shown as n (%). AKI, acute kidney injury; PRBC, packed red blood cell; FFP, fresh frozen plasma; PLT, platelet; VA-ECMO, veno-arterial extracorporeal membrane oxygenation; PGD, primary graft dysfunction; ICU, intensive care unit; ECMO, extracorporeal membrane oxygenation; CLAD, chronic lung allograft dysfunction; HD, hemodialysis.
Survival after lung transplantation by AKI stage and PGD grade
The survival curve was stratified according to AKI stage and PGD grade (Figure 1A,1B).
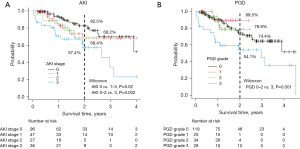
The survival rate of patients with AKI stage 0 was better than that of patients with AKI stages 1–3 (P=0.02, Figure 1A). Furthermore, the survival rate of patients with AKI stage 3 was lower than that of patients with AKI stages 0–2 (P=0.002). The survival curves of AKI 1 and 2 were almost identical, and the 2-year survival rates were 68.2% and 68.4% for AKI stages 1 and 2, respectively. PGD grades 1 and 2 did not affect survival, and only PGD grade 3 affected survival (P=0.001, Figure 1B).
HRs of complications as predictors of mortality
Univariate Cox regression analysis was performed to determine the major complications that significantly affected survival (Table 3). Ischemic events (stroke, bowel ischemia, and digital ischemia) had large HRs (Stroke, HR =3.26; bowel ischemia, HR =79.0; digital ischemia, HR =5.72); however, they were relatively rare [stroke, number (N) =6 (2.9%); bowel ischemia, N=2 (1.0%); and digital ischemia, N=6 (2.9%)]. In addition, AKI stage and dialysis during hospitalization and after discharge were found to have a significant impact on mortality (AKI ≥1, HR =1.89; dialysis during hospitalization, HR =2.41; dialysis after discharge, HR =7.27). These complications were also observed in relatively more patients than other complications [AKI ≥1, N=110 (53.4%); dialysis during hospitalization, N=29 (14.1%); dialysis after discharge, N=37 (18.0%)].
Table 3
Variable | Number | HR | P value | 95% CI |
---|---|---|---|---|
Early phase | ||||
AKI ≥1 | 110 | 1.89 | 0.04 | 1.04–3.44 |
AKI ≥2 | 63 | 2.17 | 0.008 | 1.23–3.82 |
AKI ≥3 | 36 | 2.45 | 0.003 | 1.35–4.46 |
PGD ≥1 | 87 | 1.40 | 0.25 | 0.79–2.48 |
PGD ≥2 | 62 | 1.81 | 0.04 | 1.02–3.22 |
PGD ≥3 | 28 | 2.46 | 0.005 | 1.32–4.59 |
Dialysis | 29 | 2.41 | 0.006 | 1.29–4.49 |
Stroke | 6 | 3.26 | 0.048 | 1.01–10.5 |
Bowel ischemia | 2 | 79.0 | <0.001 | 14.2–440 |
Digital ischemia | 6 | 5.72 | <0.001 | 2.03–16.1 |
Early to chronic phase | ||||
CLAD | 15 | 3.72 | 0.002 | 1.64–8.44 |
HD after discharge | 37 | 7.27 | <0.001 | 4.06–13.0 |
HR, hazard ratio; CI, confidence interval; AKI, acute kidney injury; PGD, primary graft dysfunction; CLAD, chronic lung allograft dysfunction; HD, hemodialysis.
The correlation between PGD grade and AKI stage
Of the 206 patients, 119 (57.8%) had PGD grade 0, 25 (12.1%) had PGD grade 1, 34 (16.5%) had PGD grade 2, and 28 (13.6%) had PGD grade 3. The distribution of PGD and AKI stages is summarized in Figure 2A. Sixty-nine of 119 patients (58.0%) with PGD grade 0 had AKI stage 0, and 21 of 28 patients (75.0%) with PGD grade 3 had AKI stage 3. Spearman’s test showed a significantly stronger correlation between PGD grade and AKI stage (P<0.001) (Figure 2B).
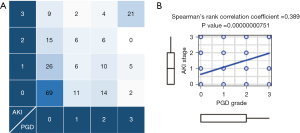
The correlation between AKI stage and GFR categories of CKD
The AKI stage was compared to the GFR category of the CKD criteria at 3, 6, 9, and 12 months after lung transplantation. At all time points, AKI stages were significantly and strongly correlated with GFR categories (P<0.001) (Figure 3). For the GFR category of CKD, missing values were found in 3 (1.5%), 24 (11.7%), 40 (19.4%), and 54 (26.2%) patients at 3, 6, 9, and 12 months after lung transplantation, with similar results in the data with missing GFR category values replaced by the LOCF method (Figure S1). In addition, linear mixed-effects models showed worsening of GFR categories at 6, 9, and 12 months compared with 1 month after lung transplantation in the entire patient cohort (Figure 4A). Similarly, in all AKI stages, the GFR categories of the CKD criteria worsened with the postoperative time (Figure 4B).
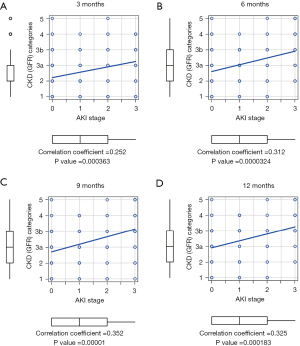
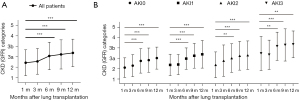
Survival after lung transplantation by GFR categories of CKD
Conditional survival curves were stratified according to the GFR category of CKD to determine whether the GFR category affected patient survival (Figure S2). However, survival curves were similar for all categories at all time points. Evidence that the GFR categories of CKD had an impact on survival was not observed in our cohort.
Discussion
Our study demonstrated a strong correlation between the clinical PGD grade and AKI stage. Previous studies have also reported a correlation between PGD and AKI (20); however, there are no reports of an association between PGD and AKI severity, except for one report related only to cystic fibrosis (21). Our study provides a clinical basis for speculating the potential causal relationship between PGD and AKI. However, it remains unclear whether both are downstream effects of other post-reperfusion systemic factors or whether only PGD is a factor in the development of AKI. As no studies have investigated the molecular epidemiology of AKI after lung transplantation, it remains to be seen whether these very high rates of clinical grade concordance reflect a novel molecular mechanism of renal injury.
Common factors post-lung transplantation such as hypoxia (15,31,32) and systemic inflammatory cytokine production (33,34) can exacerbate both lung and kidney dysfunction. Moreover, inflammatory cytokines have been reported to exacerbate PGD and AKI (15,34). One study showed that warm ischemic reperfusion with pulmonary portal clamping in rats resulted in higher plasma inflammatory cytokine levels and worsened structural kidney injury on electron microscopy compared to sham controls (35). AKI typically develops within 24 h after PGD. Therefore, elevated cytokine levels associated with lung tissue injury may be the primary trigger for AKI. Management of PGD often includes prolonged ventilation and attempts to maintain negative fluid balance, both of which can adversely affect the kidneys (18,36,37). Injurious ventilation can cause AKI, and low-tidal-volume ventilation improves mortality in patients with ARDS. The effect of mechanical ventilation during the lung transplant operation and immediately afterwards could be an important factor in the development of AKI (38-40). Conversely, increased sCr levels and decreased urine output promote fluid load, which may promote graft pulmonary edema and hypoxemia (41).
The survival rate in our cohort was decreased with AKI stage 3 by KDIGO criteria and PGD grade 3, consistent with previous reports (9,18,21,42). In addition, 75% of patients with PGD grade 3 had AKI stage 3. These results indicate that once PGD grade 3 develops, management strategies that balance lung allograft protection and kidney risks are very important, but very difficult, especially with respect to infusions and calcineurin inhibitors. It is important to avoid a significant decline in renal function while protecting lung function and recovery. AKI is a known risk factor for mortality after lung transplantation. AKI is an attractive therapeutic target because of its high incidence compared with other complications and its impact on prognosis.
This study showed that the AKI stage was strongly associated with the GFR category of the CKD criteria within one year. Furthermore, regardless of the stage of AKI, the GFR category was elevated, even within as short of a period as 1 year. This increase may be due to drug-induced renal damage caused by calcineurin inhibitors, analgesics, antibiotics, and antiviral therapies. Therefore, patients with a higher stage of AKI will have even lower renal function at one year, which further limits the use of drugs and makes the chronic phase of post-transplant management more difficult.
Previously, a few groups, including ours, have reported that surgical factors for PGD include blood transfusion volume, high FiO2 during reperfusion, high post-reperfusion systolic pulmonary artery pressure, and operative time (43-46). Central VA-ECMO has been applied in our institution instead of cardiopulmonary bypass (CPB) to reduce PGD based on evidence that ECMO has been shown to reduce intraoperative blood transfusion volume compared with CPB (47). In addition to efforts to minimize these surgical risk factors, perioperative renal protection requires appropriate management of perioperative hemodynamic changes, minimization of nephrotoxic drugs, appropriate monitoring of AKI using cystatin and other agents, and early intervention by nephrologists.
Limitations
The relatively small sample size of this study, due to the single institution, limits its generalizability. In addition, accurate diuresis records were not available retrospectively, which limited the ability to correctly classify KDIGO AKI stages. Furthermore, only the GFR category of the KDIGO diagnostic criteria for chronic renal failure was used, because albuminuria was not measured during our standardized follow-up. Therefore, it may not accurately reflect the severity of chronic renal failure.
Conclusions
We demonstrated a strong association between AKI and PGD severity after lung transplantation. Challenges to PGD grade 3 reduction with protection of renal function will improve the life expectancy and quality of life of lung transplant recipients. The direct association between PGD and AKI remains unclear and requires basic and clinical research to understand the underlying mechanisms.
Acknowledgments
The authors would like to thank Mrs. Kweli Pryor for her administrative assistance with the submission of this manuscript. A summary of this study was presented at the 43rd Annual International Society for Heart and Lung Transplantation Meeting, April 19-22, 2023, Denver, CO, USA.
Funding: This study was supported by the National Institutes of Health of the United States (Nos. HL145478, HL147290, and HL147575 to AB).
Footnote
Reporting Checklist: The authors have completed the STROBE reporting checklist. Available at https://jtd.amegroups.com/article/view/10.21037/jtd-23-256/rc
Data Sharing Statement: Available at https://jtd.amegroups.com/article/view/10.21037/jtd-23-256/dss
Peer Review File: Available at https://jtd.amegroups.com/article/view/10.21037/jtd-23-256/prf
Conflicts of Interest: All authors have completed the ICMJE uniform disclosure form (available at https://jtd.amegroups.com/article/view/10.21037/jtd-23-256/coif). AB was supported by the National Institutes of Health (Nos. HL145478, HL147290, and HL147575). The other authors have no conflicts of interest to declare.
Ethical Statement: The authors are accountable for all aspects of the work in ensuring that questions related to the accuracy or integrity of any part of the work are appropriately investigated and resolved. The study was conducted in accordance with the Declaration of Helsinki (as revised in 2013). This study was approved by the Institutional Review Board of Northwestern University (Nos. STU00207250 and STU00213616). The need for patient consent for data collection was waived by the institutional review board because this was a retrospective study.
Open Access Statement: This is an Open Access article distributed in accordance with the Creative Commons Attribution-NonCommercial-NoDerivs 4.0 International License (CC BY-NC-ND 4.0), which permits the non-commercial replication and distribution of the article with the strict proviso that no changes or edits are made and the original work is properly cited (including links to both the formal publication through the relevant DOI and the license). See: https://creativecommons.org/licenses/by-nc-nd/4.0/.
References
- Snell GI, Yusen RD, Weill D, et al. Report of the ISHLT Working Group on Primary Lung Graft Dysfunction, part I: Definition and grading-A 2016 Consensus Group statement of the International Society for Heart and Lung Transplantation. J Heart Lung Transplant 2017;36:1097-103. [Crossref] [PubMed]
- Daud SA, Yusen RD, Meyers BF, et al. Impact of immediate primary lung allograft dysfunction on bronchiolitis obliterans syndrome. Am J Respir Crit Care Med 2007;175:507-13. [Crossref] [PubMed]
- Kreisel D, Krupnick AS, Puri V, et al. Short- and long-term outcomes of 1000 adult lung transplant recipients at a single center. J Thorac Cardiovasc Surg 2011;141:215-22. [Crossref] [PubMed]
- Lertjitbanjong P, Thongprayoon C, Cheungpasitporn W, et al. Acute Kidney Injury after Lung Transplantation: A Systematic Review and Meta-Analysis. J Clin Med 2019;8:1713. [Crossref] [PubMed]
- Jing L, Chen W, Guo L, et al. Acute kidney injury after lung transplantation: a narrative review. Ann Transl Med 2021;9:717. [Crossref] [PubMed]
- Rocha PN, Rocha AT, Palmer SM, et al. Acute renal failure after lung transplantation: incidence, predictors and impact on perioperative morbidity and mortality. Am J Transplant 2005;5:1469-76. [Crossref] [PubMed]
- George TJ, Arnaoutakis GJ, Beaty CA, et al. Acute kidney injury increases mortality after lung transplantation. Ann Thorac Surg 2012;94:185-92. [Crossref] [PubMed]
- Jacques F, El-Hamamsy I, Fortier A, et al. Acute renal failure following lung transplantation: risk factors, mortality, and long-term consequences. Eur J Cardiothorac Surg 2012;41:193-9. [PubMed]
- Fidalgo P, Ahmed M, Meyer SR, et al. Incidence and outcomes of acute kidney injury following orthotopic lung transplantation: a population-based cohort study. Nephrol Dial Transplant 2014;29:1702-9. [Crossref] [PubMed]
- Balci MK, Vayvada M, Salturk C, et al. Incidence of Early Acute Kidney Injury in Lung Transplant Patients: A Single-Center Experience. Transplant Proc 2017;49:593-8. [Crossref] [PubMed]
- Nguyen AP, Gabriel RA, Golts E, et al. Severity of Acute Kidney Injury in the Post-Lung Transplant Patient Is Associated With Higher Healthcare Resources and Cost. J Cardiothorac Vasc Anesth 2017;31:1361-9. [Crossref] [PubMed]
- Kim NE, Kim CY, Kim SY, et al. Risk factors and mortality of acute kidney injury within 1 month after lung transplantation. Sci Rep 2021;11:17399. [Crossref] [PubMed]
- Hoste EA, Bagshaw SM, Bellomo R, et al. Epidemiology of acute kidney injury in critically ill patients: the multinational AKI-EPI study. Intensive Care Med 2015;41:1411-23. [Crossref] [PubMed]
- Cheungpasitporn W, Kashani K. Electronic Data Systems and Acute Kidney Injury. Contrib Nephrol 2016;187:73-83. [Crossref] [PubMed]
- Ishikawa S, Griesdale DE, Lohser J. Acute kidney injury within 72 hours after lung transplantation: incidence and perioperative risk factors. J Cardiothorac Vasc Anesth 2014;28:931-5. [Crossref] [PubMed]
- Kellum JA, Prowle JR. Paradigms of acute kidney injury in the intensive care setting. Nat Rev Nephrol 2018;14:217-30. [Crossref] [PubMed]
- Puttarajappa CM, Bernardo JF, Kellum JA. Renal Complications Following Lung Transplantation and Heart Transplantation. Crit Care Clin 2019;35:61-73. [Crossref] [PubMed]
- Atchade E, Barour S, Tran-Dinh A, et al. Acute Kidney Injury After Lung Transplantation: Perioperative Risk Factors and Outcome. Transplant Proc 2020;52:967-76. [Crossref] [PubMed]
- Meyer DM, Bennett LE, Novick RJ, et al. Effect of donor age and ischemic time on intermediate survival and morbidity after lung transplantation. Chest 2000;118:1255-62. [Crossref] [PubMed]
- Shashaty MGS, Forker CM, Miano TA, et al. The association of post-lung transplant acute kidney injury with mortality is independent of primary graft dysfunction: A cohort study. Clin Transplant 2019;33:e13678. [Crossref] [PubMed]
- Scaravilli V, Merrino A, Bichi F, et al. Longitudinal assessment of renal function after lung transplantation for cystic fibrosis: transition from post-operative acute kidney injury to acute kidney disease and chronic kidney failure. J Nephrol 2022;35:1885-93. [Crossref] [PubMed]
- Sawhney S, Marks A, Fluck N, et al. Post-discharge kidney function is associated with subsequent ten-year renal progression risk among survivors of acute kidney injury. Kidney Int 2017;92:440-52. [Crossref] [PubMed]
- Chambers DC, Cherikh WS, Harhay MO, et al. The International Thoracic Organ Transplant Registry of the International Society for Heart and Lung Transplantation: Thirty-sixth adult lung and heart-lung transplantation Report-2019; Focus theme: Donor and recipient size match. J Heart Lung Transplant 2019;38:1042-55. [Crossref] [PubMed]
- Chawla LS, Amdur RL, Amodeo S, et al. The severity of acute kidney injury predicts progression to chronic kidney disease. Kidney Int 2011;79:1361-9. [Crossref] [PubMed]
- Kanda Y. Investigation of the freely available easy-to-use software 'EZR' for medical statistics. Bone Marrow Transplant 2013;48:452-8. [Crossref] [PubMed]
- Kidney Disease: Improving Global Outcomes (KDIGO) Acute Kidney Injury Work Group. KDIGO Clinical Practice Guideline for Acute Kidney Injury. Kidney International Supplements 2012;2:1-138.
- Kidney Disease: Improving Global Outcomes (KDIGO) CKD Work Group. KDIGO 2012 Clinical Practice Guideline for the Evaluation and Management of Chronic Kidney Disease. Kidney International Supplements 2013;3:1-150.
- Levey AS, Stevens LA, Schmid CH, et al. A new equation to estimate glomerular filtration rate. Ann Intern Med 2009;150:604-12. [Crossref] [PubMed]
- Verleden GM, Glanville AR, Lease ED, et al. Chronic lung allograft dysfunction: Definition, diagnostic criteria, and approaches to treatment-A consensus report from the Pulmonary Council of the ISHLT. J Heart Lung Transplant 2019;38:493-503. [Crossref] [PubMed]
- Kurihara C, Walter JM, Karim A, et al. Feasibility of Venovenous Extracorporeal Membrane Oxygenation Without Systemic Anticoagulation. Ann Thorac Surg 2020;110:1209-15. [Crossref] [PubMed]
- Conde E, Alegre L, Blanco-Sánchez I, et al. Hypoxia inducible factor 1-alpha (HIF-1 alpha) is induced during reperfusion after renal ischemia and is critical for proximal tubule cell survival. PLoS One 2012;7:e33258. [Crossref] [PubMed]
- Wu G, Xu G, Chen DW, et al. Hypoxia Exacerbates Inflammatory Acute Lung Injury via the Toll-Like Receptor 4 Signaling Pathway. Front Immunol 2018;9:1667. [Crossref] [PubMed]
- Hoke TS, Douglas IS, Klein CL, et al. Acute renal failure after bilateral nephrectomy is associated with cytokine-mediated pulmonary injury. J Am Soc Nephrol 2007;18:155-64. [Crossref] [PubMed]
- Bihorac A, Baslanti TO, Cuenca AG, et al. Acute kidney injury is associated with early cytokine changes after trauma. J Trauma Acute Care Surg 2013;74:1005-13. [Crossref] [PubMed]
- Xiang BQ, Gao H, Luo ZY, et al. Effect of dexmedetomidine on renal injury induced by lung ischemia/reperfusion in mice. Zhongguo Ying Yong Sheng Li Xue Za Zhi 2017;33:380-4. [PubMed]
- Prowle JR, Echeverri JE, Ligabo EV, et al. Fluid balance and acute kidney injury. Nat Rev Nephrol 2010;6:107-15. [Crossref] [PubMed]
- Hepokoski ML, Malhotra A, Singh P, et al. Ventilator-Induced Kidney Injury: Are Novel Biomarkers the Key to Prevention? Nephron 2018;140:90-3. [Crossref] [PubMed]
- Si MK, Mitaka C, Tulafu M, et al. Inhibition of poly (adenosine diphosphate-ribose) polymerase attenuates lung-kidney crosstalk induced by intratracheal lipopolysaccharide instillation in rats. Respir Res 2013;14:126. [Crossref] [PubMed]
- Kapoor K, Singla E, Sahu B, et al. PARP inhibitor, olaparib ameliorates acute lung and kidney injury upon intratracheal administration of LPS in mice. Mol Cell Biochem 2015;400:153-62. [Crossref] [PubMed]
- Hegeman MA, Hennus MP, Heijnen CJ, et al. Ventilator-induced endothelial activation and inflammation in the lung and distal organs. Crit Care 2009;13:R182. [Crossref] [PubMed]
- Basu RK, Wheeler D. Effects of ischemic acute kidney injury on lung water balance: nephrogenic pulmonary edema? Pulm Med 2011;2011:414253. [Crossref] [PubMed]
- Christie JD, Bellamy S, Ware LB, et al. Construct validity of the definition of primary graft dysfunction after lung transplantation. J Heart Lung Transplant 2010;29:1231-9. [Crossref] [PubMed]
- Diamond JM, Lee JC, Kawut SM, et al. Clinical risk factors for primary graft dysfunction after lung transplantation. Am J Respir Crit Care Med 2013;187:527-34. [Crossref] [PubMed]
- Weber D, Cottini SR, Locher P, et al. Association of intraoperative transfusion of blood products with mortality in lung transplant recipients. Perioper Med (Lond) 2013;2:20. [Crossref] [PubMed]
- Toyoda T, Cerier EJ, Manerikar AJ, et al. Recipient, donor, and surgical factors leading to primary graft dysfunction after lung transplant. J Thorac Dis 2023;15:399-409. [Crossref] [PubMed]
- Cerier E, Manerikar A, Kandula V, et al. Postreperfusion Pulmonary Artery Pressure Indicates Primary Graft Dysfunction After Lung Transplant. Ann Thorac Surg 2022;S0003-4975(22)01549-1.
- Magouliotis DE, Tasiopoulou VS, Svokos AA, et al. Extracorporeal membrane oxygenation versus cardiopulmonary bypass during lung transplantation: a meta-analysis. Gen Thorac Cardiovasc Surg 2018;66:38-47. [Crossref] [PubMed]