Role of interleukin-17A in early graft rejection after orthotopic lung transplantation in mice
Introduction
Lung transplantation is an accepted therapeutic option for selected patients with end-stage lung disease. However, allograft rejection represents a major cause of morbidity and mortality after lung transplantation. A report from the registry of the International Society for Heart and Lung Transplantation indicated that 28.9% of adult primary lung recipients had at least one episode of treated acute rejection between discharge and 1-year follow-up, and 50% of primary adult lung transplant recipients developed obliterative bronchiolitis (OB) within 5 years of transplantation (1). Although immunosuppressive regimens can efficiently control acute rejection (2), two main problems impact recipients’ survival. First, primary graft dysfunction (PGD) that occurs during the immediate postoperative period is a severe form of ischemia/reperfusion injury (IRI). Severe PGD is associated with up to 40% mortality within 30 days after transplantation (3). Secondly, chronic rejection-OB is a fibroproliferative disease affecting the small airways. Previous research has shown that PGD is associated with a greater risk of OB (4), and histopathologic reports indicate that both inflammation and injury precede aberrant tissue repair and epithelial regeneration that lead to the fibrous disruption of the small airways that occurs during OB (5).
Lung allograft rejection is associated with the production of a multitude of cytokines. Interleukin (IL)-17, which is involved in both innate and adaptive immunity, has six known isoforms (A, B, C, D, E, and F), and IL-17A has been shown to play a key role in the pathogenesis of alloimmune-induced autoimmunity post lung transplantation (6). Indeed, cells responsible for both innate and adaptive immunity can produce IL-17A and may therefore generate graft lesions at different time points after transplantation (7). IL-17 isoforms are known to activate inflammatory, endothelial, and epithelial cells and to induce the production of a variety of proinflammatory cytokines, chemokines, and adhesion molecules (8). High amounts of IL-17A were found in bronchoalveolar lavage from lung transplant recipients who experienced PGD or OB, suggesting the involvement of IL-17A in lung both PGD and OB (9,10). Studies in rodents have demonstrated pathogenic roles for IL-17A in an in situ lung IRI model (11), a heterotopic trachea allotransplantation model (12), and in the development of OB after lung transplantation with minor histocompatibility mismatch (13). However, the relative importance of IL-17A’s action in mediating tissue damages during the chronological stages of transplant rejection remains poorly understood in the case of full major histocompatibility complex (MHC) mismatch. Additional research is needed to determine the mechanisms by which IRI, PGD, and environmental and microbial responses may promote the secretion of IL-17A from T helper 17 (Th17) cells and other lymphocytes. The previously identified pleiotropic effects of IL-17A provide compelling reasons for further research into the potential of targeting the IL-17A pathway to affect outcomes of lung transplantation.
A better understanding of the cellular and molecular mechanisms of lung graft injury will be critical to improving survival among lung transplant recipients. The specific aim of this study was to investigate the role of IL-17A during early rejection in a fully MHC mismatched mouse model of lung transplantation. We used the orthotopic lung transplantation model described by Okazaki et al. (14), which successfully recreates acute allograft rejection, and investigated the interplay between the innate and adaptive immune responses. This model has become an accepted approach for researching the immunopathology of lung transplant rejection.
Methods
Animals
Pathogen-free male mice C57BL/6 (n=95) and BALB/c (n=45) were purchased from Weikang-Lihua Company (Beijing, China). All animals were maintained in the Laboratory Animal Resource Center at Capital Medical University in accordance with institutional guidelines. Mice 10−12 weeks of age and 25−30 g were used as both donors and recipients. Weight-matched mice were randomly assigned to the five treatment groups (5 per group and time point): isograft recipients (isograft group; n=5+15, 5 for functional assessment of grafts), allograft recipients (allograft group; n=15), allograft recipients treated with IL17A-neutralizing antibody (Ab) (allograft + Ab group; n=15); allograft recipients treated with an IgG control Ab (allograft + IgG group; n=15); and control lungs (control group; n=5+5, 5 for functional assessment of native lung, 5 for cytokine measurement and flow cytometric analysis). All studies were approved by the Laboratory Animal Resource Center at Capital Medical University.
Orthotopic lung transplantation
Orthotopic lung transplantation, utilizing the cuff technique, was performed according to the method of Okazaki (14). Syngeneic transplants were performed in the C57BL/6→C57BL/6 strain combination, and allogeneic transplants were performed in the Balb/c→C57BL/6 strain combination. All surgical procedures were performed utilizing aseptic techniques. Both harvesting and transplantation operations were performed under a stereoscope microscope (SZ61, Olympus, Japan) with 6.7−45X (SZ51_8−40X) magnification. Donors and recipients were anesthetized with pentobarbital sodium (50 mg/kg) administered by intraperitoneal injection prior to surgery.
Donor procedure
After induction of anesthesia, donor mice were orotracheally intubated with a 20-guage catheter under direct vision and ventilated (Harvard Rodent Ventilator, model 687; Harvard Apparatus, Holliston, MA, USA) with room air at 125 breaths/minute and a tidal volume of 0.5 mL. Median laparosternotomy was performed to expose the thoracic and peritoneal cavity after sterilization. The graft lungs were flushed though the main pulmonary artery (PA) with Perfadex solution (Vitrolife, Inc., Goteberg, Sweden) after heparinization. Subsequently, the heart−lung block was harvested with the lungs inflated at 50% of total lung capacity. The left lung was then isolated and prepared for cuff placement. Briefly, the pulmonary vein (PV) cuff was made from a 22-gauge intravenous catheter (Terumo Medical Corporation, Somerset, NJ, USA), the PA cuff was a 24-gauge catheter, and the bronchial cuff was a 20-gauge catheter. The distal ends of the PV and PA were passed through the special cuffs, and the ends were everted over the cuff and then firmly fixed with a circumferential 10−0 silk suture (Shanghai Pudong Jinhuan Medical Products Co., Ltd., Shanghai, China). The donor lung was wrapped in Perfadex-soaked gauze and stored at 4 °C until transplantation. The bronchial cuff was placed immediately prior to implantation.
Recipient procedure
The procedures used for anesthesia induction and tracheal intubation in the recipients mice were the same as those used for donor mice. A thoracotomy was performed through the left fifth intercostal space, and the left lung hilum structure was carefully dissected. The PV and PA were clamped with a microvascular clamp or occluded with silk suture with a slip knot. Then a small U-shaped incision of approximately one-third of the circumference of the vessel or bronchus was made for insertion of the cuff. The donor lung was positioned in the thoracic cavity of the recipient. Then the cuffs were inserted into the recipient PV, bronchus, and PA and secured with 10−0 silk sutures. The hilar clamp or silk knot was removed, and then the graft was re-perfused. The thoracotomy incision was closed, with a 20-gauge catheter attached to a syringe inserted into the thoracotomy. The mice recovered from anesthesia approximately one hour later, and then the chest drainage catheter and tracheal intubation were removed. No mice were given any immunosuppressive agents.
Ab treatment
For mice that received anti-IL17A treatment, based on a dosage described previously (12), 200 µg of neutralizing anti-IL17A (BioXcell, West Lebanon, NH, USA, cloneMM17F3) or IgG (BioXcell) control was injected intraperitoneally starting the day of transplantation and continuing with one injection every other day after transplantation for a total of four injections per recipient.
Functional assessment of grafts
Seven days after transplantation, four syngeneic transplantation C57BL/6→C57BL/6 recipient mice were subjected to anesthesia, tracheotomy, and intubation. Then the recipients underwent a thoracotomy, and the right pulmonary hilum was occluded for 10 minutes. Subsequently, the lung graft function was assessed based on arterial blood gases (ABL-700 blood gas analyzer, Radiometer, Copenhagen, Denmark) on a FiO2 of 21%, drawn by left ventricular puncture. The same procedure was performed in five wild-type C57BL/6 mice that served as controls.
Histologic analysis
Immediately prior to graft retrieval, recipient mice were sacrificed by cervical dislocation. Lung grafts from the isograft, allograft, allograft + Ab, and allograft + IgG groups were harvested on postoperative day (POD) 3, 7, or 28. Cross-sectional specimens were fixed in 4% formaldehyde, embedded in paraffin, sectioned at 5-µm thickness, and stained with hematoxylin-eosin and Masson’s Trichrome staining.
The specimens were examined for signs of graft rejection. Pathological grading of rejection was conducted in a blinded fashion using standard criteria for clinical lung transplantation (15). Scoring was focused on the severity of vascular lesions (“A” scores): A0 = no acute rejection, A1 = minimal acute rejection, A2 = mild acute rejection, A3 = moderate acute rejection and A4 = severe acute rejection. Because airway involvement during the acute rejection response parallels that observed in the vasculature, “B” scores (for airways) were not recorded. Finally, OB was defined by the presence of partial or complete luminal occlusion due to dense eosinophilic hyaline fibrosis in the sub-mucosa of membranous and respiratory bronchioles.
Cytokine profiling by quantitative real-time polymerase chain reaction (RT-PCR)
Three and seven days after transplantation, total RNA was extracted from lung grafts and normal lungs (as controls) using TRIzol reagent (Invitrogen, Carlsbad, CA, USA) according to manufacturers’ instructions. cDNA was reverse transcribed using the ProtoScript II Fist Strand cDNA Synthesis Kit (New England Biolabs, Ipswich, MA, USA). The following primers for IL-17A, IL-6, IL-22, interferon (IFN)-γ, and cyclophilin A were synthesized by Invitrogen: IL-17A: forward 5’-CTC CAG AAG GCC CTC AGA CTA-3’, reverse 5’-AGC TTT CCC TCC GCA TTG ACA-3’; IL-6: forward 5’-GTC ACA GAA GGA GTG GCT A-3’, reverse 5’-AGA GAA CAA CAT AAG TCA GAT ACC-3’; IL-22: forward 5’-CGC TGC CCG TCA ACA CCC GG-3’, reverse 5’-CTG ATC CTT AGC ACT GAC TCC TCG-3’; IFN-γ: forward 5’-TGG CTC TGC AGG ATT TTC ATG-3’, reverse 5’-TCA AGT GGC ATA GAT GTG GAA GAA-3’; and cyclophilin A: forward 5’-AGG GTG GTG ACT TTA CAC GC-3’, reverse 5’-ATC CAG CCA TTC AGT CTT GG-3’. Each reaction was performed with the Roter gene Q system (Qiagen, Valencia, CA, USA) and the SYBR Green PCR kit (Qiagen). All experiments were performed at least three times. The cyclophilin A gene was used as a housekeeping gene. Graft mRNA levels were expressed as 2−ΔΔCT, in which CT represents the “cycle threshold”, ΔΔCT = ΔCTgraft lung − ΔCTcontrol lung, and nCT = CTgene of interest − CTcyclophilin A.
Statistical analyses
Statistical analyses were performed with SPSS19.0 for Windows software (SPSS, Inc., Chicago, IL, USA), and the data plots were prepared using GraphPad Prism 5 (GraphPad Software, La Jolla, CA, USA). Unless noted otherwise, data expressed as mean ± standard deviation (SD) values. Comparisons between two groups were performed using independent sample t tests, and multiple comparisons were performed using one-way analysis of variance (ANOVA) and least significant difference (LSD) analysis. P<0.05 was considered statistically significant.
Results
Surgical outcomes
After 6 months of practicing the lung transplantation procedure, we had basically resolved all technical problems. To assess the functional status of lung grafts in our pilot studies, we initially conducted five syngeneic transplantations. The PaO2 levels of C57BL/6 recipients of syngeneic grafts were measured 7 days after transplantation and compared to those in wild-type C57BL/6 mice in which arterial blood was also drawn after 10-min occlusion of the right pulmonary hilum. The PaO2 level in recipients of syngeneic grafts did not differ from that in the wild-type c57BL/6 mice (P=0.100; Figure 1).
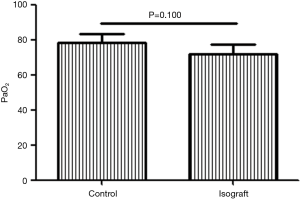
For the present study, we conducted 60 lung transplantations with an 87% technical success rate; 52 of 60 recipient mice survived at the time of graft harvesting at POD days 3, 7, and 28. One recipient died of intraoperative bleeding (allograft group); two recipients died of intraoperative venous tearing (isograft group and allograft + Ab group); one recipient died of tension pneumothorax approximately 1 hour after extraction of the chest tube (allograft group); three recipients died from venous torsion and venous thrombosis due to cuff-related problems on POD day 2 (allograft group and allograft + IgG group); and one recipient died from severe lung and pleural cavity infection 9 days after transplantation (allograft + Ab group). The whole operation time was 162.3±22.2 minutes. The mean warm ischemia time was 19.7±3.2 minutes, and the mean cold ischemia time was 96.3±10.5 minutes for the 57 mice who survived the operation.
Gross evaluation and histologic analysis of lung grafts
Gross evaluation of the harvested isografts showed slight edema at 3 days after transplantation, whereas isograft lungs appeared normal at both 7 and 28 days after transplantation. In contrast, harvested lungs from the allograft group showed mild hyperemia and edema at 3 days after transplantation, marked edema after 7 days, and fibrosis and consolidation after 28 days (Figure 2). Lungs removed from mice in the allograft + Ab and allograft + IgG groups appeared similar to those in the allograft group at each time point (not shown).
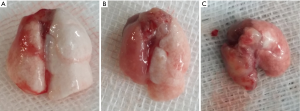
Lung grafts harvested at different time points after transplantation was examined histopathologically to assess the intensity of the cellular infiltrate and the presence or absence of fibrosis (Figure 3). Lung grafts from the isograft group showed a normal histological appearance with minimal infiltration of T lymphocytes, essentially no inflammation was observed in the vessels and airways at 7 and 28 days after transplantation. By contrast, circumferential infiltration of the perivascular interstitium by mononuclear cells and alveolar hemorrhage was observed on POD 3 in lung grafts from the allograft group. By POD 7, the allografts showed acute cellular rejection with extensive perivascular and interstitial mononuclear cell infiltration, and small bronchioles were associated with peribronchiolar mononuclear infiltrates. By POD 28, atrophy and consolidation were observed in the allografts as well as perivascular and alveolar septal mononuclear cell infiltration, and fibrotic changes were observed in the peribronchiolar tissues along with airway blockage in some recipients. Some damage to the overlying epithelium was observed in addition to apoptosis and distortion of the airway lumen. Two of the three (67%) studied grafts demonstrated OB. Allografts from mice treated with anti-IL17A (allograft + Ab group) showed similar lymphocyte infiltration compared to that in lungs from the allograft group at POD 3, but by POD 7, considerably less perivascular and interstitial mononuclear infiltration was observed in these grafts. Inflammatory cells were observed in the airways in some grafts of the allograft + Ab group, but no fibrosis was detected, indicating the absence of OB. However, by POD 28, the grafts also demonstrated signs of severe rejection similar to those in the allograft group, and 50% of grafts (2/4) demonstrated OB. The histologic features of allografts from mice treated with IgG closely matched those of the allograft group at all time points post-transplantation (not shown).
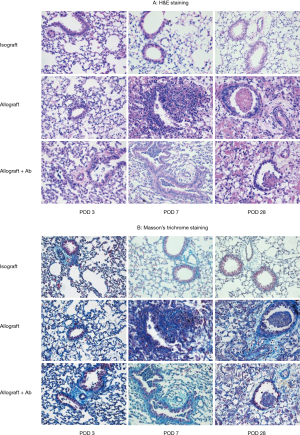
Histologic grading of the four groups of grafts revealed varying degrees of an acute rejection response on POD 3, but lesions were more obvious in allografts than in isografts (Figure 4). No histologic lesions were routinely detectable in the isograft lungs at POD 7 or 28, nor were OB detected in isografts post-transplantation. All allografts in in the allograft and allograft + IgG groups exhibited severe acute rejection (Grade A3−A4) at PODs 7 and 28 (Figure 4). In contrast, mild rejection (Grade A2, Figure 4) was detected on POD 7 in the allograft + Ab group, but this outcome progressed to severe rejection by POD 28 (Grade A3−A4, Figure 4). Although OB was not detected on POD 7 in any of the allograft groups, lesions characteristic of OB were observed in approximately 64% (7/11) of all allografts at POD 28.
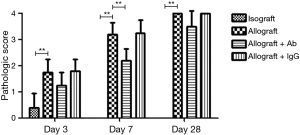
Cytokine profiles
To investigate the role of IL-17A in lung allograft rejection, we examined the expression of IL-17A and related proinflammatory cytokines in lung grafts at different time points after transplantation. Compared to the level in control lungs, IL17-A mRNA expression in isografts and allografts was significantly upregulated on PODs 3 and 7, but IL-17A expression in allografts had increased more than 20-fold by POD 7 compared to a 5-fold increase observed in isografts on POD 7. Moreover, allografts exhibited significantly greater IL-17A transcript levels compared to isografts at both PODs 3 and 7 (Figure 5A).
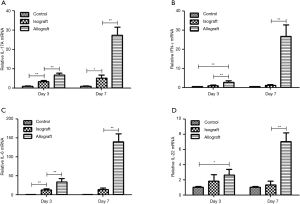
The IFN-γ mRNA transcript level was significantly greater in allografts compared to control lungs or isografts at PODs 3 and 7, whereas no difference in IFN-γ mRNA expression was detected between isografts and control lungs on PODs 3 and 7 (Figure 5B). Although IL-6 mRNA expression was significantly greater in isografts than in control lungs on POD 3, IL-6 mRNA transcripts were significantly upregulated in allografts compared to control lungs or isografts on PODs 3 and 7 (Figure 5C). Compared to that in control lungs, IL-22 mRNA expression in allografts was significantly greater on PODs 3 and 7, and allografts exhibited significantly greater IL-22 mRNA expression than isografts at POD 7. IL-22 mRNA expression did not differ between the control lungs and the isograft group at either POD 3 or day 7 (Figure 5D).
Discussion
Animal model of lung transplantation
Rejection is a serious complication after lung transplantation that occurs too frequently. We choose a fully MHC mismatched murine model of orthotopic lung transplantation model for two reasons: this murine model permits full use of knockout or transgenic technology in further studies, and there has been no similar research with a fully MHC mismatched murine model. Using this model, we demonstrated an important role for IL-17A in the development of early post-transplantation lung lesions. IL-17A mRNA levels were much higher in allografts than in isografts, and an early (7 days) protective effect of IL-17A neutralization was observed in allogeneic conditions.
Graft rejection and pathology
PGD and OB are the leading causes of morbidity and mortality after lung engraftment, and the frequency and severity of acute rejection episodes are known to be associated with an increased risk of OB (16). Acute rejection is characterized by a temporary perivascular and peribronchiolar infiltration of lymphocytes, and OB is characterized by persistent airway neutrophilia that leads to airway fibrosis and obliteration (17). It is possible that both acute rejection and OB represent a continuum of inflammatory events, starting with macrophage activation, followed by early lymphocyte activation and neutrophil migration. Because IL-17 promotes neutrophil chemotaxis (18), the presence of neutrophils may be secondary to an alloimmune response mediated by IL-17 producing cells.
Peribronchiolar fibrosis and fibrotic plugs are histologic hallmarks of OB (15). Therefore, Masson’s trichrome staining was used to evaluate the presence of fibrosis in graft lesions. In the present study, on gross inspection and histologically, the isografts healed rapidly and recovered a normal structure by POD 7. In contrast, the allografts showed signs of severe acute rejection at POD 7 with evidence of necrosis, but no evidence of OB. Moreover, the allograft group treated with anti-IL-17A demonstrated obviously alleviated rejection on POD 7. Independent of Ab treatment though, the allografts became generally fibrotic with loss of normal lung structures by POD 28, and OB was noted on POD 28 in the majority (7/11) of mice in the allograft, allograft + Ab, and allograft + IgG groups.
Relation between IL-17A and rejection
IL-17 has been linked to the development of acute and chronic rejection after lung transplantation in both animal models and humans. Verleden and colleagues found that increased IL-17 is associated with increased numbers of lymphocytes and neutrophils in bronchoalveolar lavage during acute rejection after clinical lung transplantation, and IL-17 levels also are positively correlated with the severity of rejection (19). However, although another study found increased numbers of IL-17–positive cells in endobronchial biopsies early after lung transplantation, no correlation was found between the number of IL-17−positive cells and the severity of acute rejection (20). The discrepancies in these findings suggest the mechanism of lung graft rejection mediated by IL-17-positive cells should be further research.
Because previous reports demonstrated a possible link between IL-17A and graft rejection and that the progression of OB lesions occurs over time, we also evaluated IL-17A lung mRNA expression in isografts versus allografts. Our data suggest a temporal pattern of IL-17A expression that corresponds to the presence of rejection. To further confirm the role of IL-17A in the development of early post-transplantation lesions, we treated mice that received allografts with an Ab to IL-17A, and that obviously alleviated the lung grafts inflammatory stress and lymphocyte infiltration. We speculate that it is the reason for anti-IL17A to reduce rejection POD 7 in our experiment.
Potential mechanism of rejection involving IL-17A
IL-17 is known to be capable of inducing fibroblast cytokine secretion and proliferation, as well as stromal cell differentiation, making it an attractive target for mediating chronic rejection (21-23). Because IL-17 induces stromal cells and monocytes to produce cytokines and promotes granulopoiesis, it has long been recognized as a possible target for therapy during lung transplantation specifically. Several studies have linked IL-17 to fibrosis in the lung in mouse models of pulmonary fibrosis as well as to idiopathic pulmonary fibrosis in humans (22,24,25). IL-17 may be a key regulator of the airway fibrotic response that drives the development of OB. Moreover, OB has been linked to local neutrophilic infiltration, and IL-17 is known to promote recruitment and differentiation of neutrophils. Thus, IL-17 production by autoreactive T cells may in part explain the pathogenesis of bronchoalveolar lavage neutrophilia observed in OB (19).
Furthermore, immune recognition of non-histocompatibility antigens, such as the autoantigens type V collagen and tubulin, which has been implicated in the pathogenesis of OB in patients and pre-clinical models (6,26), may have contributed to the onset of OB in the current study. Collagen V and donor antigens have been shown to induce IL-17 secretion (13), with one study specifically demonstrating that collagen V induces IL-17 production in clinical OB (6). IL-17 may also play a role in alloantibody and autoantibody production, given that IL-17 has been implicated in the development of germinal centers and autoantibody production in autoimmune disease (27).
Interestingly, the macrolide, azithromycin, an important therapeutic agent for a subset of patients with OB, has previously been found to suppress IL-17—induced IL-8 production from human smooth muscle cells, providing further support for a role of IL-17 in OB (28). Neutralization of IL-17 beyond the effects of azithromycin may represent a promising therapy for lung transplantation patients, and treatments to block IL-17 or its receptor are currently in trials for autoimmune diseases (29) and hold promise for complications of lung transplantation.
Expression of the IL-17A−related cytokines IFN-γ, IL-6, and IL-22
IFN-γ, which has often been linked to rejection responses, was found to be upregulated in allografts after transplantation in our study. Graft-infiltrating T lymphocytes in both isografts and allografts have the capacity to produce IFN-γ, and the level of IFN-γ expression was higher in T cells in allografts than in isografts. IL-17 could enhance early neutrophil influx into allografts thus facilitating further recruitment of pathogenic IFN-γ-producing T cells into the grafts (30). Neutralizing IL-17 receptor-immunoglobulin fusion protein could reduce intragraft production of IFN-γ (31). Interestingly, a previous study showed that IFN-γ deficiency enhances graft damage, supporting an unexpected regulatory role for this cytokine (32). Further investigations are required to address the complex, coordinated regulation of IL-17 and IFN-γ expression in the development of graft rejection. In addition, sustained allorecognition of multiple MHC disparities may induce IFN-γ−producing Th1 cells and cytotoxic T lymphocyte responses, which might mask the early IL-17A-dependent mechanisms (33).
IL-6 is produced by multiple cell types and is a pleiotropic proinflammatory cytokine. IL-17 can induce IL-6 production in various types of cells (34), and IL-6 is not only a proinflammatory cytokine, but also can inhibit regulatory T cell (Treg) differentiation (35). Tregs are potent immunosuppressive cells that have been shown to prolong allograft survival (36). Inhibition of Tregs via increased IL-6 production may represent another pathway of rejection caused by IL-17, and this has been demonstrated previously in rodent heart graft rejection (37).
Vγ4 + γδT cells can mediate inflammation by secreting IL-17A, thus causing lung injury and fibrosis (38). Vγ6 + γδT cells of the lung can express IL-22, which can inhibit inflammation and lung injury, and protect against lung fibrosis (39). In the present study, IL-22 expression was significantly greater in allografts than in isografts, following a pattern similar to that of IL-17A expression. It is possible that IL-22 may neutralize some of the effects of IL-17A in allografts, and thus, immunotherapy directed toward increasing IL-22 levels in allografts may be beneficial in preventing OB.
Study limitations
At POD 28, all allografts in our study exhibited severe rejection, and we even observed destruction of parts of the lung tissue structure. The significant difference in rejection scores observed between grafts from the allograft and allograft + Ab groups at POD 7 was no longer significant at POD 28 (P=0.053, Figure 4). Therefore, our model may not be appropriate for long-term observation post-transplantation. Similarly, IL-17A deficiency alone did not prevent fibrosis, confirming the existence of multiple overlapping fibrotic pathways (40). Because this work focused on the role of IL-17A, we could not rule out a potential effect for the homologous IL-17F. Indeed, cells that produce IL-17A can also secrete IL-17F, and both bind the IL-17 receptor as homodimers or heterodimers (41).
Conclusions
In summary, our data directly implicated IL-17A as a pro-inflammatory mediator of early lung graft rejection, and thus, IL-17A may represent a potential therapeutic target for preventing rejection in clinical lung transplantation.
Acknowledgements
Funding: This work was supported by grants from the Beijing Natural Science Foundation (kz201310025019).
Footnote
Conflicts of Interest: The authors have no conflicts of interest to declare.
References
- Yusen RD, Edwards LB, Kucheryavaya AY, et al. The registry of the International Society for Heart and Lung Transplantation: thirty-first adult lung and heart-lung transplant report--2014; focus theme: retransplantation. J Heart Lung Transplant 2014;33:1009-24. [Crossref] [PubMed]
- Lodhi SA, Lamb KE, Meier-Kriesche HU. Solid organ allograft survival improvement in the United States: the long-term does not mirror the dramatic short-term success. Am J Transplant 2011;11:1226-35. [Crossref] [PubMed]
- Christie JD, Bellamy S, Ware LB, et al. Construct validity of the definition of primary graft dysfunction after lung transplantation. J Heart Lung Transplant 2010;29:1231-9. [Crossref] [PubMed]
- Daud SA, Yusen RD, Meyers BF, et al. Impact of immediate primary lung allograft dysfunction on bronchiolitis obliterans syndrome. Am J Respir Crit Care Med 2007;175:507-13. [Crossref] [PubMed]
- Estenne M, Hertz MI. Bronchiolitis obliterans after human lung transplantation. Am J Respir Crit Care Med 2002;166:440-4. [Crossref] [PubMed]
- Burlingham WJ, Love RB, Jankowska-Gan E, et al. IL-17-dependent cellular immunity to collagen type V predisposes to obliterative bronchiolitis in human lung transplants. J Clin Invest 2007;117:3498-506. [Crossref] [PubMed]
- Onishi RM, Gaffen SL. Interleukin-17 and its target genes: mechanisms of interleukin-17 function in disease. Immunology 2010;129:311-21. [Crossref] [PubMed]
- Kolls JK, Lindén A. Interleukin-17 family members and inflammation. Immunity 2004;21:467-76. [Crossref] [PubMed]
- Vanaudenaerde BM, De Vleeschauwer SI, Vos R, et al. The role of the IL23/IL17 axis in bronchiolitis obliterans syndrome after lung transplantation. Am J Transplant 2008;8:1911-20. [Crossref] [PubMed]
- Bobadilla JL, Love RB, Jankowska-Gan E, et al. Th-17, monokines, collagen type V, and primary graft dysfunction in lung transplantation. Am J Respir Crit Care Med 2008;177:660-8. [Crossref] [PubMed]
- Sharma AK, LaPar DJ, Zhao Y, et al. Natural killer T cell-derived IL-17 mediates lung ischemia-reperfusion injury. Am J Respir Crit Care Med 2011;183:1539-49. [Crossref] [PubMed]
- Lemaître PH, Vokaer B, Charbonnier LM, et al. IL-17A mediates early post-transplant lesions after heterotopic trachea allotransplantation in Mice. PLoS One 2013;8:e70236. [Crossref] [PubMed]
- Fan L, Benson HL, Vittal R, et al. Neutralizing IL-17 prevents obliterative bronchiolitis in murine orthotopic lung transplantation. Am J Transplant 2011;11:911-22. [Crossref] [PubMed]
- Okazaki M, Krupnick AS, Kornfeld CG, et al. A mouse model of orthotopic vascularized aerated lung transplantation. Am J Transplant 2007;7:1672-9. [Crossref] [PubMed]
- Stewart S, Fishbein MC, Snell GI, et al. Revision of the 1996 working formulation for the standardization of nomenclature in the diagnosis of lung rejection. J Heart Lung Transplant 2007;26:1229-42. [Crossref] [PubMed]
- Boehler A, Kesten S, Weder W, et al. Bronchiolitis obliterans after lung transplantation: a review. Chest 1998;114:1411-26. [Crossref] [PubMed]
- Verleden GM. Chronic allograft rejection (obliterative bronchiolitis). Semin Respir Crit Care Med 2001;22:551-8. [Crossref] [PubMed]
- Shilling RA, Wilkes DS. Role of Th17 cells and IL-17 in lung transplant rejection. Semin Immunopathol 2011;33:129-34. [Crossref] [PubMed]
- Vanaudenaerde BM, Dupont LJ, Wuyts WA, et al. The role of interleukin-17 during acute rejection after lung transplantation. Eur Respir J 2006;27:779-87. [Crossref] [PubMed]
- Snell GI, Levvey BJ, Zheng L, et al. Interleukin-17 and airway inflammation: a longitudinal airway biopsy study after lung transplantation. J Heart Lung Transplant 2007;26:669-74. [Crossref] [PubMed]
- Fossiez F, Djossou O, Chomarat P, et al. T cell interleukin-17 induces stromal cells to produce proinflammatory and hematopoietic cytokines. J Exp Med 1996;183:2593-603. [Crossref] [PubMed]
- Kurasawa K, Hirose K, Sano H, et al. Increased interleukin-17 production in patients with systemic sclerosis. Arthritis Rheum 2000;43:2455-63. [Crossref] [PubMed]
- Huang W, La Russa V, Alzoubi A, et al. Interleukin-17A: a T-cell-derived growth factor for murine and human mesenchymal stem cells. Stem Cells 2006;24:1512-8. [Crossref] [PubMed]
- Wilson MS, Madala SK, Ramalingam TR, et al. Bleomycin and IL-1beta-mediated pulmonary fibrosis is IL-17A dependent. J Exp Med 2010;207:535-52. [Crossref] [PubMed]
- Braun RK, Martin A, Shah S, et al. Inhibition of bleomycin-induced pulmonary fibrosis through pre-treatment with collagen type V. J Heart Lung Transplant 2010;29:873-80. [Crossref] [PubMed]
- Goers TA, Ramachandran S, Aloush A, et al. De novo production of K-alpha1 tubulin-specific antibodies: role in chronic lung allograft rejection. J Immunol 2008;180:4487-94. [Crossref] [PubMed]
- Hsu HC, Yang P, Wang J, et al. Interleukin 17-producing T helper cells and interleukin 17 orchestrate autoreactive germinal center development in autoimmune BXD2 mice. Nat Immunol 2008;9:166-75. [Crossref] [PubMed]
- Sato M, Keshavjee S. Bronchiolitis obliterans syndrome: alloimmune-dependent and -independent injury with aberrant tissue remodeling. Semin Thorac Cardiovasc Surg 2008;20:173-82. [Crossref] [PubMed]
- van den Berg WB, Miossec P. IL-17 as a future therapeutic target for rheumatoid arthritis. Nat Rev Rheumatol 2009;5:549-53. [Crossref] [PubMed]
- Gorbacheva V, Fan R, Li X, et al. Interleukin-17 promotes early allograft inflammation. Am J Pathol 2010;177:1265-73. [Crossref] [PubMed]
- Li J, Simeoni E, Fleury S, et al. Gene transfer of soluble interleukin-17 receptor prolongs cardiac allograft survival in a rat model. Eur J Cardiothorac Surg 2006;29:779-83. [Crossref] [PubMed]
- Lemaître PH, Vokaer B, Charbonnier LM, et al. Cyclosporine A drives a Th17- and Th2-mediated posttransplant obliterative airway disease. Am J Transplant 2013;13:611-20. [Crossref] [PubMed]
- Le Moine A, Goldman M, Abramowicz D. Multiple pathways to allograft rejection. Transplantation 2002;73:1373-81. [Crossref] [PubMed]
- Oboki K, Ohno T, Saito H, et al. Th17 and allergy. Allergol Int 2008;57:121-34. [Crossref] [PubMed]
- Mangan PR, Harrington LE, O'Quinn DB, et al. Transforming growth factor-beta induces development of the T(H)17 lineage. Nature 2006;441:231-4. [Crossref] [PubMed]
- Joffre O, Santolaria T, Calise D, et al. Prevention of acute and chronic allograft rejection with CD4+CD25+Foxp3+ regulatory T lymphocytes. Nat Med 2008;14:88-92. [Crossref] [PubMed]
- Itoh S, Kimura N, Axtell RC, et al. Interleukin-17 accelerates allograft rejection by suppressing regulatory T cell expansion. Circulation 2011;124:S187-96. [Crossref] [PubMed]
- Martin B, Hirota K, Cua DJ, et al. Interleukin-17-producing gammadelta T cells selectively expand in response to pathogen products and environmental signals. Immunity 2009;31:321-30. [Crossref] [PubMed]
- Simonian PL, Wehrmann F, Roark CL, et al. γδ T cells protect against lung fibrosis via IL-22. J Exp Med 2010;207:2239-53. [Crossref] [PubMed]
- Wynn TA. Integrating mechanisms of pulmonary fibrosis. J Exp Med 2011;208:1339-50. [Crossref] [PubMed]
- Gaffen SL. Recent advances in the IL-17 cytokine family. Curr Opin Immunol 2011;23:613-9. [Crossref] [PubMed]