Sealing effectiveness of a novel NHS-POx based patch: experiments in a dynamic ex vivo porcine lung
Highlight box
Key findings
• The novel gelatin patch impregnated with functionalized polyoxazolines exhibits good adhesive strength, better reduction of air leaks and elevated bursting pressures in a dynamic ex vivo porcine lung model.
What is known and what is new?
• Current lung sealants are insufficient for prevention of prolonged pulmonary air leakage, and better products are needed.
• The novel lung sealant patch is promising for further research and development, as compared with currently used products (TachoSil®, Hemopatch®, Progel®, Coseal®).
What is the implication, and what should change now?
• The novel patch shows promise as a lung sealant due to favourable mechanical properties. Further in vivo safety and efficacy studies should now be performed, in preparation for clinical use.
Introduction
Pulmonary air leakage (PAL) is an important concern for patients undergoing pulmonary resections. Prolonged PAL (pPAL), defined as PAL lasting longer than 5 days, affects 5.6–30% of patients (1). pPAL is the main reason for delayed chest tube removal, prolonged hospital stay and readmissions (2-4), while resulting in more complications including empyema (5), pneumonia (6,7) and mortality (8). Furthermore, 4.8% of patients with pPAL require additional interventions (extra chest tubes, bronchoscopy and reoperation) (6). In an attempt to prevent these postoperative consequences, various sealants have been developed for intraoperative use (9).
There is a broad variation in surgical management of air leaks. A recent study found significant between-hospital variation for pPAL incidence in the Netherlands (2.6–19.3% adjusted for case-mix), and also important variation regarding intraoperative PAL management, including sealant use (10). Available evidence supporting intraoperative sealant use in this setting is controversial at best, highlighted by a recent survey in which only 42% of surgeons believed enough evidence exists to support their sealant use (11). This is in line with a Cochrane review on this subject published in 2010 (12), that does not recommend the routine use of sealants due to unclear benefits, for example on length of hospital stay. However, this study pooled multiple products with varying modes of action into one analysis, but head-to-head comparisons between sealants are scarce (12,13). Considering some sealants might be more effective than others (14), a comparison of existing and novel products is warranted, to further substantiate sealant selection and use in current surgical practice. Furthermore, there is an unmet need for a single-component and more effective surgical sealant capable of sealing PAL in wet and dynamic biological environments, as stated by Brunelli (11).
To this end a novel patch based on porcine derived gelatin impregnated with synthetic polymers, may hold promise as a lung sealant. The gelatin-based patch contains poly (2-oxazolines) functionalized with N-hydroxysuccimide ester (NHS-POx) and nucleophilically activated polyoxazoline (NU-POx) (GATT Technologies BV, Nijmegen, The Netherlands) (15-18). After wet tissue contact, the reactive NHS side-chains will form covalent bonds with amines present on tissues, blood, NU-POx and the gelatin carrier itself. The polymers are further functionalized using hydroxyl groups for increased hydrophilicity to ensure adequate blood and water uptake, acting synergistically with coagulation mechanisms. This results in a strongly adhesive and cohesive polyoxazoline hydrogel with good sealing properties. Positive results have been obtained for hemostatic applications in vivo (15,17,18) and clinical trials are currently being performed to assess hemostatic efficacy on liver bleeding (ClinicalTrials.gov Identifier: NCT04819945 and NCT05385952).
In this study, we aim to investigate the aerostatic properties of this novel NHS-POx based patch in an ex vivo porcine lung model compared to other commonly used lung sealants, by measuring bursting pressure (BP), air leak (AL) reduction and conformability to the lung surface. While the ARRIVE reporting checklist is designed to improve the reporting of in vivo studies, we decide to follow the ARRIVE 2.0 reporting checklist in this ex vivo study considering many applicable items for better reporting in the study design, statistical analysis and results (available at https://jtd.amegroups.com/article/view/10.21037/jtd-22-1821/rc).
Methods
Study setup
We performed an ex vivo study in a dynamic porcine lung model. First, a multigroup comparison was undertaken evaluating the novel patch applied in two ways (GATT-Patch Single and GATT-Patch Double) compared to Progel® (C. R. Bard, Inc., Murray Hill, New Jersey, USA), Coseal® (Baxter International Inc., Deerfield, Illinois, USA), Hemopatch® (Baxter International Inc., Deerfield, Illinois, USA), and TachoSil® (Takeda Pharmaceutical Company Limited, Tokyo, Japan). Sample size (N=10 per group) was chosen based on previous reported experiments (14). GATT-Patch Single/Double and Hemopatch® groups were randomized (Microsoft Excel, Aselect function) and blinded to the allocated group until the sealant was handed to the researcher, to mitigate unconscious influences on lesion creation. All GATT-Patches were provided by the manufacturer to the researchers, and all control products were commercially ordered.
Secondly, following this multigroup comparison, we performed a randomized head-to-head comparison of the novel GATT-Patch Single compared to the best product with the same mode of application (Hemopatch®). This experiment was undertaken in a similar randomized and blinded fashion after a formal sample size calculation (power =0.8, two-sided alpha =0.05, N=10 per group).
Porcine lung model
No live animals were involved in experimental procedures, and all experiments were performed on ex vivo porcine lung tissue obtained from a slaughterhouse. Lungs from domestic pigs, used for human consumption and weighing between 90–100 kg, were excised in the slaughterhouse, and put on ice as soon as possible for transport. All excess tissue was removed and sutures were used to prevent blood leaking in the setup. Caudal lobes were suctioned and selectively intubated (6.0 mm endo-tracheal tube). Lungs were inflated manually, followed by clamping of the tube. Airtightness was ensured before use. Lobes with poor compliance or persistent atelectasis (as visually assessed) were rejected. Rewarming of the included porcine lungs was started approximately fifteen minutes before measurements.
Specimens were examined in a clear container containing 10 L 0.9% saline (NaCl) kept at 37 ℃. A camera positioned under the container and a ruler positioned at water level were used to record PAL and surface area expansion (SAE) (Figure 1 and Video 1). Airway pressures were recorded from the mechanical ventilator (Appendix 1), and synchronized to the video data by pressing on the lung (visible on both datafiles). Tidal volumes were noted from the mechanical ventilator at the end of each measurement cycle to calculate AL.
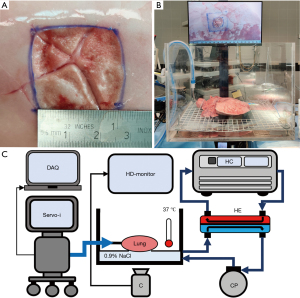
Study protocol and outcome measures
Study outcomes were collected during three separate measurement cycles: (I) before and (II) after creating a standardized superficial pleural lesion, and (III) after sealant application. All study outcomes and definitions are presented in Table 1. To ensure standardized study measurements, porcine lung specimen were ventilated under increasing pressures with pressure control ventilation [initial settings: respiratory rate 12/min, inspiratory:expiratory ratio 1:2, positive end-expiratory pressure (PEEP) 5 cmH2O and pressure above PEEP 5 cmH2O]. Each measurement cycle, the plateau ventilatory pressure (Pplat) was re-started at the initial settings and increased with 5 cmH2O every 90 seconds. Pressure was increased until a maximum of 40 cmH2O for cycles (I) and (II) and 70 cmH2O or upon reaching of grade III leakage [Table 1, Macchiarini scale (19)] during cycle (3). These measurement cycles were conducted as follows: (I) baseline SAE measurements were performed after marking a 5 cm × 5 cm area at static lung inflation of 5 cmH2O PEEP on a dorsal non-atelectatic segment of the caudal lobe and measuring ‘Baseline SAE’, ‘Lung compliance’ and ‘Baseline AL’. The SAE is defined as the percentual increase of this previously marked area at 40 cmH2O with respect to 5 cmH2O as a measure for lung surface expandability (see Figure 1B); (II) Defect AL measurements were performed after creating a standardized superficial pleural lesion (2.5 cm × 2.5 cm, Figure 1A) using a sanding wheel (Dremel Lite 7760) and tweezers/scissors at static lung inflation of 10 cmH2O PEEP. The lesion was made at the minimum depth required to remove the superficial pleura and expose the underlying parenchyma. Measurements included ‘Baseline leaking pressures’ [using the Macchiarini scale (19)] and ‘Defect AL’; (III) BP measurements were performed after sealant application, applying all patches at 5 cmH2O PEEP static lung inflation and gels on a deflated lung (Table 2). In case of atelectasis following sealant application, 15–25 cmH2O PEEP was administered for 5–10 s. Measurements included ‘BP’, ‘AL-reduction’, ‘Mode of failure’ and ‘expansion area change (EAC)’. BP was defined as the pressure setting at which the first bubbles were visible (i.e., grade I AL), and for values higher than 70 cmH2O, 75 cmH2O was noted as an off-scale value. EAC is the measure of sealant conformability to the lung, defined as the proportion SAE post sealant application with respect to SAE pre sealant application (Table 1).
Table 1
Variable | Definition |
---|---|
Measurement cycle 1: baseline SAE | |
Baseline SAE | Surface area at 40 cmH2O/surface area at 5 cmH2O (ImageJ, version 1.53a, Wayne Rasband) |
Baseline AL | Calculated at Pplat =40 cmH2O, as “12x(TVi-‘expiratory tidal volume’) (read from the mechanical ventilator user interface) |
AL measured during ‘Baseline SAE’ measurements was subtracted from ‘Defect AL’ and ‘BP measurements’ to correct for circuit leakage and bias flow | |
Lung compliance | TVi at 40 cmH2O/35 |
Ischemic time | Time from arrival at laboratory until start of experiments |
Measurement cycle 2: defect AL | |
Baseline leaking pressures | Macchiarini scale (grades: I = countable bubbles, II = stream of bubbles, III = coalesced bubbles) (16), assessed by two investigators |
Defect AL | See ‘Baseline AL’ |
Surface and water temperature | Measured before sealant application |
Measurement cycle 3: BP measurements | |
Sealed leaking pressures | See ‘Baseline leaking pressures’ |
BP = pressure at which first AL occurred (grade I) | |
AL-reduction | BP measurements AL: see ‘Baseline AL’ |
100%x [(Defect AL–BP measurements AL)/Defect AL], capped at 0 and 100% (calculated at Pplat =40 cmH2O). Always noted as 100% if BP >40 cmH2O | |
EAC | Sealed SAE: see ‘Baseline SAE’ |
EAC = Sealed SAE/Baseline SAE | |
EAC =100% indicates perfect conformability of the sealant to lung expansion, EAC <100% indicates reduced SAE after sealant application compared to baseline (Appendix 1) | |
Mode of failure | Adhesive failure (leakage between sealant and lung; Figure 3D) |
Cohesive failure (leakage through the sealant; Figure 3F) |
SAE, surface area expansion; AL, air leak; Pplat, plateau ventilatory pressure; TVi, inspiratory tidal volume; BP, bursting pressure; EAC, expansion area change.
Table 2
Sealant | Application |
---|---|
TachoSil® (4.8x4.8 cm) | Saline gauzes: 3-minute compression |
Hemopatch® (4.5x4.5 cm) | SBB lung priming |
Dry gauzes: 2-minute compression | |
GATT-patch single (5x5 cm) | SBB lung priming |
Diluted SBB gauzes: 2-minute compression + 60 mL diluted SBB irrigation | |
GATT-patch double (5x5 cm) | SBB lung priming, apply first patch |
Apply second patch perpendicularly with 60 mL SBB irrigation between patches | |
Saline gauzes: 2-minute compression + 60 mL saline irrigation | |
Coseal® (2 mL) | Spray marked area, dry 60 seconds |
Progel® (2 mL) | Spray marked area, dry 120 seconds |
SBB, sodium-bicarbonate buffer (pH 8.3–8.5); diluted SBB, SBB with saline (1:2).
Recorded video data was used for independent assessment of leaking pressures and BP by two investigators, noting the timepoints when different leaking grades occurred in the videos (Table 1), followed by reaching consensus and synchronization of the corresponding pressure values. Assessors were blinded to the assigned group for the baseline videos, but not for the sealed samples due to obvious differences in product appearance.
Statistical analysis
Statistical analysis was performed using IBM SPSS Statistics for Windows, Version 26.0 (Armonk, New York, USA; IBM Corp). Kolmogorov-Smirnov test was used for assessment of data distribution. Non-normally distributed data and normally distributed data were presented as median ± interquartile range (IQR) or mean ± standard deviation (SD) respectively. For the first experiment, Kruskal-Wallis test (non-parametric) or analysis of variance (parametric) were used. Bonferroni correction was used during post-hoc analysis (pairwise). For the second experiment, Mann-Whitney-U test (non-parametric) or independent samples t-test (parametric) were used. Fischer’s exact test was used for categorical data. Missing data was analyzed by pairwise exclusion. Tests were performed two-tailed and the null-hypothesis was rejected if P<0.05.
Results
First experiment
We performed 60 measurements on 60 lungs, 10 for each group of sealants. Baseline characteristics were similar between groups (Table 3). Four measurements had missing or incomplete video/pressure data, due to technical errors (GATT-Patch Double and Single, TachoSil® and Coseal®). One measurement had missing tidal volumes for baseline AL measurements (GATT-Patch Double).
Table 3
Characteristics | Patch sealants | Spray sealants | P value | |||||
---|---|---|---|---|---|---|---|---|
GATT-Patch double | GATT-Patch single | Hemopatch® | TachoSil® | Progel® | Coseal® | |||
Baseline characteristics | ||||||||
Sample characteristics | ||||||||
Ischemic time (minutes)† | 238±141 | 233±105 | 119±66 | 215±158 | 213±102 | 226±164 | 0.32 | |
Left lower lobe, n [%] | 8 [80] | 9 [90] | 5 [50] | 7 [70] | 6 [60] | 9 [90] | 0.33 | |
Physical characteristics | ||||||||
Surface temperature (℃)† | 31.2±1.2 | 31.8±1.6 | 31.9±1.3 | 31.7±2.2 | 33.0±1.0 | 31.7±1.2 | 0.15 | |
Water temperature (℃)‡ | 37.2±0.5 | 37.2±0.3 | 37.3±0.3 | 37.2±0.5 | 37.2±0.5 | 37.1±0.2 | 0.49 | |
Baseline SAE (%)‡ | 152±13 | 149±25 | 153±18 | 148±20 | 153±35 | 151±15 | >0.99 | |
Lung compliance (mL/cmH2O)‡ | 24±7 | 23±7 | 27±2 | 26±7 | 24±6 | 26±5.0 | 0.30 | |
Leakage characteristics | ||||||||
Baseline leaking pressures | ||||||||
Grade I (cmH2O)‡ | 10±5 | 13±11 | 10±5 | 15±5 | 10±1 | 13±5 | 0.15 | |
Grade II (cmH2O)‡ | 15±3 | 18±15 | 15±1 | 20±10 | 18±5 | 15±5 | 0.44 | |
Grade III (cmH2O)† | 23±6 | 26±8 | 23±5 | 24±8 | 23±6 | 22±5 | 0.84 | |
AL | ||||||||
Amount (L/min)‡ | 1.1±0.6 | 0.6±1.5 | 1.2±0.8 | 0.7±1.4 | 1.1±1.4 | 0.9±0.9 | 0.64 | |
Percent of TVi (%)‡ | 10.7±6.2 | 5.9±11.2 | 9.6±7.9 | 6.7±9.6 | 10.2±12.2 | 7.9±6.3 | 0.62 | |
Experiment outcomes | ||||||||
Sealed leaking pressures | ||||||||
Grade I (cmH2O)‡ | 60±24 | 40±10 | 33±6 | 30±11 | 33±11 | 25±13 | <0.001* | |
Grade II (cmH2O)‡ | 60±24 | 45±15 | 33±6 | 30±11 | 33±15 | 30±13 | <0.001* | |
Grade III (cmH2O)‡ | 65±21 | 50±29 | 35±6 | 30±20 | 40±19 | 40±25 | <0.001* | |
AL-reduction (%)‡ | 100±1 | 100±14 | 46±50 | 31±29 | 89±40 | 90±58 | <0.001* | |
Cohesive failure, n [%] | 8 [89] | 9 [90] | 3 [30] | 2 [20] | 8 [89] | 10 [100] | <0.001* | |
EAC (%)† | 76±9 | 77±9 | 92±13 | 80±11 | 88±14 | 98±13 | 0.001* |
*, P value indicate statistical significance (P<0.05). †, mean ± standard deviation (SD); ‡, median ± interquartile range (IQR). SAE, surface area expansion; AL, air leak; TVi, inspiratory tidal volume; EAC, expansion area change.
GATT-Patch Double (60±24 cmH2O) showed significantly higher BP compared to Progel® (33±11 cmH2O, P=0.005), Coseal® (25±13 cmH2O, P=0.001), Hemopatch® (33±6 cmH2O, P=0.006) and TachoSil® (30±11 cmH2O, P<0.001), but no significant differences in BP compared to GATT-Patch Single (40±10 cmH2O) (P>0.99). Between GATT-Patch Single, Progel®, Coseal®, Hemopatch® and TachoSil® no significant differences in BP were found (Figure 2A, Table 3). AL-reduction was significantly better for GATT-Patch Double compared to Hemopatch® (P=0.010) and TachoSil® (P<0.001). GATT-Patch Single (P=0.004) and Progel® (P=0.027) showed significantly better AL-reduction compared to TachoSil® (Figure 2B, Table 3).
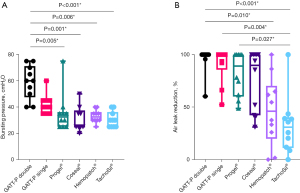
The EAC was significantly better for Coseal® (98%±13%) compared to TachoSil® (80%±11%, P=0.023), GATT-Patch Single (77%±9%, P=0.005) and GATT-Patch Double (76%±9%, P=0.004) (Table 3). Cohesive failure was observed more often for GATT-Patch Double, GATT-Patch Single, Progel® and Coseal®, while adhesive failure was seen more frequently for Hemopatch® and TachoSil® (P<0.001) (Table 3, Figure 3, Video 1). Interestingly, different failure mechanisms were observed: progression until grade III leakage was generally more gradual for GATT-Patch Double, GATT-Patch Single, Progel® and Coseal®, requiring one or more pressure intervals to reach grade III leakage. For Hemopatch® and TachoSil®, grade III leakage was usually reached in the same pressure interval where first leakage occurred (Table 3).
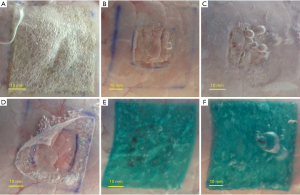
Second experiment
Twenty measurements were performed on 20 lungs, 10 for each group. Despite adequate randomization, baseline AL was found to be significantly higher in the GATT-Patch Single group compared to the Hemopatch group (1.5±0.8 vs. 0.8±0.5 L/min, P=0.03). Other baseline characteristics were not significantly different (Table S1). Despite the larger baseline AL, BP for GATT-Patch Single (45±10 cmH2O) was significantly higher compared to Hemopatch® (40±6 cmH2O, P=0.043) (Figure 4A). AL-reduction also reached statistical significance (P=0.043) (Figure 4B). Hemopatch® showed only adhesive failure and GATT-Patch Single only cohesive failure (P<0.001). EAC measurements were not performed due to extensive adhesive failure of Hemopatch®.
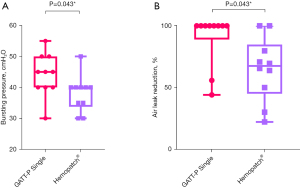
Discussion
This ex vivo experimental study demonstrated favorable mechanical properties for the novel NHS-POx based lung sealant (GATT-Patch). The patch exhibited excellent adhesive characteristics to the lung parenchyma, withstanding repetitive lung expansions in a wet and dynamic ex vivo lung environment without tearing or debonding until pressures of at least 30–40 cmH2O. Importantly, in the second head-to-head comparative experiment, GATT-Patch Single showed improved performance compared with a commercially available patch used for lung sealing with a similar application method (Hemopatch®).
Interpretation of results
The potentially higher BP which can be achieved with the NHS-POx patch may be of clinical relevance. It has been suggested that the BP of a sealant should be high enough to withstand pressures during coughing (14) or during positive-pressure recruitment maneuvers (up to 40 cmH2O) (20,21). Physiologically, intra-thoracic pressures during coughing can reach up to 408 cmH2O (22) and up to 71 cmH2O in the post-thoracotomy state (23). Pedersen suggested that the actual pressures on the lesion might be lower due to pleural apposition, relieving transpulmonary pressures during coughing (14). In this context, we hypothesize that different modes of failure could make some sealants more effective than others. We observed that TachoSil® and Hemopatch® generally lose their sealing capacities after bursting due to lower adhesive strength. Progel®, Coseal®, GATT-Patch Double and Single remain effective in reducing the AL even after reaching BP and take one or more pressure-intervals to reach higher leakage intensities, due to the strong adhesive properties to the entire parenchymal lung defect (Table 3) (24).
As described previously, a lung sealant should have high conformability to the lung surface, to prevent debonding when re-expanding the lung (24). We hypothesize this is especially true for gel-based sealants, as they cannot be applied to a slightly inflated lung with active leakage (air bubble formation). We observed tears leading to sealant failure for Coseal® and Progel® under increasing lung expansions, which may render these sprays less suitable for coverage of large areas. Patch sealants might not require as much conformability compared to gel sealants, being applicable to a slightly inflated lung surface with an active leak (counterpressure with gauzes). We observed successful applications at PEEP =5 cmH2O for the TachoSil®, Hemopatch® and GATT-Patch groups. Debonding was not observed in the GATT-Patch groups during lung expansions due to strong adhesive properties. Thus, the experimentally measured lower EAC compared to Coseal® might not be of clinical importance.
Ease of use is an important benchmark for a sealants usability in the operating room. GATT-Patch Single has a similar mode of application compared to Hemopatch® and TachoSil®. However, GATT-Patch Double might be more difficult to implement in a clinical setting, especially minimally invasive surgery. In this case, a sprayable sealant might be preferable, which may also be better suited on irregular surfaces such as stapler lines, due to mechanical interlocking. Consequently, different modes of application are required depending on the surgical scenario.
Before clinical use of GATT-Patch as a lung sealant, safety outcomes for implantation in the thoracic cavity need to be assessed in-vivo, including inflammatory properties, influences on wound healing, adhesion formation with the parietal pleura and biodegradation. Roozen et al. has shown that the histological results of GATT-Patch for intra-abdominal implantation were similar to control patches, showing complete biodegradation within 4–6 weeks (17). However, no significant conclusions could be drawn with respect to adhesion formation (17). Chemically, the amide and thioester bonds formed due to NHS reactivity are hydrolysable, and the NHS-POx degradation products have been shown to be effectively renally excreted in a rat model (16). Porcine-derived gelatin, constituting the patch-carrier for NHS-POx, is already being used in other devices such as SURGIFLO® Hemostatic Matrix (Ethicon Inc. Raritan, New Jersey, USA), Gelfoam® and Gelita TUFT-IT® and may be cleared in 3 weeks (17). A theoretical downside to this material is the potential for antigenicity and allergic reactions, but this is very rare (25). Finally, GATT-Patch is being investigated in multi-center clinical trials of liver surgery (ClinicalTrials.gov Identifier: NCT04819945 and NCT05385952), suggestive of a promising safety profile in humans.
Comparison to literature
The extent of intra-operative PAL has previously been used to predict the occurrence of pPAL. Kim found an AL percentage of inspiratory tidal volume (TVi) >9.5% is predictive of pPAL, while Brunelli showed this for AL >500 mL/min (26,27). Furthermore, a recent consensus survey has proposed that AL >400 mL/min is classified as severe (28). Therefore, the amount of AL observed in our study as a result of the created pleural lesions in porcine lungs (median 0.6–1.2 L/min at Pplat =40 cmH2O, Table 3) seems clinically relevant.
In previously published ex vivo experiments, defect AL wasn’t always determined, making interpretation of those results more troublesome. Pedersen and Zang/Bures found higher BP for TachoSil® (median =35 cmH2O, range =30–55 cmH2O and mean =36.0 cmH2O, SD =4.9 cmH2O, respectively) when compared to our finding (median 30, range, 25–40 cmH2O) (14,29). This may be due to differences in defect used. Fibrin glues, commonly used in thoracic surgery (9), were also investigated by Pedersen. However, Tisseel® and Evicel® both performed significantly worse when compared to TachoSil®, and were therefore not included in our study (14).
In current literature, comparative clinical studies evaluating effectiveness between sealants are scarce. In a recent systematic review (13), only 2/21 included studies compared one sealant to another. In these two randomized clinical trials (RCTs) [BioGlue vs. Vivostat (30) and Tisseel vs. Vivostat (31)], no significant differences were found in postoperative PAL duration. A more recent RCT not included in this systematic review compared TachoSil and Neoveil, showing no significant differences (32). Since 2018, a new clinical trial has been started (ClinicalTrials.gov Identifier: NCT03450265), aiming to compare Hemopatch® to TachoSil® in a non-inferiority study. Based on a favourable trend in AL-reduction seen in our study (Figure 2), the capabilities of Hemopatch® for intraoperative AL management and preventing pPAL may be superior when compared to TachoSil® clinically, but results of this trial have to be awaited.
Limitations
For AL quantification, the tidal volumes displayed by the ventilator were used, and corrected for baseline deviations which could be caused by system leaks or bias flow. The last breath of each pressure setting was used, to ensure the measurement was not influenced by resolving atelectasis (overestimation) or escaping trapped air (underestimation). However, despite the use of similar AL measurements in previous studies (26,27,29), the most optimal air leak quantification approach remains to be validated.
With our SAE measurements, we attempted to integrate a conformability measurement in the BP setup. Due to possible influences by tears forming in the sealant (Progel® and Coseal®), debonding of the sealant (Hemopatch®) and camera angle, results should be interpreted cautiously (Appendix 1). The method used by Yamaoka may have advantages in this perspective, not being influenced by different tearing/debonding characteristics (24).
Difficulties were observed while applying Coseal® with the standard applicator, leading to inhomogeneous coverage, and higher BP may be reached with a more homogenous layer (e.g., dedicated spray set). Due to cohesive failure in Progel® and Coseal® groups, a thicker layer may lead to higher BP. Due to adhesive failure in TachoSil® and Hemopatch® groups, higher BP may be obtained with a larger adhesive overlap around the defect.
In the first experiment, randomization was not performed in the Progel® and Coseal® groups due to the limited shelf life of the prepared syringes (4 mL) and not in the TachoSil® group using the same allocation scheme. Influence of possible unconscious biases are expected to be low, due to the demonstrated statistically similar baseline measurements in the first experiment. Sample sizes were not large enough to detect all significant differences. Due to a significantly larger air leak in the GATT-Patch Single group in the second experiment, the actual effect size between GATT-Patch Single and Hemopatch may have been underestimated.
Recommendations for future research
While the porcine lung has been found to be comparable to the human lung (33), ex vivo results should be interpreted cautiously, as they may not translate accordingly to in-vivo situations (e.g., due to pleural mechanisms, coagulation, immune response, physiological breathing). The NHS-POx patch mode of application should be further optimized to improve usability in a clinical setting while maximizing mechanical cohesive strength (e.g., by creating a thicker single-layer patch). Before clinical use can be established, safety outcomes for implantation of the GATT-Patch in the thoracic cavity need to be assessed in-vivo. Fundamentally, mechanisms of sealant failure and BP should be further studied in relation to critical moments such as coughing or recruitment maneuvers, to establish threshold values for product development.
Conclusions
In conclusion, the novel NHS-POx based patch shows promise as a lung sealant due to favorable mechanical properties, demonstrating elevated BP and good adhesive strength to the lung. The gradual leakage pattern may provide superior reduction of an AL, especially in challenging clinical scenario’s such as coughing or mechanical recruitment. The mode of sealant application should be optimized, and further in-vivo research is required to validate our findings and establish a safety profile for intra-thoracic implantation.
Acknowledgments
The authors would like to thank Nicole Calon, Roger Lomme and Pim van Sambeeck for hands-on support during experiments, Jeroen van Doorn and Jonne Doorduin for their help with the mechanical ventilator setup, Timo Bogaarts and Jasper Toebes for their help with conceptualizing air leak measurements and the Radboudumc department of statistics with help during the statistical analysis. Part of this work has previously been presented at the STS 58th Annual Meeting.
Funding: This work was funded by GATT-Technologies B.V. (Nijmegen, The Netherlands).
Footnote
Reporting Checklist: The authors have completed the ARRIVE reporting checklist. Available at https://jtd.amegroups.com/article/view/10.21037/jtd-22-1821/rc
Data Sharing Statement: Available at https://jtd.amegroups.com/article/view/10.21037/jtd-22-1821/dss
Conflicts of Interest: All authors have completed the ICMJE uniform disclosure form (available at https://jtd.amegroups.com/article/view/10.21037/jtd-22-1821/coif). ER is employee of GATT Technologies and HG was a Scientific Advisor for GATT Technologies B.V until 31 December 2021, but not in relation to lung sealing technology. BH received funding and study materials through the institution for conduction of the study from GATT-Technologies B.V. The authors have no conflicts of interest to declare.
Ethical Statement: The authors are accountable for all aspects of the work in ensuring that questions related to the accuracy or integrity of any part of the work are appropriately investigated and resolved. No live animals were involved in experimental procedures, and all experiments were performed on
Open Access Statement: This is an Open Access article distributed in accordance with the Creative Commons Attribution-NonCommercial-NoDerivs 4.0 International License (CC BY-NC-ND 4.0), which permits the non-commercial replication and distribution of the article with the strict proviso that no changes or edits are made and the original work is properly cited (including links to both the formal publication through the relevant DOI and the license). See: https://creativecommons.org/licenses/by-nc-nd/4.0/.
References
- Attaar A, Tam V, Nason KS. Risk Factors for Prolonged Air Leak After Pulmonary Resection: A Systematic Review and Meta-analysis. Ann Surg 2020;271:834-44. [Crossref] [PubMed]
- Seely AJ, Ivanovic J, Threader J, et al. Systematic classification of morbidity and mortality after thoracic surgery. Ann Thorac Surg 2010;90:936-42; discussion 942. [Crossref] [PubMed]
- Brunelli A, Chapman K, Pompili C, et al. Ninety-day hospital costs associated with prolonged air leak following lung resection. Interact Cardiovasc Thorac Surg 2020;31:507-12. [Crossref] [PubMed]
- Liu J, Yang X, Liu X, et al. Predictors of Readmission After Pulmonary Resection in Patients With Lung Cancer: A Systematic Review and Meta-analysis. Technol Cancer Res Treat 2022;21:15330338221144512. [Crossref] [PubMed]
- Brunelli A, Xiume F, Al Refai M, et al. Air leaks after lobectomy increase the risk of empyema but not of cardiopulmonary complications: a case-matched analysis. Chest 2006;130:1150-6. [Crossref] [PubMed]
- Liang S, Ivanovic J, Gilbert S, et al. Quantifying the incidence and impact of postoperative prolonged alveolar air leak after pulmonary resection. J Thorac Cardiovasc Surg 2013;145:948-54. [Crossref] [PubMed]
- Singhal S, Ferraris VA, Bridges CR, et al. Management of alveolar air leaks after pulmonary resection. Ann Thorac Surg 2010;89:1327-35. [Crossref] [PubMed]
- Yoo A, Ghosh SK, Danker W, et al. Burden of air leak complications in thoracic surgery estimated using a national hospital billing database. Clinicoecon Outcomes Res 2017;9:373-83. [Crossref] [PubMed]
- Rocco G, Rendina EA, Venuta F, et al. The use of sealants in modern thoracic surgery: a survey. Interact Cardiovasc Thorac Surg 2009;9:1-3. [Crossref] [PubMed]
- Hoeijmakers F, Hartemink KJ, Verhagen AF, et al. Variation in incidence, prevention and treatment of persistent air leak after lung cancer surgery. Eur J Cardiothorac Surg 2021;61:110-7. [Crossref] [PubMed]
- Brunelli A, Bölükbas S, Falcoz PE, et al. Exploring consensus for the optimal sealant use to prevent air leak following lung surgery: a modified Delphi survey from The European Society of Thoracic Surgeons. Eur J Cardiothorac Surg 2021;59:1265-71. [Crossref] [PubMed]
- Belda-Sanchís J, Serra-Mitjans M, Iglesias Sentis M, et al. Surgical sealant for preventing air leaks after pulmonary resections in patients with lung cancer. Cochrane Database Syst Rev 2010;2010:CD003051. [Crossref] [PubMed]
- McGuire AL, Yee J. Clinical outcomes of polymeric sealant use in pulmonary resection: a systematic review and meta-analysis of randomized controlled trials. J Thorac Dis 2018;10:S3728-39. [Crossref] [PubMed]
- Pedersen TB, Honge JL, Pilegaard HK, et al. Comparative study of lung sealants in a porcine ex vivo model. Ann Thorac Surg 2012;94:234-40. [Crossref] [PubMed]
- Boerman MA, Roozen E, Sánchez-Fernández MJ, et al. Next Generation Hemostatic Materials Based on NHS-Ester Functionalized Poly(2-oxazoline)s. Biomacromolecules 2017;18:2529-38. [Crossref] [PubMed]
- Boerman MA, Roozen EA, Franssen GM, et al. Degradation and excretion of poly(2-oxazoline) based hemostatic materials. Materialia 2020;12:100763. [Crossref]
- Roozen E, Lomme R, Calon N, et al. Efficacy of a novel polyoxazoline based hemostatic patch in liver and spleen surgery. 2023.
- Roozen EA, Warlé MC, Lomme RMLM, et al. New polyoxazoline loaded patches for hemostasis in experimental liver resection. J Biomed Mater Res B Appl Biomater 2022;110:597-605. [Crossref] [PubMed]
- Macchiarini P, Wain J, Almy S, et al. Experimental and clinical evaluation of a new synthetic, absorbable sealant to reduce air leaks in thoracic operations. J Thorac Cardiovasc Surg 1999;117:751-8. [Crossref] [PubMed]
- Marini JJ. Recruitment by sustained inflation: time for a change. Intensive Care Med 2011;37:1572-4. [Crossref] [PubMed]
- Annabi N, Zhang YN, Assmann A, et al. Engineering a highly elastic human protein-based sealant for surgical applications. Sci Transl Med 2017;9:eaai7466. [Crossref] [PubMed]
- McCool FD. Global physiology and pathophysiology of cough: ACCP evidence-based clinical practice guidelines. Chest 2006;129:48S-53S. [Crossref] [PubMed]
- Byrd RB, Burns JR. Cough dynamics in the post-thoracotomy state. Chest 1975;67:654-7. [Crossref] [PubMed]
- Yamaoka M, Maki N, Wijesinghe A, et al. Novel Alaska Pollock Gelatin Sealant Shows High Adhesive Quality and Conformability. Ann Thorac Surg 2019;107:1656-62. [Crossref] [PubMed]
- White RZ, Kerr L, White TJ, et al. Review of topical gelatin-based haemostatic agents; an insidious culprit of intraoperative anaphylaxis? ANZ J Surg 2021;91:2002-7. [Crossref] [PubMed]
- Kim WH, Lee HC, Ryu HG, et al. Intraoperative ventilatory leak predicts prolonged air leak after lung resection: A retrospective observational study. PLoS One 2017;12:e0187598. [Crossref] [PubMed]
- Brunelli A, Salati M, Pompili C, et al. Intraoperative air leak measured after lobectomy is associated with postoperative duration of air leak. Eur J Cardiothorac Surg 2017;52:963-8. [Crossref] [PubMed]
- Zaraca F, Brunelli A, Pipitone MD, et al. A Delphi Consensus report from the "Prolonged Air Leak: A Survey" study group on prevention and management of postoperative air leaks after minimally invasive anatomical resections. Eur J Cardiothorac Surg 2022;62:ezac211. [Crossref] [PubMed]
- Zhang R, Bures M, Höffler K, et al. In vitro comparison of two widely used surgical sealants for treating alveolar air leak. Thorac Cardiovasc Surg 2014;62:705-9. [Crossref] [PubMed]
- Belcher E, Dusmet M, Jordan S, et al. A prospective, randomized trial comparing BioGlue and Vivostat for the control of alveolar air leak. J Thorac Cardiovasc Surg 2010;140:32-8. [Crossref] [PubMed]
- Kılıç B, Erşen E, Demirkaya A, et al. A prospective randomized trial comparing homologous and autologous fibrin sealants for the control of alveolar air leak. J Thorac Dis 2017;9:2915-22. [Crossref] [PubMed]
- Bachmann H, Dackam SVC, Hojski A, et al. Neoveil versus TachoSil in the treatment of pulmonary air leak following open lung surgery: a prospective randomized trial. Eur J Cardiothorac Surg 2022;63:ezad003. [Crossref] [PubMed]
- Rogers CS, Abraham WM, Brogden KA, et al. The porcine lung as a potential model for cystic fibrosis. Am J Physiol Lung Cell Mol Physiol 2008;295:L240-63. [Crossref] [PubMed]