Continuous intraoperative neuromonitoring of the recurrent laryngeal nerve during lung operations
Highlight box
Key findings
• Continuous neuromonitoring of the recurrent laryngeal nerve (RLN) is safe and reproducible during lung surgery.
What is known and what is new?
• Especially on the left side, the laryngeal nerve is vulnerable during lung operations.
• Despite the use of a double lumen tube, we are able to monitor the laryngeal nerve continuously.
What is the implication, and what should change now?
• With the use of continuous intraoperative neuromonitoring, the postoperative rate of nerve palsy should decrease.
Introduction
Owing to its topographical location below the aortic arch, the recurrent laryngeal nerve (RLN) is at risk during left-sided thoracic procedures. Different configurations and courses of the left RLN have been described (1-4). Unilateral injury to the RLN results in dysfunction of the cricoarytenoid muscle resulting in vocal cord paralysis. Typical clinical symptoms may include hoarseness, a disturbed cough reflex, dyspnea, and swallowing difficulties with microaspiration (5,6). RLN injuries that occur within the scope of left thoracic procedures have been associated with a rate of postoperative pneumonia, re-intubation, aspiration of secretion, and an altogether increased 90-day mortality (7,8). Chiu et al. (9) reported a left RLN paralysis rate of 26.6% following esophageal resection with radical lymphadenectomy and Taenaka et al. a rate of 4.7% after 688 cardiac surgeries (10). Within the scope of thoracic aortic surgery, Ohta et al. observed an RLN paralysis rate of 5%, increasing up to 26% following aortic arch replacement or surgery of the descending aorta (11,12). Following thoracic surgery, the rates of postoperative RLN paralysis ranged between 3.7% and 15% the retrospective series (9,13). The left side was affected up to eight times more frequently than the right side (13).
The use of continuous intraoperative neuromonitoring (cIONM), as is common in thyroid surgery, might be beneficial (14,15). It involves exposing the nerve to a supra-threshold continuous stimulus which, if the nerve function is intact, triggers an action potential by conducting the stimulus to the muscle. This action potential is picked up by an electrode and displayed visually and audibly on a monitor. To record the action potential of the muscle, an electrode is placed at the level of the vocal cords. The success of cIONM, therefore depends essentially on the correct position of this adhesive electrode. The clear advantage of cIONM is its ability to intraoperatively monitor the function of the nerve during dissection and to become aware of potential nerve damage by traction (15,16).
Nearly all thoracic surgical procedures require the use of one-lung ventilation. To enable this, in most cases, patients are intubated with a double-lumen tube (DLT) under bronchoscopic control. For the routine use of cIONM during left thoracic surgical procedures some key points have to be clearly established. It needs to be clarified, how to best stimulate the nerve intrathoracally, also where precisely the adhesive electrode should be positioned on the DLT.
The aim of this study was to evaluate the feasibility and safety of cIONM during left sided thoracic operations. We present this article in accordance with the TREND reporting checklist (available at https://jtd.amegroups.com/article/view/10.21037/jtd-22-1515/rc).
Methods
We recruited patients who were scheduled to undergo a left-sided thoracotomy at University Hospital Marburg. The study was conducted in accordance with the Declaration of Helsinki (as revised in 2013). The responsible ethics committee of the Faculty of Human Medicine of Phillips University approved the study (ref. 134/18). All patients were briefed about the study and signed the informed consent. A phoniatric study was carried out in all participants before and after the surgery to determine whether the vocal cords were functioning properly. Patients who refused the postoperative phoniatric examination were excluded from the following analysis. Another criterion for exclusion was preoperatively existing RLN paralysis. All patients were intubated with a DLT.
Position of the recording electrodes on the DLT
We used DLTs from Medicoplast International (Illingen, Germany) made from latex-free polyvinyl chloride (PVC), which are routinely used at our clinic. Right- and left-sided variants of these tubes are available. The sizes of the DLTs used ranged between 28 and 41 Charrière (Ch). The tube size to be used was based on the patient’s respective height (Table 1). In the majority of patients, a left-sided tube was used.
Table 1
Height (cm) | Tube size (Ch) |
---|---|
150–160 | 35 |
161–170 | 37 |
171–180 | 39 |
181–190 | 41 |
The recording electrodes from Dr. Langer Medical (Waldkirch, Germany) are adhesive. They feature two long contact surfaces, which are separated by a printed centerline and are made from silver chloride-coated soft silicone. The electrodes are protected by a numbered application paper, which protrudes approx. Ten mm beyond the end of the electrodes. At the lower end of the electrodes, there is a separating strip from which the electrode cables exit. The electrode cables are 750 mm long and are insulated red and blue for the respective connection.
Prior to intubation, the DLT was cleaned and dried in order to ensure optimal adhesion of the electrodes. First, the distal electrode [called electrode 1 (E1)] was affixed. The proximal end of the tracheal cuff has a black marking which indicates the starting point of the adhesive electrode. It is attached in a way that the recording surfaces are placed laterally to the presumed median line of the body after intubation.
The second electrode was affixed proximal to the first electrode [electrode 2 (E2)] such that it formed precisely one line with the first one. In doing so, the recording cables of the distal electrode are not placed below the second electrode but are kept outside the adhesive film. The recording cable of the distal electrode was marked green and the cable of the proximal electrode was marked red. Figure 1 demonstrates a DLT with the affixed electrodes prior to intubation.
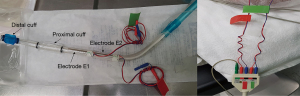
We used the AVALANCHE® neuromonitor from Dr. Langer Medical (Waldkirch, Germany). This equipment can be run with a program designed specifically for simultaneous recording via two electrodes, both for intermittent and also for continuous stimulation. The manufacturer installed a special program on the neuromonitor for the recordings on the DLT program, such that a multi-channel recording was available.
Intraoperative nerve stimulation
Ventilation of the left lung was interrupted before opening the thorax. Anterolateral left-sided thoracotomy was performed and the thoracic cavity was inspected. The course of the vagus nerve was identified (Figure 2). The pleurae were dissected next to the nerve, the nerve was carefully mobilised with a blunt instrument and looped. The Saxophon® stimulation electrode was positioned around the nerve (Figure 3). This electrode consists of a conductor enveloped in silicone and clings to the nerve without exerting any pressure.
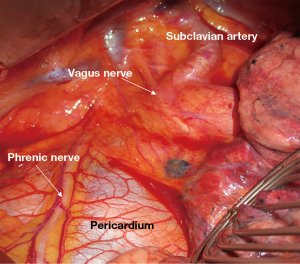
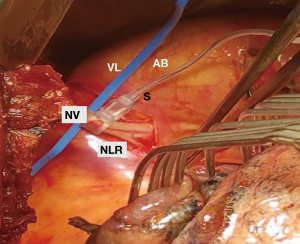
The vagus nerve was stimulated with a current of 1–2 mA. This corresponds to the manufacturer’s and the guideline’s recommendation for routine use during thyroid surgery (17,18). If the nerve stimulation and recording are functioning correctly, the signal picked up by the proximal electrode is displayed on the monitor as a white curve and that of the distal electrode as a yellow curve. Based on information in the literature (17,19), we considered an amplitude of 165 µV as a significant recording signal. Reference values for the latency periods after cervical stimulation were also found in the above-mentioned literature. Owing to the anatomical course, the latency of the vagus nerve following cervical stimulation is significantly longer than that of the RLN. Reference values for the latency of the vagus nerve and the RLN following thoracic stimulation have not yet been defined. However, we expect to see values between those of the vagus nerve and the RLN following cervical stimulation, which according to the literature vary from mean 3.6 to 7.4 ms.
According to the literature we defined a loss of signal as a decrease of the amplitude <100 µV (17).
Documentation: Encrypted patient data, type of surgery, tube size, depth of intubation from the incisors (IN), and the position of the electrodes on the tube were documented. We additionally noted special features or difficulties. The signal recorded on the monitor was printed from the neuromonitor (Figure 4).
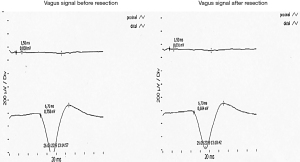
Evaluation
Proportions in percentages of the signals recorded by electrodes E1 and E2 were evaluated. In the so called OD group a signal was recorded only over the distal electrode E1. In addition, we performed a sub-group analysis of the patients’ characteristics regarding the tube sizes.
Statistical analysis
Qualitative data was described using number and percentage. Distributed data was expressed as range (minimum and maximum), mean, standard deviation and median.
Results
A total of 20 patients (11 men and 9 women; mean age 64 years; mean height 170 cm) were included in the study (March 2019 to October 2019). Fourteen of the 20 patients had been diagnosed with a malignant and 6 patients with a benign pulmonary disease in the finished pathological examination after surgery. A left-sided DLT was used in 18 procedures and a right-sided DLT in 2 procedures. Three size Ch 35, seven size 37 Ch, ten size Ch 39 and two size Ch 41 tubes were used. The mean depth of intubation from the incisors was 29.00 cm (range, 26–38 cm).
The mean distance between the proximal cuff and the distal electrode E1 was 10.00 mm (E1C; range, 0–18 mm). The mean distance between the distal and the proximal electrode E2 was 13.00 mm (E1E2; range, 0–26 mm). The mean distance between the proximal electrode E2 and the proximal cuff is 73.00 mm (E2C; range, 50–82 mm).
In two cases, in contrast to the acoustic signal, we were not able to record a signal above 165 µV with either the proximal or the distal electrode. In patient No. 13 (female, depth of intubation 27 cm), we reached only 23 µV on the proximal and 86 µV on the distal electrode. In patient No. 18 (male, intubation depth 31 cm) we recorded 145 µV on the proximal and 104 µV on the distal electrode. While the distance from the proximal cuff to the distal electrode was measured 12 mm in patient No. 13, it was measured 8mm in patient No. 18. In case 13 a left-sided DLT and in case 18 a right-sided tube was used.
Therefore in 18 of 20 (90%) patients, the distal electrode E1 recorded a significant signal with an amplitude of at least 165 µV throughout the entire surgery. In four of these 18 patients, a signal was additionally recorded by the proximal electrode E2. Conversely, therefore, in 14 of 18 cases (77.78%) [= group only distal (OD)], no signal was recorded by the proximal electrode E2. Table 2 shows an overview of the results.
Table 2
Electrode | No. of signals | % |
---|---|---|
E1+ | 18 | 90.00 |
E1+E2+ (B) | 4 | 22.22 |
E1+ (OD) | 14 | 77.78 |
E2+ (OP) | 0 | 0.00 |
E1, distal electrode; E2, proximal electrode; +, significant signal recorded; B, both; OD, only distal; OP, only proximal.
Provided that all RLNs were intact during the neuromonitoring, the sensitivity for signal detection is 90% for electrode E1 and 22.22% for electrode E2.
In group OD, the mean distance of the distal electrode to the proximal cuff was 10.86 mm, compared to 10.1 mm in the group of all cases where a significant signal was recorded.
Regarding the latencies the recorded data ranged from 3.3 to 6.7 ms on the distal electrode, therefore in 19 of 20 cases we recorded latencies in the expected mean time interval (3.6 to 7.4 ms). Only in one patient the latency was 3.3 ms (female, 67 years old, 172 cm, left-sided DLT, Ch 39, 1,086 µV proximal electrode, 53 µV distal electrode). in the two cases we did not record any significant signal of 165 µV on either electrode, the latencies were measured at 4.3 ms (patient No. 13, 86 µV distal electrode, 23 µV proximal electrode) and 6.2 ms on the distal electrode (patient No. 18, 104 µV distal electrode, 145 µV on the proximal electrode).
The sub-group analysis of all cases with a significant signal revealed that in the group where a signal was recorded only by the distal electrode E1 and not by the proximal electrode E2 (group OD, n=14), a left-sided DLT was used in all cases (DLT-L). In the group where a signal was additionally recorded by the proximal electrode E2 [group both (B), n=4], a right-sided tube (DLT-R) was used in one case and a left-sided tube in three cases.
In this regard, there was no difference regarding the depth of intubation in both groups, with a standard deviation of 3.58 cm and a mean depth of intubation of 29.50 cm (29.14 cm group OD vs. 30.50 cm group B). Table 3 shows a comparison of the key data of these two sub-groups.
Table 3
Signal (n=18) | Group OD = E1+ and E2− (n=14) | Group B = E1+ and E2+ (n=4) |
---|---|---|
Male | 7 (50.00) | 3 (75.00) |
Female | 7 (50.00) | 1 (25.00) |
DLT-L | 14 (100.00) | 3 (75.00) |
DLT-R | 0 (0.00) | 1 (25.00) |
Charrière 35 | 2 (14.28) | 0 (0.00) |
Charrière 37 | 5 (35.71) | 2 (50.00) |
Charrière 39 | 5 (35.71) | 2 (50.00) |
Charrière 41 | 2 (14.28) | 0 (0.00) |
Mean depth in cm from IN | 29.14 [26–37] | 30.5 [27–38] |
Mean E1C in mm | 10.86 [9–18] | 7.50 [0–11] |
Mean E1E2 in mm | 13.57 [10–22] | 8.75 [0–13] |
Mean E2C in mm | 72.47 [71–84] | 66.25 [50–73] |
Malignant | 10 (71.43) | 3 (75.00) |
Not malignant | 4 (28.57) | 1 (25.00) |
Mean age (years) | 61 [28–80] | 71 [67–79] |
Mean height (cm) | 162.79 [153–191] | 172.50 [167–179] |
Mean weight (kg) | 64.36 [46–98] | 58.75 [49–74] |
Data are shown as n (%) or mean [range]. +, signal recorded; −, no signal recorded; DLT-R, right-sided tube; DLT-L, left-sided tube; E1, distal electrode; E2, proximal electrode; C, proximal tube cuff; IN, from the incisors; Group OD, a signal was recorded only over the distal electrode E1.
In two cases we observed a reversible decrease of the amplitude with a resulting increase in latency and lowered sound level when putting tension on the lung tissue. One of them was observed in case 17. Here the initial signal, which was significant, measured at 505 µV on the distal electrode decreased by pulling on the left upper lobe. The second decreasing signal was monitored in case 18. Although we initially did not record a signal above 165 µV (145 µV) which was therefore not significant, we noticed a decreasing volume of the typical knocking sound by pulling on the tissue while dissecting the lymph nodes in the aortopulmonary space. In both cases the signal recovered immediately by releasing the tension and the amplitude and latency immediately returned to the initial values. In none of the cases did we observe a loss of signal, defined in the literature (20) as a complete signal failure with a drop in amplitude to below 100 µV.
A postoperative phoniatric examination confirmed a regular mobility of the vocal cords in all 20 patients.
Discussion
The first authors described the use of cIONM in lung surgery when using a DLT were Chai et al. (21) in 2020. They used only one recording electrode. Following trial intubation with the DLT, the level of the vocal cords was marked on the tube. After extubation, the electrode was affixed to the tube and the patient re-intubated. This method enabled him to perform continuous neuromonitoring in all patients with a signal amplitude of >500 µV. It is critical that the patient had to be intubated several times, each time harboring the potential risk of injury, until finally the electrode was positioned correctly. The results of our study demonstrate that we now for the first time have an easily reproducible technique at our disposal to solve this issue.
Our study in 20 patients is the second one describing cIONM of the laryngeal nerve in left-thoracic surgery showed that cIONM is feasible in at least 90% of our cases. The function of the RLN was demonstrated by stimulation of the vagus nerve above the aortic arch. We had bridged a longer segment of the tube above the proximal cuff by affixing two electrodes. Our results clearly demonstrated that the recording electrode should be affixed to the recommended left-sided DLT at least 10 mm, better yet 11 mm, above the proximal cuff. In the future, the second adhesive electrode can therefore be omitted. In all cases, intraoperative neuromonitoring was available for the entire duration of the surgery, irrespective of the actual procedure performed. We did not record any adverse EMG event (mild, severe, loss of signal) and were able to demonstrate a regular function of the RLN verified by phoniatric examination in all cases, which also shows the safety of our method. The continuous neuromonitoring technique described by us is easy to perform, reliable, and meets all the requirements in the surgical setting.
Other authors’ attempts at establishing intraoperative monitoring in the context of one-lung ventilation have not been very successful to date. In 2001, in a case report on abdominothoracic esophageal resection, Hemmerling et al. were able to record a signal from the RLN using two adhesive electrodes rotated at a 90° angle to one another on the DLT. However, they used transcutaneous, intermittent nerve stimulation (22). Zhao et al. (23) carried out intermittent neuromonitoring in left-sided thoracotomy procedures involving one-lung ventilation. He used a pre-assembled endotracheal tube. These tubes are sold by the respective manufacturers with the electrodes already placed. It is therefore presumed that the one-lung ventilation was carried out with an endobronchial blocker, but this was not specified. He described a signal amplitude that was slightly below that reported in the literature. This result was confirmed by Moritz et al. (20), who also used a pre-assembled endotracheal tube with a bronchial blocker for one-lung ventilation, as well as only intermittent neuromonitoring. Moritz et al. also reported 100 µV as a significant amplitude for the signal. Randolph et al. (17) described a variance in the signal amplitude of the vocalis muscle between 100–800 µV during phonation in an alert state. Other signal amplitude measurements described in the literature were at least 165 µV (17,19), which is why we used this value as a reference value in our study and defined it as significant. Because intermittent neuromonitoring—contrary to continuous neuromonitoring—does not provide an immediate control of injury to the nerve, it appears less suitable and therefore somewhat inferior in terms of its predictive value concerning the function of the nerve (8,16).
At this point we would also like to highlight some possible difficulties. Exposing the vagal nerve must be done as carefully as possible and without grasping the nerve directly. In patient 13 we recorded an acoustic signal of neuromonitoring, but did not reach our defined limit of at least 165 µV. According to the surgical report and the histological findings, the pleura was chronically thickened as to be seen in chronic inflammation due to multiple infections.
If this is the case, the pleura should be opened up in the area in which the nerve is suspected and then moved aside. When faced with this problem, we also used a hand stimulation probe to narrow down the rough location of the nerve. To underrun the nerve we use a blunt, nearly orthogonally curved Overholt clamp. Under no circumstances must the clamp be used as a lever. Tension on the cable and thus on the probe should be avoided throughout the entire procedure in order to prevent nerve injury.
After repositioning the patient in a lateral position, the DLT can become dislocated. In our cohort, this happened in two patients. In one case it was discovered due to an increased leak volume during ventilation and in the other case due to insufficient one-lung ventilation. In both cases we were able to correct the position of the tube using the video laryngoscope and by increasing the depth of the intubation. After correcting the position, we were able to record a significant signal in the first patient; in the second patient (patient 18), with an amplitude of 104 µV, the signal remained below our specified limit.
When affixing the recording electrodes, care should be taken that no creases form. To ensure good adhesion of the electrodes, the tube must be dried before the adhesive electrode is affixed. This is meant to prevent detachment of the electrodes after intubation.
Larger, prospective trials will have to demonstrate whether the use of continuous, intraoperative, thoracic neuromonitoring (CIOTN) can significantly lower the rate of RLN paralysis during left-sided thoracic surgical procedures. In particular, its use in cardiac surgery and thoracic esophageal surgery should be assessed. The advantage of the technique is that the surgeon is given an acoustic signal confirming the function of the nerve, which effectively increases the safety of the dissection.
Conclusions
In many left-thoracic surgical procedures involving one-lung ventilation, the RLN appears vulnerable during dissection. To prevent postoperative RLN injury, the use of continuous, intraoperative neuromonitoring has been described as safe and successful despite the use of a DLT.
Acknowledgments
Funding: None.
Footnote
Reporting Checklist: The authors have completed the TREND reporting checklist. Available at https://jtd.amegroups.com/article/view/10.21037/jtd-22-1515/rc
Data Sharing Statement: Available at https://jtd.amegroups.com/article/view/10.21037/jtd-22-1515/dss
Peer Review File: Available at https://jtd.amegroups.com/article/view/10.21037/jtd-22-1515/prf
Conflicts of Interest: All authors have completed the ICMJE uniform disclosure form (available at https://jtd.amegroups.com/article/view/10.21037/jtd-22-1515/coif). The authors have no conflicts of interest to declare.
Ethical Statement: The authors are accountable for all aspects of the work in ensuring that questions related to the accuracy or integrity of any part of the work are appropriately investigated and resolved. The study was conducted in accordance with the Declaration of Helsinki (as revised in 2013). The responsible ethics committee of the Faculty of Human Medicine of Phillips University approved the study (ref. 134/18). All patients were briefed about the study and signed the informed consent.
Open Access Statement: This is an Open Access article distributed in accordance with the Creative Commons Attribution-NonCommercial-NoDerivs 4.0 International License (CC BY-NC-ND 4.0), which permits the non-commercial replication and distribution of the article with the strict proviso that no changes or edits are made and the original work is properly cited (including links to both the formal publication through the relevant DOI and the license). See: https://creativecommons.org/licenses/by-nc-nd/4.0/.
References
- Cetin F, Gürleyik E, Dogan S. Morphology and Functional Anatomy of the Recurrent Laryngeal Nerve with Extralaryngeal Terminal Bifurcation. Anat Res Int 2016;2016:9503170. [Crossref] [PubMed]
- Chiang FY, Lu IC, Chen HC, et al. Anatomical variations of recurrent laryngeal nerve during thyroid surgery: how to identify and handle the variations with intraoperative neuromonitoring. Kaohsiung J Med Sci 2010;26:575-83. [Crossref] [PubMed]
- Chiang FY, Lu IC, Tsai CJ, et al. Does extensive dissection of recurrent laryngeal nerve during thyroid operation increase the risk of nerve injury? Evidence from the application of intraoperative neuromonitoring. Am J Otolaryngol 2011;32:499-503. [Crossref] [PubMed]
- Vural V, Comcali B, Saylam B, et al. Identification of the recurrent laryngeal nerve during thyroidectomy can affect the complication rate. Ann Ital Chir 2021;92:217-26.
- Bou-Malhab F, Hans S, Perie S, et al. Swallowing disorders in unilateral recurrent laryngeal nerve paralysis. Ann Otolaryngol Chir Cervicofac 2000;117:26-33.
- Marques JAS, Marronnier A, Crampon F, et al. Early Management of Acute Unilateral Vocal Fold Paralysis: Update of the Literature. J Voice 2021;35:924-6. [Crossref] [PubMed]
- Zumtobel M, End A, Bigenzahn W, et al. Reduced quality of life in patients with unilateral vocal cord paralysis after thoracic surgery. Chirurg 2006;77:518-22. [Crossref] [PubMed]
- Schneider B, Schickinger-Fischer B, Zumtobel M, et al. Concept for diagnosis and therapy of unilateral recurrent laryngeal nerve paralysis following thoracic surgery. Thorac Cardiovasc Surg 2003;51:327-31. [Crossref] [PubMed]
- Chiu CH, Wen YW, Chao YK. Lymph node dissection along the recurrent laryngeal nerves in patients with oesophageal cancer who had undergone chemoradiotherapy: is it safe? Eur J Cardiothorac Surg 2018;54:657-63. [Crossref] [PubMed]
- Taenaka H, Shibata SC, Okitsu K, et al. Perioperative factors related to the severity of vocal cord paralysis after thoracic cardiovascular surgery: A retrospective review. Eur J Anaesthesiol 2017;34:425-31. [Crossref] [PubMed]
- Ohta N, Kuratani T, Hagihira S, et al. Vocal cord paralysis after aortic arch surgery: predictors and clinical outcome. J Vasc Surg 2006;43:721-8. [Crossref] [PubMed]
- DiLisio RP, Mazzeffi MA, Bodian CA, et al. Vocal cord paralysis after aortic surgery. J Cardiothorac Vasc Anesth 2013;27:522-7. [Crossref] [PubMed]
- Kim TH, Park B, Cho JH, et al. Pneumonectomy for Clinical Stage I Non-Small Cell Lung Cancer in Elderly Patients over 70 Years of Age. Korean J Thorac Cardiovasc Surg 2015;48:252-7. [Crossref] [PubMed]
- Phelan E, Schneider R, Lorenz K, et al. Continuous vagal IONM prevents recurrent laryngeal nerve paralysis by revealing initial EMG changes of impending neuropraxic injury: a prospective, multicenter study. Laryngoscope 2014;124:1498-505. [Crossref] [PubMed]
- Stankovic P, Wittlinger J, Georgiew R, et al. Continuous intraoperative neuromonitoring (cIONM) in head and neck surgery-a review. HNO 2020;68:86-92. [Crossref] [PubMed]
- Sedlmaier A, Steinmüller T, Hermanns M, et al. Continuous versus intermittent intraoperative neuromonitoring in complex benign thyroid surgery: A retrospective analysis and prospective follow-up. Clin Otolaryngol 2019;44:1071-9. [Crossref] [PubMed]
- Randolph GW, Dralle H, et al. Electrophysiologic recurrent laryngeal nerve monitoring during thyroid and parathyroid surgery: international standards guideline statement. Laryngoscope 2011;121:S1-16. [Crossref] [PubMed]
- Dralle H, Lorenz K, Schabram P, et al. Intraoperative neuromonitoring in thyroid surgery. Recommendations of the Surgical Working Group for Endocrinology. Chirurg 2013;84:1049-56. [Crossref] [PubMed]
- Lorenz K, Sekulla C, Schelle J, et al. What are normal quantitative parameters of intraoperative neuromonitoring (IONM) in thyroid surgery? Langenbecks Arch Surg 2010;395:901-9. [Crossref] [PubMed]
- Moritz A, Schmidt J, Schreiner W, et al. Combined recurrent laryngeal nerve monitoring and one-lung ventilation using the EZ-Blocker and an electromyographic endotracheal tube. J Cardiothorac Surg 2019;14:111. [Crossref] [PubMed]
- Chai YJ, Lee JM, Seong YW, et al. Application of Continuous Intraoperative Neuromonitoring During VATS Lobectomy for Left Lung Cancer to Prevent Recurrent Laryngeal Nerve Injury. Sci Rep 2020;10:4636. [Crossref] [PubMed]
- Hemmerling TM, Schmidt J, Jacobi KE, et al. Intraoperative monitoring of the recurrent laryngeal nerve during single-lung ventilation in esophagectomy. Anesth Analg 2001;92:662-4. [Crossref] [PubMed]
- Zhao J, Xu H, Li W, et al. Intraoperative recurrent laryngeal nerve monitoring during surgery for left lung cancer. J Thorac Cardiovasc Surg 2010;140:578-82. [Crossref] [PubMed]