Management of primary graft dysfunction after lung transplantation with extracorporeal life support: an evidence-based review
Primary graft dysfunction (PGD) is a syndrome of acute organ dysfunction in the early post-lung transplantation (LTx) period. Currently, there are no interventions that reliably treat or prevent PGD. However, supportive measures can significantly impact the outcomes of lung transplant recipients suffering from severe PGD. In this review, we provide an overview of the diagnosis, epidemiology, and pathophysiology of PGD after lung transplantation and describe the management of PGD with extracorporeal life support (ECLS), including criteria for instituting ECLS, cannulation strategies, and recent outcomes in the use of ECLS as a bridge to recovery (BTR) from PGD.
Definition and diagnosis
PGD is defined as opacifications noted on imaging in the setting of a decreased ratio of partial pressure of arterial oxygen (PaO2) to fraction of inspired oxygen (FiO2), commonly referred to as P/F ratio, within the first 72 hours post-transplantation without another identifiable cause (1).
Post-transplant patients are assessed for PGD at 4 time points, first at the time of reperfusion of the second lung and then at 24, 48, and 72 hours after transplant (1). Assessment and grading is based on chest radiograph (evidence of diffuse pulmonary opacities in at least one of the allografts) and on the P/F ratio, ideally measured with a positive end-expiratory pressure (PEEP) of 5 cmH2O at a FiO2 of 1.0 (Table 1) (1). All post-transplant patients receive a PGD grade. Patients without opacities on chest radiograph are classified as PGD grade 0 and are clinically considered to not have PGD. Patients with opacities on chest radiograph and P/F ratio of >300 are classified as PGD grade 1, patients with opacities and P/F ratio of 200–300 as PGD grade 2, and patients with opacities and P/F ratio of <200 as PGD grade 3. Patients with opacities on chest radiograph while on ECLS are automatically classified as PGD grade 3. Patients on ECLS without opacities on chest radiograph are considered ungradable (Table 1) (1).
Table 1
Grade at T0, T24, T48, T72 (hours) | Opacities on CXR | P/F | Revision |
---|---|---|---|
0 | No | Any | – |
1 | Yes | >300 | – |
2 | Yes | 200–300 | – |
3 | Yes | <200 | – |
3 | Yes | – | If on ECLS |
Ungradable | No | – | If on ECLS |
Note: (I) Patients not mechanically ventilated should have P/F ratio calculated in similar manner as those being ventilated; (II) inhaled nitric oxide or other pharmacologic agents that may improve oxygenation should not change grading methods; (III) P/F ratio should ideally be measured on positive end expiratory pressure of 5 cmH2O at FiO2 of 1.0 while patients are on mechanical ventilation; (IV) T0 starts at reperfusion of second lung. Adapted from 2016 ISHLT consensus statement on Primary Graft Dysfunction (1). CXR, chest X-ray; P/F, partial pressure of arterial oxygen to fraction of inspired oxygen; FiO2, fraction of inspired oxygen; ECLS, extracorporeal life support.
PGD is a diagnosis of exclusion. Therefore, patients presenting with hypoxia and lung opacities in the immediate post-transplant period must undergo a complete workup to exclude conditions which may present in a similar manner, including hyperacute rejection, volume overload, left ventricular dysfunction, pulmonary venous outflow tract obstruction, aspiration pneumonia, and transfusion-related acute lung injury (1). The work up for suspected PGD will typically include assessment of cardiac function [transthoracic or transesophageal echocardiogram (TEE)], airway obstruction from secretions or mucous plugs (bronchoscopy), and lung pathology [chest computed tomography (CT)] (1).
Epidemiology and pathophysiology
The 2016 consensus statement by the International Society for Heart and Lung Transplantation (ISHLT) PGD working group describes the incidence of PGD to be between 15% and 57% and the incidence of severe PGD between 15% and 20% (2). Severe PGD accounts for approximately 30% of patient mortality in the first month and 50% within the first year of transplant (3,4). PGD has been linked to the development of bronchiolitis obliterans syndrome, the predominant form of chronic lung allograft dysfunction (CLAD) and a major determinant of long-term outcomes in lung transplant recipients. Overall, despite significant advancements in patient selection, donor selection, pre-operative optimization, surgical technique, and post-transplant care, PGD is still a major contributor to overall morbidity, mortality and poor allograft function in lung transplant recipients (3-6).
Pathogenesis of PGD is multifactorial. Donor lungs are vulnerable to injury at multiple time points beginning at donor brain death, which is associated with an inflammatory cascade that is particularly detrimental to lung function (7). Following organ recovery, an obligatory period of ischemic cold storage followed by organ reperfusion plays a critical role in the development of PGD via generation of reactive oxygen species (ROS) (5). It is hypothesized that chemokines generated from oxidative stress recruit and activate innate immune cells, driving antigen presentation and resulting in chronic inflammation (6,8). Risk of PGD depends on the severity of ischemic injury, measured as warm and cold organ ischemic time. Prolonged ischemic time has been associated with the need for post-operative ECLS for refractory grade 3 PGD (9). To minimize the deleterious effects of ischemic time, donor organs are transported cold in a preservation fluid at 4 to 8 degrees Celsius and limit the duration of cold ischemic time to less than 8 hours (10). Recent research has challenged this convention by relaxing the preservation temperature to 10 degrees Celsius and widening the cold ischemic time to greater than 8 hours (11). The donor lungs are transported while inflated with an FiO2 between 0.3 to 0.5 to facilitate aerobic metabolism—a practice that may also increase the production of reactive oxygen species (10,12). Allograft ischemia is further potentiated by hypoperfusion, as bronchial arteries are typically not re-anastomosed during transplant. New evidence is emerging supporting warmer preservation temperatures, up to 10 degrees Celsius, that can not only improve organ function by producing cytoprotective metabolites but can also prolong preservation time up to 16 hours without deleterious effects (11).
PGD is also driven by non-ischemic mediators of graft injury. Both recipient and donor characteristics have been implicated. Recipient characteristics linked with an increased risk of PGD include female gender, African American descent, diagnosis of idiopathic pulmonary fibrosis or sarcoidosis, primary pulmonary hypertension (PH), and body mass index greater than 25 (13-15). Donor characteristics linked to PGD include age greater than 65, blood transfusion (greater than or equal to 3 units of packed red blood cells (PRBCs) shortly before organ donation, and chronic tobacco or alcohol use (16-22). These donor and recipient characteristics are thought to represent acute and chronic stressors that contribute to the development of PGD by potentiating inflammation (23-25).
Role of ECLS in PGD
The first reports of ECLS for PGD date back to 1978, when Dr. Cooper and his colleagues in Toronto performed a single right lung transplantation on a 19 year-old burn victim supported preoperatively with ECLS. ECLS was continued intraoperatively and then extended postoperatively for 96 hours to allow organ recovery from reperfusion injury. The patient was successfully weaned off ECLS and was ambulatory without supplemental oxygen by postoperative day 10 (26).
While approximately 5% of recipients in the UNOS registry are reported to require ECLS post-LTx (8), this number is likely to increase in the future as higher-risk candidates become prioritized on the waitlist (27). There are currently no consensus guidelines for using ECLS post-transplantation, and frequency and threshold for instituting ECLS post-implantation varies widely from institution to institution. A number of high-volume centers have described their approach to ECLS deployment in this setting (28-32). There are two main indications for ECLS post-transplantation: (I) treatment of severe PGD; or (II) prevention of PGD. Because extracorporeal membrane oxygenation (ECMO) is the terminology used in nearly all the reports in reference to PGD, we will refer to ECLS as ECMO for the remainder of the discussion.
ECMO as a BTR for severe PGD (treatment of PGD)
Patients with grade 1 and 2 PGD can typically be conservatively managed with fluid restriction, diuresis, lung-protective ventilation, and empiric antibiotics. However, patients with refractory hypoxemia may benefit from the addition of pulmonary vasodilator therapy, such as inhaled nitric oxide (iNO) or epoprostenol. One center recommends high-frequency jet ventilation, a steady administration of prostacyclins or surfactant into the lungs (29). However, when supportive medical therapy and ventilatory support fails, patients may require initiation of ECMO as a BTR. The deployment of ECMO relies on a rapid and accurate assessment of the primary underlying issue: (I) PGD without cardiac or hemodynamic instability; (II) primary cardiac dysfunction and/or hemodynamic instability without PGD; or (III) a mixed picture of cardiopulmonary dysfunction.
ECMO for PGD without cardiac or hemodynamic instability
Criteria for ECMO institution
Despite the lack of uniform criteria for initiating ECMO for severe PGD, specific clinical recommendations can still be offered based on the currently available literature. Some providers consider initiating ECMO for worsening general trends in laboratory and clinical parameters, such as progressive hypoxemia despite optimized ventilatory support, worsening intraoperative and postoperative P/F ratios, new iNO requirements, worsening radiographic imaging, and frothy pulmonary edema on bronchoscopy (33). Others initiate ECMO based on specific and set clinical criteria, such as P/F less than 100 (34), peak inspiratory pressures reaching 35 cmH2O, or FiO2 exceeding 0.6 required to maintain adequate oxygenation (35). All efforts are focused on protecting the allograft from the injurious effects of aggressive ventilation and oxidative stress from high FiO2, allowing the lungs time to rest and recover.
Type of ECMO and cannulation strategy
Although veno-arterial (V-A) ECMO is most commonly employed intraoperatively during implantation, veno-venous (V-V) ECMO is the preferred strategy for PGD without hemodynamic dysfunction or right ventricular (RV) compromise (30,35-37). The Duke group recommends V-V ECMO even when there are signs of early right heart dysfunction and elevated pulmonary arterial (PA) pressures, as they believe that improving gas exchange will resolve hypoxia- and acidosis-driven pulmonary vasoconstriction (35). In the absence of hemodynamic fluctuations or PH, surgeons may elect to transition from intraoperative V-A ECMO to either central V-V ECMO [authors’ institutional experience with right atrial (RA) to PA cannulation] or to peripheral V-V ECMO by one of three methods: (I) bilateral femoral venous cannulation (30); (II) femoral vein-internal jugular (IJ) venous cannulation (35); or (III) dual-lumen single site cannulation of the internal jugular vein (right or left) (35). The choice of cannulation depends on patient factors, including anatomy (patency and accessibility of femoral or internal jugular veins), physiology (degree of hypoxia and need for higher flows), and rehabilitation plans, as well as on surgeon experience and preference.
Advantages and pitfalls of V-V ECMO
One major advantage of V-V ECMO is that it maintains the physiologic direction of well-oxygenated, pulsatile blood flow through the pulmonary vascular bed, allowing the lung parenchyma time to recover from hypoxic and ischemic insult (38). The advantage of central V-V (RA to PA) ECMO over peripheral V-V ECMO is that central V-V ECMO can unload the RV in the event of hypoxia-driven RV dysfunction while maintaining circulation through the left side of the heart. Despite these advantages, large cannulae in low pressure venous systems can impede flow leading to venous stasis, narrowing, or occlusion and subsequent deep venous thrombosis, complicating patient recovery and increasing the need for systemic anticoagulation. Also, central V-V ECMO requires partial chest closure, increasing the risks of bleeding, infection, and prolonged sedation and/or paralysis with resultant extended bedrest.
ECMO for PGD with cardiac or hemodynamic instability (cardiopulmonary dysfunction)
Criteria for ECMO institution
Post-LTx PGD complicated by RV dysfunction can occur in recipients with or without pre-existing PH. In addition to hypoxia, clinical signs may include hemodynamic instability upon weaning from intraoperative extracorporeal support [ECMO or cardiopulmonary bypass (CPB)], increasing inotrope requirements, increasing pulmonary arterial pressure, RV dysfunction on TEE, ventricular tachycardia, premature ventricular contractions, lactic acidosis, and decline in mixed venous oxygen (SvO2) (38). However, careful evaluation of other etiologies of hemodynamic instability (e.g., hemorrhagic, cardiogenic, anaphylactic, or distributive shock), with particular attention to TEE to rule out new valvular abnormalities or wall-motion abnormalities, should be carried out prior to diagnosis of PGD with cardiac dysfunction.
Type of ECMO and cannulation strategy
For those with cardiac and/or hemodynamic instability with PGD, V-A ECMO is the support of choice. There are two possible cannulation strategies, each with advantages and disadvantages: (I) continuation of or transition (from central CPB) to central V-A ECMO (right atrium to aorta); or (II) transition from central to peripheral femoral V-A ECMO.
Advantages and pitfalls of central vs. peripheral femoral V-A ECMO
Central V-A ECMO with an ‘open’ chest not only eliminates the need for another operative site and procedure (peripheral femoral V-A ECMO), but also diminishes some of the physiologic and mechanical derangements on the edematous allografts from primary chest closure (39). Though there is a theoretical concern for mediastinal infection in patients with partial chest closures, several studies have not demonstrated a difference in deep wound infection in patients with open chests (40,41). Additionally, central V-A ECMO maintains antegrade flow of oxygenated blood to the coronaries and brain, avoiding the Harlequin syndrome associated with femoral V-A ECMO (27). Furthermore, femoral V-A ECMO is associated with significant vascular complications including limb-threatening arterial and venous insufficiency from stenosis and/or thrombosis (42). On the other hand, central cannulation with an open chest frequently requires sedation and/or paralysis while femoral cannulation may not. In the end, the decision between central versus peripheral femoral V-A ECMO is made based upon the patient’s anatomy (including size of the femoral vessels and risk of vascular insufficiency) and physiological needs (as central ECMO’s larger cannula size provides higher flow), alongside surgeon preference and experience.
ECMO for prevention of PGD and/or to facilitate cardiac remodeling
Prevention is the best intervention. In addition to preoperative planning and acknowledgment of donor and recipient factors, intraoperative choices can prevent or reduce the risk of PGD. Ongoing work with portable and non-portable ex vivo lung perfusion platforms have made notable benefits on graft function in the short term, though the impact of these devices in the long-term still have not demonstrated superiority to conventional preservation methods. The high costs of these devices also restrict their use. Thus, long term follow up is needed to clarify the effectiveness and role of EVLP in prevention of PGD (43).
The use of CPB during lung transplantation has also been linked to an increased risk of PGD. A large meta-analysis of 13 studies over four decades, as well as a multicenter prospective study by the Lung Transplant Outcomes Group examining the risk factors for PGD, found the use of CPB to be an independent risk factor for PGD (OR 3.4; 95% CI: 2.2–5.3; P<0.001) (13,14). A more recent study including 10 high-volume centers (>40 LTx/year) around the globe compared intraoperative mechanical circulatory support (CPB and ECMO) with off-pump LTx. ECMO was associated with a lower incidence of PGD compared to CPB (28.9% vs. 42.7%), but a higher incidence than off-pump LTx (12%) (44). There are no prospective randomized trials comparing ECMO to CPB. Several single-center retrospective studies have shown lower transfusion rates, decreased renal impairment, and reduced need for reintubation with intraoperative ECMO as compared to CPB (45-47). For these reasons, alongside recent improvement in ECMO technology, ECMO has replaced CPB in most centers as the intraoperative support strategy of choice during lung transplant surgery.
While only 30% to 40% of patients require mechanical circulatory support during lung transplantation for either cardiac, pulmonary, or cardiopulmonary support, emerging evidence suggests that routine use of ECMO intraoperatively can diminish or prevent PGD (48). By reducing blood flow through the allograft vascular bed, fluid extravasation and further impairment of pulmonary function can be decreased. Therefore, in some practices (including authors’), controlled reperfusion of the allograft by ‘slow’ ECMO wean intraoperatively after implantation has become standard practice (49-52). In fact, the lung transplant team at the Medical University of Vienna in Austria now not only performs all lung transplantations on central V-A ECMO, but also extends ECMO to the postoperative setting (transitioning from central to peripheral femoral V-A) for a period of hours to days to facilitate prolonged controlled reperfusion of the allograft. They report excellent outcomes with a decrease in the frequency of grade 3 PGD (1.3% versus 15% to 20% ISHLT reported rates in the same time period) with a global 2-year survival rate of 86% (53).
The concomitant use of intra- and post-operative V-A ECMO is also advocated for prevention of PGD in patients with pulmonary arterial hypertension (PAH) or WHO group 3 PH undergoing bilateral lung transplant. Patients with PH undergoing LTx carry a high risk of early procedural morbidity (3.5-fold increased risk of PGD) (13,16) and mortality (54) attributed to PGD. The mechanism of PGD in PH is likely multifactorial, including pulmonary edema secondary to endothelial injury as well as left ventricular deconditioning in PH requiring acclimatization to a new ‘normal’ preload after transplantation. Tudorache and colleagues from Hanover, Germany published their experience with prophylactic extension of V-A ECMO post-transplant for PH. The median duration of mechanical ventilation (P<0.0001), intensive care unit length of stay (P<0.0005) and median total length of stay post-transplantation (P<0.0023) were all shorter in the group who had V-A ECMO extended post-operatively. The 90-day survival was 100% in the V-A ECMO extension group compared to 85% in the group that did not receive extended ECMO, though this finding did not reach statistical significance (55).
Outcomes of PGD with ECMO support
The ISHLT registry reports a 1- and 5-year survival rate of 85% and 59%, respectively, for those undergoing lung transplant, with some individuals surviving up to 10 years post-transplant (56). However, outcomes for patients who experience PGD requiring ECMO are inferior.
Survival outcomes
Early morbidity and mortality for PGD is high. Several studies have reported 30- and 90-day mortality from severe PGD to be roughly between 23% to 35% (13,57,58). Similarly, long term survival is diminished in those with severe PGD with 1-year survival ranging between 35% to 73% (59,60). While there are no prospective randomized trials comparing outcomes of severe PGD managed with and without ECMO, several series have specifically examined the impact of ECMO on both short and long-term outcomes. For example, the Pittsburgh group reviewed their experience with ECMO for PGD and compared it those who did not require ECMO. The ECMO group’s 30-day survival was 56%. The 1- and 5-year survival in patients requiring ECMO was significantly lower than those who did not require ECMO (40% and 25% vs. 82% and 52%, respectively) (30). Hartwig and colleagues published their experience exclusively with V-V ECMO for severe PGD. The 30-day graft survival for the ECMO group was 82% compared to 97% for those who did not require ECMO. The 3- and 5-year survival was 49% for patients receiving ECMO while the no-ECMO group had survival rates of 74% and 61%, respectively (35). Bellier and colleagues from the Foch Hospital in France reported a 90-day mortality of 50% in patients receiving ECMO (36). Boffini and colleagues from the University of Torino in Italy examined the impact of post-transplant ECMO on outcomes over an 11-year period. ECMO after lung transplantation was an independent predictor of in-hospital mortality (44% ECMO vs. 8% without ECMO). The 1- and 5-year survival were also significantly impacted by post-transplant ECMO [hazard ratio (HR) 5.5, P<0.001 and HR 3.5, P<0.001 for 1- and 5-year, respectively] (61).
These international studies demonstrate the reproducible finding that while ECMO BTR is a feasible option in a cohort with an otherwise dismal outlook, the short- and long-term outcomes are poorer compared to patients who do not require ECMO. A summary of representative studies on ECMO as a BTR from PGD is presented in Table 2.
Table 2
Authors, year of study | Total number of patients (N); incidence of ECMO | Type of ECMO and timing | ECMO for PGD survival outcomes | No ECMO survival outcomes |
---|---|---|---|---|
Meyers et al., 2000 | N=444; incidence: 2.7% | V-A ECMO: N=3 femoral access, N=9 central access | 58.33% to discharge | Not reported |
Wigfield et al., 2007 | N=286, incidence: 7.9% | V-A ECMO: Early: ECMO <24 h from transplant, N=17; Late: ECMO >24 h from transplant, N=5 | Early: 30-day: 74.6%, 1-year: 54%, 3-year: 36%. Late: 0% to discharge | 30-day: 97.5%; 1-year: 88.6%; 3-year: 73.8% |
Bermudez et al., 2009 | N=763; incidence: 7.6% | V-A ECMO: N=26. V-V ECMO: N=32, ECMO started within 7 days of transplant | V-A ECMO: 30-day: 58%; 1-year: 42%; 5-year: 29%. V-V ECMO: 30-day: 55%; 1-year: 39%; 5-year: 22% | 30-day not reported; 1-year: 82%; 5-year: 54% |
Hartwig et al., 2012 | N=498; incidence: 5.6% | V-V ECMO: N=28 | 30-day: 82%; 3-year: 49%; 5-year: 49% | 30-day: 97%; 3-year: 74%; 5-year: 61% |
Ius et al., 2018 | N=319; ECMO incidence: 16%, CBP incidence: 14% | V-V ECMO: N=52; CBP: N=46 | 90-day: 81%; 1-year: 81% | CBP group: 90-day: 70%, 1-year: 56% |
Boffini et al., 2019 | N=195; incidence: 12.8% | V-V ECMO: N=25 | HR, ECMO vs. no ECMO: 1-year, 5.5 (95% CI: 3–10); 5-year, 3.5 (95% CI: 2–6) | – |
Bellier et al., 2019 | N=211; incidence: 11.4% | V-A ECMO: N=23; V-V ECMO: N=1 | 90-day: 50% | Grade 1 & 2 PGD: 90-day: 89% & 91% |
Harano et al., 2021 | N=1,049; incidence: 9.15% | V-A ECMO: N=18; V-V ECMO: N=78 | 90-day: 67.3%; 1-year: 50%; 5-year: 31.5% | Not reported |
ECMO, extracorporeal membrane oxygenation; PGD, primary graft dysfunction; V-A, veno-arterial; V-V, veno-venous; CBP, cardiopulmonary bypass; HR, hazard ratio.
Impact of timing of ECMO deployment on outcomes
The timeliness of instituting ECMO for PGD has been associated with favorable survival outcomes. The report from Nelems and colleagues from Toronto General Hospital in 1978 may be the first study advocating ‘early’ ECMO support for PGD, by extending intraoperative support to the postoperative setting (26). Since then, a variety of contemporary reports have supported early institution of ECMO for PGD. Meyers and colleagues described their experience with ECMO for PGD and concluded that early institution of ECMO increases the likelihood of success (62). Wigfield and colleagues reported outcomes of early (<24 h post transplantation) versus late (>24 h post transplantation) institution of ECMO. The early ECMO group had a survival rate of 67% and 49% at 1 and 5 years, respectively. In contrast, the late group had 100% mortality with a mean survival of 33 days (33). A more recent retrospective cohort study from the University of Pittsburgh focusing on time to initiation of ECMO for refractory PGD found that time to initiation of ECMO more than 48 hours post-transplant was independently associated with an increased risk of in-hospital, 1-year, and 3-year mortality (63).
While the definition of early and late ECMO timing varies from report to report, most studies support the institution of ECMO as soon as signs of graft failure become evident rather than using it as a last resort when all else has failed. Early institution of ECMO can limit the extent of ventilator-induced allograft injury by reducing barotrauma and exposure to oxygen free radicals.
Functional outcomes
Most studies of ECMO for PGD have focused on survival statistics, but a few studies have addressed the long-term impact on graft function. Virtually no studies have examined the quality-of-life following ECMO for PGD. Two studies demonstrated a significantly reduced forced expiratory volume in one second (FEV1) in patients who received ECMO (34,35), yet another found no difference in FEV1 at 1 or 2 years in those who were successfully weaned from ECMO compared to those who did not receive ECMO (30). Future studies should examine the long-term graft function and quality of life following ECMO BTR for PGD.
How we do it: V-A and V-V ECMO for PGD
At the University of California, San Francisco, the authors’ approach to post-LTx recovery is hallmarked by two simple guiding principles: (I) preventing PGD; and (II) ‘early’ treatment of PGD. To this end, we manage our patients immediately post-LTx by following the decision tree described in Figure 1. Beginning in 2013, our intraoperative mechanical support of choice was switched from full CPB to central V-A ECMO for most cases. Upon completion of the final anastomosis, the patient undergoes controlled reperfusion with obligatory ECMO wean over 10 to 15 minutes with the lungs ventilated at an FiO2 of 0.4, PEEP of 5–6 cmH2O, and tidal volumes of 6 cc/kg. During this reperfusion period, the surgeon continues to monitor and assess the allograft function (via oxygen saturation and ventilation, peak airway pressures, and bronchoscopic findings), hemodynamics, and RV function. Hypoxia, frothy pulmonary edema on bronchoscopy, lung parenchymal swelling, and elevated airway pressures are valuable clinical indicators of respiratory failure after lung transplantation. Trends in arterial blood gases are closely monitored as ECMO is weaned.
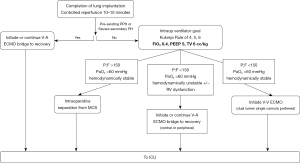
For the prevention of PGD
If the patient has a pre-existing diagnosis of PH (primary PH or WHO group 3 severe PH) or is on ECMO as a bridge to transplant for severe RV dysfunction, we strongly consider extending intraoperative V-A ECMO to the early postoperative period to allow prolonged controlled reperfusion and to facilitate LV remodeling.
For the treatment of PGD
Intraoperatively, once the period of controlled reperfusion is complete and the patient has been weaned off V-A ECMO, a P/F less than 150 in the absence of hemodynamic instability warrants strong consideration for V-V ECMO as a BTR from PGD. We prefer a dual lumen single cannula placed in the right internal jugular vein. Conversely, in patients with P/F less than 150 with the presence of hemodynamic instability and/or RV dysfunction, V-A ECMO is extended to the postoperative setting to support graft recovery, hemodynamics, and RV unloading. In contrast to the Vienna group practice, we frequently will continue central V-A ECMO with partial chest closure, as opposed to conversion to peripheral femoral V-A ECMO support. In our experience, central V-A ECMO and delayed chest closure do not increase post-transplant surgical-site infection or mortality (41). Finally, if the patient demonstrates a P/F greater than 150 without any hemodynamic instability or RV dysfunction, intraoperative separation from ECMO is performed.
Conclusions
This review highlights the feasibility of instituting early ECMO after lung transplant, summarizes the data available from major centers around the world, and provides a step-wise approach to bringing a patient from the immediate post-implantation period to the intensive care unit. Although this review is limited to observational retrospective and prospective studies, the data presented above summarize the currently available knowledge of worldwide practices. A randomized controlled trial has yet to be performed in this field of study but the current data validates V-A and V-V ECMO as support modalities for severe PGD. Modern practices for lung transplantation vary widely, but there is growing evidence that ECMO is accepted as a major tool for surgeons treating PGD. While it is important for surgeons to familiarize themselves with the different indications and strategies for instituting ECMO, emphasis should be placed on developing uniform strategies for approaching PGD in lung transplant recipients.
Acknowledgments
Funding: None.
Footnote
Provenance and Peer Review: This article was commissioned by the Guest Editor (Ilhan Inci) for the series “Extracorporeal Life Support in Thoracic Surgery” published in Journal of Thoracic Disease. The article has undergone external peer review.
Peer Review File: Available at https://jtd.amegroups.com/article/view/10.21037/jtd-22-1387/prf
Conflicts of Interest: All authors have completed the ICMJE uniform disclosure form (available at https://jtd.amegroups.com/article/view/10.21037/jtd-22-1387/coif). The series “Extracorporeal Life Support in Thoracic Surgery” was commissioned by the editorial office without any funding or sponsorship. JS received salary support from NIH T32 FAVOR Grant during the academic year 2022–2023 and will fund travel to ISHLT 2023 in Denver CO, USA on 19-22 April, 2023. The authors have no other conflicts of interest to declare.
Ethical Statement:
Open Access Statement: This is an Open Access article distributed in accordance with the Creative Commons Attribution-NonCommercial-NoDerivs 4.0 International License (CC BY-NC-ND 4.0), which permits the non-commercial replication and distribution of the article with the strict proviso that no changes or edits are made and the original work is properly cited (including links to both the formal publication through the relevant DOI and the license). See: https://creativecommons.org/licenses/by-nc-nd/4.0/.
References
- Snell GI, Yusen RD, Weill D, et al. Report of the ISHLT Working Group on Primary Lung Graft Dysfunction, part I: Definition and grading-A 2016 Consensus Group statement of the International Society for Heart and Lung Transplantation. J Heart Lung Transplant 2017;36:1097-103. [Crossref] [PubMed]
- Diamond JM, Arcasoy S, Kennedy CC, et al. Report of the International Society for Heart and Lung Transplantation Working Group on Primary Lung Graft Dysfunction, part II: Epidemiology, risk factors, and outcomes-A 2016 Consensus Group statement of the International Society for Heart and Lung Transplantation. J Heart Lung Transplant 2017;36:1104-13. [Crossref] [PubMed]
- Li D, Weinkauf J, Kapasi A, et al. Baseline lung allograft dysfunction in primary graft dysfunction survivors after lung transplantation. Respir Med 2021;188:106617. [Crossref] [PubMed]
- Keller M, Sun J, Mutebi C, et al. Donor-derived cell-free DNA as a composite marker of acute lung allograft dysfunction in clinical care. J Heart Lung Transplant 2022;41:458-66. [Crossref] [PubMed]
- Christie JD, Bavaria JE, Palevsky HI, et al. Primary graft failure following lung transplantation. Chest 1998;114:51-60. [Crossref] [PubMed]
- el-Sawy T, Fahmy NM, Fairchild RL. Chemokines: directing leukocyte infiltration into allografts. Curr Opin Immunol 2002;14:562-8. [Crossref] [PubMed]
- Barklin A. Systemic inflammation in the brain-dead organ donor. Acta Anaesthesiol Scand 2009;53:425-35. [Crossref] [PubMed]
- Hancock WW, Gao W, Faia KL, et al. Chemokines and their receptors in allograft rejection. Curr Opin Immunol 2000;12:511-6. [Crossref] [PubMed]
- Mulvihill MS, Yerokun BA, Davis RP, et al. Extracorporeal membrane oxygenation following lung transplantation: indications and survival. J Heart Lung Transplant 2017; Epub ahead of print. [Crossref] [PubMed]
- Van Raemdonck D, Hartwig MG, Hertz MI, et al. Report of the ISHLT Working Group on primary lung graft dysfunction Part IV: Prevention and treatment: A 2016 Consensus Group statement of the International Society for Heart and Lung Transplantation. J Heart Lung Transplant 2017;36:1121-36. [Crossref] [PubMed]
- Ali A, Wang A, Ribeiro RVP, et al. Static lung storage at 10°C maintains mitochondrial health and preserves donor organ function. Sci Transl Med 2021;13:eabf7601. [Crossref] [PubMed]
- Natalini JG, Diamond JM. Primary Graft Dysfunction. Semin Respir Crit Care Med 2021;42:368-79. [Crossref] [PubMed]
- Diamond JM, Lee JC, Kawut SM, et al. Clinical risk factors for primary graft dysfunction after lung transplantation. Am J Respir Crit Care Med 2013;187:527-34. [Crossref] [PubMed]
- Liu Y, Liu Y, Su L, et al. Recipient-related clinical risk factors for primary graft dysfunction after lung transplantation: a systematic review and meta-analysis. PLoS One 2014;9:e92773. [Crossref] [PubMed]
- Shaver CM, Ware LB. Primary graft dysfunction: pathophysiology to guide new preventive therapies. Expert Rev Respir Med 2017;11:119-28. [Crossref] [PubMed]
- Kuntz CL, Hadjiliadis D, Ahya VN, et al. Risk factors for early primary graft dysfunction after lung transplantation: a registry study. Clin Transplant 2009;23:819-30. [Crossref] [PubMed]
- Baldwin MR, Peterson ER, Easthausen I, et al. Donor age and early graft failure after lung transplantation: a cohort study. Am J Transplant 2013;13:2685-95. [Crossref] [PubMed]
- Bittle GJ, Sanchez PG, Kon ZN, et al. The use of lung donors older than 55 years: a review of the United Network of Organ Sharing database. J Heart Lung Transplant 2013;32:760-8. [Crossref] [PubMed]
- De Perrot M, Waddell TK, Shargall Y, et al. Impact of donors aged 60 years or more on outcome after lung transplantation: results of an 11-year single-center experience. J Thorac Cardiovasc Surg 2007;133:525-31. [Crossref] [PubMed]
- Bonser RS, Taylor R, Collett D, et al. Effect of donor smoking on survival after lung transplantation: a cohort study of a prospective registry. Lancet 2012;380:747-55. [Crossref] [PubMed]
- Berman M, Goldsmith K, Jenkins D, et al. Comparison of outcomes from smoking and nonsmoking donors: thirteen-year experience. Ann Thorac Surg 2010;90:1786-92. [Crossref] [PubMed]
- Elmaleh Y, De Tymowski C, Zappella N, et al. Blood transfusion of the donor is associated with stage 3 primary graft dysfunction after lung transplantation. Clin Transplant 2021;35:e14407. [Crossref] [PubMed]
- Khush KK, Potena L, Cherikh WS, et al. The International Thoracic Organ Transplant Registry of the International Society for Heart and Lung Transplantation: 37th adult heart transplantation report-2020; focus on deceased donor characteristics. J Heart Lung Transplant 2020;39:1003-15. [Crossref] [PubMed]
- Lowery EM, Kuhlmann EA, Mahoney EL, et al. Heavy alcohol use in lung donors increases the risk for primary graft dysfunction. Alcohol Clin Exp Res 2014;38:2853-61. [Crossref] [PubMed]
- Venkata-Subramani M, Nunley DR, Roman J. Donor factors and risk of primary graft dysfunction and mortality post lung transplantation: A proposed conceptual framework. Clin Transplant 2021;35:e14480. [Crossref] [PubMed]
- Nelems JM, Duffin J, Glynn FX, et al. Extracorporeal membrane oxygenator support for human lung transplantation. J Thorac Cardiovasc Surg 1978;76:28-32. [Crossref] [PubMed]
- Leard LE, Holm AM, Valapour M, et al. Consensus document for the selection of lung transplant candidates: An update from the International Society for Heart and Lung Transplantation. J Heart Lung Transplant 2021;40:1349-79. [Crossref] [PubMed]
- Dell'Amore A, Campisi A, Congiu S, et al. Extracorporeal life support during and after bilateral sequential lung transplantation in patients with pulmonary artery hypertension. Artif Organs 2020;44:628-37. [Crossref] [PubMed]
- Fischer S, Bohn D, Rycus P, et al. Extracorporeal membrane oxygenation for primary graft dysfunction after lung transplantation: analysis of the Extracorporeal Life Support Organization (ELSO) registry. J Heart Lung Transplant 2007;26:472-7. [Crossref] [PubMed]
- Bermudez CA, Adusumilli PS, McCurry KR, et al. Extracorporeal membrane oxygenation for primary graft dysfunction after lung transplantation: long-term survival. Ann Thorac Surg 2009;87:854-60. [Crossref] [PubMed]
- Weingarten N, Schraufnagel D, Plitt G, et al. Comparison of mechanical cardiopulmonary support strategies during lung transplantation. Expert Rev Med Devices 2020;17:1075-93. [Crossref] [PubMed]
- Ohsumi A, Date H. Perioperative circulatory support for lung transplantation. Gen Thorac Cardiovasc Surg 2021;69:631-7. [Crossref] [PubMed]
- Wigfield CH, Lindsey JD, Steffens TG, et al. Early institution of extracorporeal membrane oxygenation for primary graft dysfunction after lung transplantation improves outcome. J Heart Lung Transplant 2007;26:331-8. [Crossref] [PubMed]
- Bellier J, Lhommet P, Bonnette P, et al. Extracorporeal membrane oxygenation for grade 3 primary graft dysfunction after lung transplantation: Long-term outcomes. Clin Transplant 2019;33:e13480. [Crossref] [PubMed]
- Hartwig MG, Walczak R, Lin SS, et al. Improved survival but marginal allograft function in patients treated with extracorporeal membrane oxygenation after lung transplantation. Ann Thorac Surg 2012;93:366-71. [Crossref] [PubMed]
- Martin AK, Jayaraman AL, Nabzdyk CG, et al. Extracorporeal Membrane Oxygenation in Lung Transplantation: Analysis of Techniques and Outcomes. J Cardiothorac Vasc Anesth 2021;35:644-61. [Crossref] [PubMed]
- Hoetzenecker K, Donahoe L, Yeung JC, et al. Extracorporeal life support as a bridge to lung transplantation-experience of a high-volume transplant center. J Thorac Cardiovasc Surg 2018;155:1316-1328.e1. [Crossref] [PubMed]
- Ius F, Tudorache I, Warnecke G. Extracorporeal support, during and after lung transplantation: the history of an idea. J Thorac Dis 2018;10:5131-48. [Crossref] [PubMed]
- Shigemura N, Orhan Y, Bhama JK, et al. Delayed chest closure after lung transplantation: techniques, outcomes, and strategies. J Heart Lung Transplant 2014;33:741-8. [Crossref] [PubMed]
- Force SD, Miller DL, Pelaez A, et al. Outcomes of delayed chest closure after bilateral lung transplantation. Ann Thorac Surg 2006;81:2020-4; discussion 2024-5. [Crossref] [PubMed]
- Tsou S, Chen J, Feng C, et al. Delayed Chest Closure Following Bilateral Lung Transplantation: Risk Factors and Outcomes. J Heart Lung Transplant 2021;40:S162-S163. [Crossref]
- Zimpfer D, Heinisch B, Czerny M, et al. Late vascular complications after extracorporeal membrane oxygenation support. Ann Thorac Surg 2006;81:892-5. [Crossref] [PubMed]
- Iske J, Hinze CA, Salman J, et al. The potential of ex vivo lung perfusion on improving organ quality and ameliorating ischemia reperfusion injury. Am J Transplant 2021;21:3831-9. [Crossref] [PubMed]
- Loor G, Huddleston S, Hartwig M, et al. Effect of mode of intraoperative support on primary graft dysfunction after lung transplant. J Thorac Cardiovasc Surg 2022;164:1351-1361.e4. [Crossref] [PubMed]
- Machuca TN, Collaud S, Mercier O, et al. Outcomes of intraoperative extracorporeal membrane oxygenation versus cardiopulmonary bypass for lung transplantation. J Thorac Cardiovasc Surg 2015;149:1152-7. [Crossref] [PubMed]
- Bermudez CA, Shiose A, Esper SA, et al. Outcomes of intraoperative venoarterial extracorporeal membrane oxygenation versus cardiopulmonary bypass during lung transplantation. Ann Thorac Surg 2014;98:1936-42; discussion 1942-3. [Crossref] [PubMed]
- Ius F, Kuehn C, Tudorache I, et al. Lung transplantation on cardiopulmonary support: venoarterial extracorporeal membrane oxygenation outperformed cardiopulmonary bypass. J Thorac Cardiovasc Surg 2012;144:1510-6. [Crossref] [PubMed]
- Nagendran M, Maruthappu M, Sugand K. Should double lung transplant be performed with or without cardiopulmonary bypass? Interact Cardiovasc Thorac Surg 2011;12:799-804. [Crossref] [PubMed]
- Bhabra MS, Hopkinson DN, Shaw TE, et al. Controlled reperfusion protects lung grafts during a transient early increase in permeability. Ann Thorac Surg 1998;65:187-92. [Crossref] [PubMed]
- Hopkinson DN, Bhabra MS, Odom NJ, et al. Controlled pressure reperfusion of rat pulmonary grafts yields improved function after twenty-four-hours' cold storage in University of Wisconsin solution. J Heart Lung Transplant 1996;15:283-90. [PubMed]
- Bhabra MS, Hopkinson DN, Shaw TE, et al. Critical importance of the first 10 minutes of lung graft reperfusion after hypothermic storage. Ann Thorac Surg 1996;61:1631-5. [Crossref] [PubMed]
- Guth S, Prüfer D, Kramm T, et al. Length of pressure-controlled reperfusion is critical for reducing ischaemia-reperfusion injury in an isolated rabbit lung model. J Cardiothorac Surg 2007;2:54. [Crossref] [PubMed]
- Hoetzenecker K, Benazzo A, Stork T, et al. Bilateral lung transplantation on intraoperative extracorporeal membrane oxygenator: An observational study. J Thorac Cardiovasc Surg 2020;160:320-327.e1. [Crossref] [PubMed]
- Christie JD, Edwards LB, Aurora P, et al. The Registry of the International Society for Heart and Lung Transplantation: Twenty-sixth Official Adult Lung and Heart-Lung Transplantation Report-2009. J Heart Lung Transplant 2009;28:1031-49. [Crossref] [PubMed]
- Tudorache I, Sommer W, Kühn C, et al. Lung transplantation for severe pulmonary hypertension--awake extracorporeal membrane oxygenation for postoperative left ventricular remodelling. Transplantation 2015;99:451-8. [Crossref] [PubMed]
- Bos S, Vos R, Van Raemdonck DE, et al. Survival in adult lung transplantation: where are we in 2020? Curr Opin Organ Transplant 2020;25:268-73. [Crossref] [PubMed]
- Christie JD, Bellamy S, Ware LB, et al. Construct validity of the definition of primary graft dysfunction after lung transplantation. J Heart Lung Transplant 2010;29:1231-9. [Crossref] [PubMed]
- Whitson BA, Nath DS, Johnson AC, et al. Risk factors for primary graft dysfunction after lung transplantation. J Thorac Cardiovasc Surg 2006;131:73-80. [Crossref] [PubMed]
- Christie JD, Kotloff RM, Ahya VN, et al. The effect of primary graft dysfunction on survival after lung transplantation. Am J Respir Crit Care Med 2005;171:1312-6. [Crossref] [PubMed]
- Kreisel D, Krupnick AS, Puri V, et al. Short- and long-term outcomes of 1000 adult lung transplant recipients at a single center. J Thorac Cardiovasc Surg 2011;141:215-22. [Crossref] [PubMed]
- Boffini M, Simonato E, Ricci D, et al. Extracorporeal membrane oxygenation after lung transplantation: risk factors and outcomes analysis. Ann Cardiothorac Surg 2019;8:54-61. [Crossref] [PubMed]
- Meyers BF, Sundt TM 3rd, Henry S, et al. Selective use of extracorporeal membrane oxygenation is warranted after lung transplantation. J Thorac Cardiovasc Surg 2000;120:20-6. [Crossref] [PubMed]
- Harano T, Ryan JP, Morrell MR, et al. Extracorporeal Membrane Oxygenation for Primary Graft Dysfunction After Lung Transplantation. ASAIO J 2021;67:1071-8. [Crossref] [PubMed]