Development of a prediction model for exercise tolerance decline in the exercise assessment of patients with acute myocardial infarction undergoing percutaneous coronary intervention revascularization in the acute phase
Highlight box
Key findings
• It is safe to perform the cardiopulmonary exercise test (CPET) early after percutaneous coronary intervention (PCI) in patients with acute myocardial infarction (AMI). The decline of early exercise capacity in patients with AMI after PCI is related to age, diastolic dysfunction, renal function, and blood glucose control, which may lead to poor clinical prognosis.
What is known and what is new?
• Dwindling exercise capacity is recognized as a significant harbinger of unfavorable outcomes in individuals with cardiovascular conditions and even among the broader populace.
• In this research, we formulated a model to anticipate the reduction in exercise tolerance in patients with AMI post-acute phase PCI, drawing on comprehensive clinical data.
What is the implication, and what should change now?
• Patients with AMI after PCI can undergo CPET at an early stage. The results of CPET can help predict the prognosis of patients with AMI.
Introduction
Although reperfusion therapy and drug therapy strategies have advanced considerably (1), acute myocardial infarction (AMI) is still one of the main causes of death in patients with coronary heart disease, which seriously threatens the lives and health of patients (2). The 2021 China Cardiovascular Health and Disease Report shows that the morbidity and mortality of cardiovascular diseases in China are still on the rise, with the mortality of cardiovascular diseases ranking first, higher than that of tumors and other diseases, with the mortality of AMI rapidly increasing. With the development of reperfusion therapy, percutaneous coronary intervention (PCI) has become an important method for treating AMI and is one of the most effective measures for reducing the mortality of patients with AMI. It’s widely recognized that exercise incapacity serves as a significant forecaster of adverse outcomes and impairment, not only in patients grappling with cardiovascular disease and chronic heart failure, but also within the general populace (3-5). The cardiopulmonary exercise test (CPET) measures peak oxygen consumption (VO2peak), which is widely used as the gold standard for measuring exercise capacity (6,7). Additionally, the CPET has become the most important and valuable noninvasive diagnostic test for clinical evaluation of cardiac rehabilitation in patients with AMI and is also widely used to evaluate the efficacy of PCI. The CPET is also an important parameter for evaluating the quality of life of patients, which is helpful to judging the degree of myocardial ischemia and is helpful in guiding clinical medication, rehabilitation training, and subsequent related treatment (8). With the continuous development of medicine, the average hospital stay of those with AMI has been shortened. Generally, patients with AMI are discharged fewer than 7 days after PCI. Weber and Janicki (9) proposed a cardiac function evaluation method based on VO2peak and VO2 at Anaerobic threshold (AT). According to the degree of decrease of VO2peak and VO2 at AT, the cardiac function was divided into 4 grades: A, B, C, and D. The grades of VO2peak <16 mL/kg/min were C or D, while the grades of VO2peak ≥16 mL/kg/min were A or B. A growing body of research indicates that exercise training offers encouraging impacts on the restoration of the heart after infarction. These beneficial effects are apparent in aspects such as the reduction of infarct size and cardiac fibrosis, the dampening of myocardial apoptosis, enhancements in ventricular remodeling, and the mitigation of inflammation (10-14). Applying the CPET before discharge is helpful for formulating exercise prescriptions for phase II cardiac rehabilitation. However, data concerning the ability of CPET to assess the exercise capacity of patients with AMI undergoing PCI are still scarce. Therefore, this study evaluated the safety of the CPET and assessed the predictors and clinical influence of exercise capacity measured by CPET in patients with AMI within 1 week after PCI. We present this article in accordance with the TRIPOD reporting checklist (available at https://jtd.amegroups.com/article/view/10.21037/jtd-23-554/rc).
Methods
Research participants
This was a single-center, retrospective study. From January 2017 to April 2020, 275 patients with a first MI who were admitted to First Hospital of Quanzhou Affiliated to Fujian Medical University for PCI treatment in the acute phase were selected. All patients completed CPET within 1 week after PCI. There were 253 males (92%) and 22 females (8%), with mean age of 58.20±10.51 years. The patients without contraindication were routinely treated with dual-antiplatelet drugs, statins, angiotensin-converting enzyme inhibitors/angiotensin receptor antagonists, and β-receptor blockers and other secondary preventive drugs. All patients have a Killip classification of I or II. The inclusion criteria were the following: (I) age ≥18 years; (II) first MI with definite diagnosis; (III) successful PCI treatment, culprit vessel residual stenosis less than 20% immediately after treatment, and blood flow grade of thrombolysis in MI (TIMI) grade III after operation; (IV) completion of CPET within 1 week after PCI treatment; and (V) signed informed consent for PCI form and agreed to undergo CEPT examination and data collection.
The exclusion criteria were the following: (I) repeated chest distress and pain after PCI accompanied by cardiogenic shock, severe arrhythmia, or other complications; (II) active endocarditis, myocarditis, pericarditis, severe aortic stenosis, etc.; (III) complicated with other system diseases, such as malignant tumor, severe anemia, acute infection, severe liver and kidney dysfunction, moderate to severe chronic lung disease, pulmonary embolism, and interstitial lung disease; (IV) use of cardiac assist devices such as IABP, left ventricular assist devices during hospitalization; (V) refusal to accept CPET or the presence of diseases limiting movement, such as severe physical activity disorder and mental disorder. The study was conducted in accordance with the Declaration of Helsinki (as revised in 2013). The study was approved by ethics board of First Hospital of Quanzhou Affiliated to Fujian Medical University (No. K052) and individual consent for this retrospective analysis was waived.
Research methods
Data collection
Demographics, medical history, medication history, laboratory data, echocardiographic parameters, coronary angiography data and CPET parameters were collected from medical records. The following major adverse cardiovascular events (MACEs) within 24 months after telephone follow-up were recorded: all-cause death, secondary MI, secondary revascularization, and rehospitalization.
Coronary angiography and interventional therapy
Coronary angiography was performed on a Digital Subtraction Angiography system (GE Healthcare). The angiographic results were interpreted by at least 2 experienced interventional cardiologists. The vascular diameter method was used to determine the severity of the vascular disease through visual inspection, with the relatively normal vascular segments at the proximal and distal ends of the disease as the reference. The percentage of the loss of the vascular diameter was expressed as the percentage of the right coronary artery, the left main trunk, and the left anterior descending branch. The stenosis degree of the lumen diameter of the left circumflex artery and its main branches was evaluated, and the diameter of the vessels involved in the evaluation was at least 1.5 mm. Coronary artery disease was defined as the loss of vessel diameter ≥50%. According to the number of coronary artery lesions and the degree of blood circulation reconstruction, the patients were divided into the following groups: (I) the single-vessel lesion group, in whom major coronary arteries and/or only 1 major branch with a diameter of more than 2 mm had stenosis ≥50%; (II) complete blood circulation group, in whom, after PCI, the main coronary arteries (left main artery, anterior descending artery, circumflex artery, and right coronary artery) and their important branches (diagonal branch and obtuse marginal branch) in patients with multi vessel disease did not have more than 70% residual stenosis; and (III) the incomplete revascularization group, in whom stenosis of any of the above vessels and their important branches was ≥70% in patients with multivessel disease after PCI.
Cardiopulmonary exercise experiment
Vyntus CPX equipment (Vyaire Medical GmbH, Leibnizstrasse 797204 Hoechberg, Germany) was used, which was strictly graded and calibrated every day for clinical patient examination. All patients were given an exercise test with a Ramp protocol with cycle ergometer. The protocol for CPET evaluation was as follows: the test started with a 3-min rest, followed by a 3-min warm-up at 0 W, and continued cycling at an increased workload of 10–30 W per minute until volitional fatigue occurs. Encourage driving at a constant speed of 55–65 r/min while cycling. The total duration of the cycle test is usually 10–15 min (15). The patient’s blood pressure, electrocardiogram (ECG), gas exchange and other information are continuously collected and recorded during the testing. The entire process was accompanied by a professional cardiologist. The test was terminated when the heart rate reached 75–85% of the age-predicted maximal heart rate or when the respiratory exchange ratio (RER) >1.05. If the target heart rate was not reached or the RER ≤1.05, the test was terminated if any of the following occurred: (I) the patient had chest tightness, chest pain, dyspnea, dizziness, lower-limb pain, or fatigue preventing further exercise; (II) the ECG showed that adjacent leads had a horizontal or downsloping ST-segment depression of ≥0.20 mV for more than 1 min; ST-segment arch upward elevation of ≥0.1 mV; (III) drop in blood pressure during exercise of more than 10 mmHg; (IV) an abnormal elevation of blood pressure during exercise, with systolic blood pressure >200 mmHg or diastolic blood pressure >110 mmHg; (V) ventricular tachycardia, supraventricular tachycardia, frequent ventricular premature beat, II–III atrioventricular block, or significant sinus bradycardia. Reduced exercise capacity was defined as VO2peak <16 mL/kg/min.
Statistical analysis
Univariate and multivariate logistic analysis were assessed with Stata 15 (StataCorp). Nomogram model and Calibration curve were assessed with R software (version 3.6.3). Continuous variables were presented as mean ± standard deviation or median [interquartile range (IQR)]. The categorical variables were expressed in numbers and percentages. Evaluation of differences between groups was achieved using Student’s t-test for continuous variables with normal distribution, while the Mann-Whitney U test was employed for variables not conforming to a normal distribution. For variables of a categorical nature, either the chi-squared test or Fisher’s exact test was utilized. We conducted univariate and multivariate logistic regression analysis to determine the predictive factors for reduced exercise tolerance. Significance of association between predictive factors and reduced exercise tolerance was assessed with univariate logistic regression. All preoperatively available variables (demographics, medical history, medication history, laboratory data, echocardiographic parameters, coronary angiography data and CPET parameters) significant on univariate analysis with P<0.05 were including the multivariate logistic regression analysis.
Based on the multivariate logistic regression model, we then constructed a nomogram model to predict the exercise ability. Predictors deemed significant (P<0.05) through multivariate logistic regression were incorporated to establish the nomogram aimed at predicting the onset of diminished exercise tolerance. Lines were extended upward to ascertain the respective points garnered from the nomogram. The aggregation of these points was identified on the ‘Total Points’ axis; a line was then extended downward to the lower scales to determine the likelihood of low exercise tolerance. The visual predictive model subsequently underwent external validation. The Hosmer-Lemeshow test was leveraged to gauge the model’s goodness of fit. The model’s predictive precision and adherence were assessed via the receiver operating characteristic (ROC) curve, the area under the ROC curve (AUC), the concordance index (C-index), and the calibration curve. The calibration was assessed by bootstrapping with 1,000 resamples.
Results
CPET safety
The median time of CPET was 5 (IQR, 3–6) days after PCI. Most of the patients stopped exercise because they felt tired, while only 5.7% and 1.1% of the patients stopped the test because ECG showed changes in myocardial ischemia or decreased blood pressure, respectively. The ECG and blood pressure of these patients recovered to the pretest level half an hour after the exercise was stopped. None of the patients in this study experience serious adverse events, including severe arrhythmia, syncope, or sudden death (Figure 1).
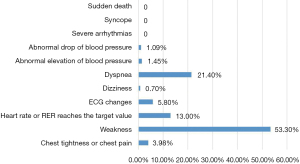
General information of patients
Table 1 summarizes the characteristics of the 275 patients. The study population mainly included men (92%), with an average age of 58.20±10.52 years. The prevalence of hypertension was 49.09% (135/275), and the prevalence of diabetes was 30.90% (85/275). Moreover, 54.90% (151/275) of the patients had ST-segment elevation myocardial infarction (STEMI), 26.2% had anterior wall MI, and 44.73% (123/275) of the patients still had incomplete revascularization. In echocardiography, the LVEF was 54.22%±9.51%, and the E/e' was 10.95±3.44. Most patients used β-receptor blockers (207/275; 75.27%). Among 275 patients, 90 (32.72%) had decreased exercise ability (VO2peak <16 mL/kg/min). As shown in Table 1, compared with patients with VO2peak ≥16 mL/kg/min, patients with VO2peak <16 mL/kg/min were more likely to be older, female, have hypertension, a lower estimated glomerular filtration rate (eGFR), a lower hemoglobin content, a higher glycosylated hemoglobin, a higher BNP. Additionally, the coronary arteries were more likely to be incompletely revascularized, and insulin was more likely to be used in the patients with VO2peak <16 mL/kg/min. There was no significant difference in the type of MI, number of stents implanted, or the use of β-receptor blockers. Regarding the echocardiographic results, there was no significant difference LVEF between the 2 groups. Meanwhile, patients with VO2peak <16 mL/kg/min had higher E/e'.
Table 1
Characteristics | ALL (n=275) | Peak VO2 <16 mL/kg/min (n=90) | Peak VO2 ≥16 mL/kg/min (n=185) | P |
---|---|---|---|---|
Age, years, mean ± SD | 58.20±10.52 | 63.20±11.05 | 55.77±9.35 | <0.001 |
Male, n (%) | 253 (92.00) | 77 (85.56) | 176 (95.14) | 0.006 |
Body mass index, kg/m2, mean ± SD | 24.88±3.07 | 25.37±3.41 | 24.64±2.87 | 0.066 |
Cardiovascular risk factors, n (%) | ||||
Hypertension | 135 (49.09) | 52 (57.78) | 83 (44.86) | 0.044 |
Diabetic mellitus | 85 (30.90) | 34 (37.78) | 51 (27.57) | 0.086 |
Smoker | 168 (61.09) | 52 (57.78) | 116 (62.70) | 0.432 |
Type of myocardial infarction, n (%) | ||||
STEMI | 151 (54.90) | 50 (55.56) | 101 (54.59) | 0.881 |
Anterior wall MI | 72 (26.18) | 23 (25.56) | 49 (26.49) | 0.869 |
TIMI level 0 | 109 (39.64) | 39 (43.33) | 70 (37.84) | 0.382 |
Incomplete revascularization | 123 (44.73) | 51 (56.67) | 72 (38.92) | 0.005 |
Number of implanted stents, n (%) | 0.120 | |||
1 | 191 (69.45) | 56 (62.22) | 135 (72.97) | |
2 | 66 (24.00) | 25 (27.78) | 41 (22.16) | |
≥3 | 18 (6.55) | 9 (10.00) | 9 (4.86) | |
Laboratory data, mean ± SD | ||||
Hemoglobin, g/dL | 142.66±16.42 | 138.38±17.05 | 144.74±15.73 | 0.002 |
TC, mg/dL | 5.23±1.29 | 5.10±1.33 | 5.29±1.27 | 0.271 |
TG, mg/dL | 1.92±1.43 | 1.86±1.15 | 1.95±1.55 | 0.616 |
LDL-C, mg/dL | 3.41±1.12 | 3.33±1.20 | 3.45±1.08 | 0.393 |
HA1c, % | 6.51±1.52 | 6.83±1.85 | 6.36±1.31 | 0.015 |
eGFR, mL/min/1.73 m2 | 93.80±21.08 | 83.96±21.21 | 98.58±19.33 | <0.001 |
BNP, pg/mL, median [IQR] | 80 [28–183] | 114.5 [40–337] | 72 [22–152.5] | <0.001 |
Medication, n (%) | ||||
Antiplatelet therapy | 275 (100.00) | 90 (100.00) | 185 (100.00) | – |
Statin | 275 (100.00) | 90 (100.00) | 185 (100.00) | – |
ACEI/ARB/ARNI | 180 (65.45) | 55 (61.11) | 125 (67.57) | 0.291 |
β-blocker | 207 (75.27) | 62 (68.89) | 145 (78.38) | 0.087 |
Oral diabetes drug | 71 (25.82) | 28 (31.11) | 43 (23.24) | 0.162 |
Insulin | 18 (6.55) | 10 (11.11) | 8 (4.32) | 0.033 |
Diuretic | 80 (29.09) | 31 (34.44) | 49 (26.49) | 0.173 |
Echocardiographic parameters, mean ± SD | ||||
LVEF, % | 54.22±9.51 | 52.84±10.72 | 54.89±8.82 | 0.095 |
LVDd, mm | 49.72±4.75 | 50.04±4.97 | 49.57±4.64 | 0.435 |
E/e' | 10.95±3.44 | 12.33±4.19 | 10.27±2.79 | <0.001 |
STEMI, ST-segment elevation myocardial infarction; MI, myocardial infarction; TIMI, thrombolysis in myocardial infarction; TC, total cholesterol; TG, triglyceride; LDL-C, low-density lipoprotein cholesterol; HA1c, glycosylated hemoglobin; eGFR, estimated glomerular filtration rate; BNP, brain natriuretic peptide; IQR, interquartile range; ACEI, angiotensin-converting enzyme inhibitor; ARB, angiotensin receptor blocker; ARNI, angiotensin receptor enkephalinase inhibitor; LVEF, left ventricular ejection fraction; LVDd, left ventricular diastolic dimension; E/e', the ratio of early diastolic transmitral flow velocity to early diastolic tissue velocity.
Development of the prediction model for CPET-related exercise capacity in patients with AMI after PCI in the early stage
Multivariate logic analysis showed that E/e' [odds ratio (OR) =1.10; 95% CI: 1.01–1.22; P=0.037], age (OR =1.05; 95% CI: 1.04–1.10; P=0.002), glycosylated hemoglobin (OR =1.26; 95% CI: 1.04–1.52; P=0.021), and eGFR (OR =0.98, 95% CI: 0.96–0.99; P=0.016) were independent predictors of early exercise capacity reduction in patients with AMI undergoing PCI (VO2peak <16 mL/kg/min) (Table 2).
Table 2
Variable | Univariate | Multivariate | |||||
---|---|---|---|---|---|---|---|
OR | 95% CI | P value | OR | 95% CI | P value | ||
Age | 1.07 | 1.04–1.11 | <0.001 | 1.05 | 1.04–1.10 | 0.002 | |
Male | 0.30 | 0.12–0.74 | 0.009 | 0.44 | 0.15–1.30 | 0.138 | |
Body mass index | 1.08 | 0.99–1.17 | 0.068 | ||||
Hypertension | 1.68 | 1.01–2.80 | 0.045 | 1.50 | 0.82–2.76 | 0.188 | |
Smoker | 0.81 | 0.49–1.36 | 0.432 | ||||
Diabetes | 1.60 | 0.93–2.72 | 0.087 | ||||
HA1c | 1.22 | 1.03–1.43 | 0.017 | 1.26 | 1.04–1.52 | 0.021 | |
STEMI | 1.04 | 0.63–1.73 | 0.881 | ||||
Hemoglobin | 0.98 | 0.96–0.99 | 0.003 | 0.99 | 0.98–1.02 | 0.928 | |
BNP | 1.001 | 1.00–1.003 | 0.002 | 1.00 | 0.99–1.00 | 0.452 | |
eGFR | 0.97 | 0.95–0.98 | <0.001 | 0.98 | 0.96–0.99 | 0.016 | |
β-blocker | 0.61 | 0.35–1.08 | 0.088 | ||||
LVEF | 0.104 | 0.007–1.494 | 0.096 | ||||
E/e' | 1.20 | 1.11–1.31 | <0.001 | 1.10 | 1.01–1.22 | 0.037 | |
TIMI flow level 0 | 1.20 | 1.11–1.31 | <0.001 | 1.68 | 0.90–3.12 | 0.098 | |
Number of implanted stents | 1.52 | 1.01–2.28 | 0.043 | 1.41 | 0.89–2.26 | 0.149 | |
Incomplete revascularization | 2.05 | 1.23–3.42 | 0.006 | 1.42 | 0.77–2.62 | 0.261 |
HA1c, glycosylated hemoglobin; STEMI, ST-segment elevation myocardial infarction; TIMI, thrombolysis in myocardial infarction; eGFR, estimated glomerular filtration rate; BNP, brain natriuretic peptide; LVEF, left ventricular ejection fraction; E/e', the ratio of early diastolic transmitral flow velocity to early diastolic tissue velocity.
A nomogram model was developed to predict the early exercise capacity of AMI patients based on the correlation coefficients of clinical indicators predicted in the logistic regression model (Figure 2). The consistency index (C-index) of the nomogram model for predicting exercise capacity was 0.771, and the area under the ROC curve of the subjects was 0.771 (95% CI: 0.710–0.832), sensitivity was 61%, specificity was 84%. The bootstrap internal verification method is used to verify the prediction effect of the model based on the modeling data validation. The C-index was determined to be 0.771, suggesting a relatively precise predictive capacity of the nomogram. The results of the Hosmer-Lemeshow test show that, χ2=5.124, P=0.744, indicating that the model has no statistically significant difference between predicted values and actual observed values. The calibration curve of the nomogram demonstrates that the predicted values closely align with the actual measurements, signifying robust calibration performance of the nomogram model implemented in this study (Figure 3).
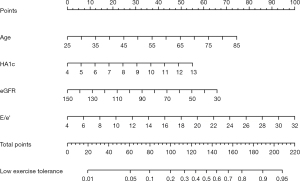
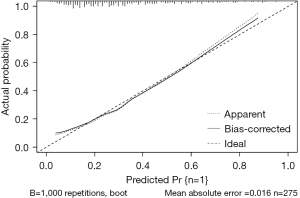
Follow-up results
The 275 patients with AMI undergoing revascularization and CPET within 1 week after operation were followed up by telephone. A total of 242 patients were followed up by telephone, and 33 patients were lost to follow-up. The follow-up time was 24 months. The incidence of all-cause death, reinfarction, revascularization, and rehospitalization in patients with low exercise tolerance was significantly higher than that in patients with high exercise tolerance, with all-cause mortality and remyocardial infarction being statistically different (P=0.009 and P=0.043) (Table 3).
Table 3
Peak VO2 | Hospitalization, n (%) | Revascularization, n (%) | Reinfarction, n (%) | Death, n (%) |
---|---|---|---|---|
<16 mL/kg/min (n=75) | 21 (28.0) | 16 (21.3) | 11 (14.7) | 3 (4.0) |
≥16 mL/kg/min (n=167) | 29 (17.4) | 20 (12.0) | 11 (6.6) | 0 (0.0) |
P value | 0.059 | 0.059 | 0.043 | 0.009 |
Discussion
The main findings of this study are as follows: (I) it is safe to perform CPET soon after PCI for those with AMI. All patients completed the CPET, and no patients had serious arrhythmia, syncope, or sudden death during the detection process. Reduced exercise ability (VO2peak <16 mL/kg/min) was seen in 32.7% of patients with AMI. (II) Patients with decreased exercise tolerance tended to be older, women, and more likely to have hypertension, lower renal function, lower hemoglobin content, higher glycosylated hemoglobin, and higher BNP. (III) Regarding echocardiography, patients with decreased exercise tolerance had higher E/e'. (IV) In terms of coronary arteries, patients with decreased exercise tolerance were more likely to have vessels with incomplete revascularization. (V) Concerning drug intake, patients with decreased exercise tolerance were more likely to use insulin. (VI) E/e', glycosylated hemoglobin, age, and renal function were independent predictors of decreased exercise capacity (VO2peak <16 mL/kg/min). (VII) Low exercise tolerance was a predictor of poor prognosis.
In 2016, the American Heart Association regards aerobic exercise capacity as the fifth vital sign of the human body besides respiration, body temperature, heart rate, and blood pressure. Exercise tolerance as an important indicator in the field of cardiac rehabilitation, which can evaluate using VO2peak obtained from CPET. Research shows that regardless of the revascularization treatment received, exercise tolerance is a strong predictor of death and reinfarction of patients with AMI (16). The low level of early exercise tolerance is related to the increased risk of all-cause death, especially the risk of death caused by cardiovascular disease (17). VO2peak is the product of cardiac output and peripheral tissue oxygen consumption. There are many factors affecting VO2peak, including age, gender, body mass index, activity level, exercise type, etc. VO2peak can be reduced if it affects the oxygen carrying capacity (hemoglobin, oxygen partial pressure, etc.) in the blood system, the status of cardiac function and circulation (heart rate, stroke output, etc.), and the status of pulmonary function. In this study, patients with decreased exercise ability tended to be older, women, and more likely to have hypertension, lower renal function, lower hemoglobin content, and higher peak BNP. These findings in patients with AMI are consistent with those of previous reports (18-24). In echocardiography, E/e' is usually considered as a marker of diastolic dysfunction (25). In our study, patients with decreased exercise ability had higher E/e', with E/e' being an independent predictor of decreased exercise ability. Previous studies (26,27) have shown that diastolic rather than systolic function assessed by echocardiography is associated with a lower VO2peak value. Our results support these conclusions.
This study developed a model for predicting reduced exercise capacity of patients with AMI undergoing PCI. Patients with higher E/e', older age, higher glycosylated hemoglobin level, and lower eGFR had a reduced exercise capacity.
Nomogram is a graphical calculation tool that visualizes the results of logistic regression analysis, which is beneficial for healthcare professionals to more intuitively analyze the risk weight of a certain indicator occurrence. In this study, the nomogram prediction model was established based on the results of multivariate logistic regression analysis, and the model was verified to have good prediction ability through the correction curve and receiver operating characteristic. An example of the nomogram’s model application is given as follows. After AMI and PCI, a 65-year-old man with diabetes had glycosylated hemoglobin 12%, eGFR 60 mL/min/1.73 m2, and E/e' 18. These measures were summarized according to the nomogram as follows: 50 points (age) + 50 points (HA1c) + 50 points (echocardiographic E/e') + 50 points (eGFR) =200 points. The total score of nomogram model indicates a probability of declining exercise capacity of approximately 92%. The nomogram prediction model performs well in predicting reduced exercise capacity in the exercise assessment of patients with AMI undergoing PCI in the acute phase.
The 275 patients with AMI receiving PCI were followed up to observe the major cardiovascular events within 24 months. The results showed that the incidence of readmission, revascularization, reinfarction, and all-cause death in the patients with normal exercise tolerance group was lower than that in patients with decreased exercise tolerance. Among these outcomes, all-cause death and reinfarction were statistically different, which was consistent with the conclusions of previous studies (16,17). Therefore, our predictive model can indirectly forecast the prognosis of AMI patients. However, we define a decrease in exercise tolerance based on whether it falls into Category C or D according to the Weber cardiovascular functional classification. Our study did not provide a precise peak oxygen uptake turning point that could predict major adverse cardiovascular events (MACE) with considerable accuracy. This is a limitation of our current research, and we hope subsequent studies will yield more precise answers.
Some other limitations of this study should be noted. First, as a retrospective study, the sample size of this study and number of events were small. The sample size needs to be further expanded to assess the impact of VO2peak on prognosis. Second, the patients who could not complete the CPET were not included in this study, meaning that patients with severe conditions (such as shock and severe heart failure requiring mechanical support) could be included, which might have affected the results.
Conclusions
Through the development and validation of a nomogram model, this study has presented a practical tool for predicting the decline in exercise tolerance in patients with AMI undergoing PCI. The model, which incorporates factors such as E/e', age, glycosylated hemoglobin, and eGFR, provides an evidence-based method to identify those patients at an increased risk of early exercise capacity reduction. This is particularly significant as lower exercise tolerance has been associated with increased incidences of all-cause mortality and reinfarction, emphasizing the need for early identification and management of these high-risk patients. Future research is encouraged to further refine and externally validate this model, with the ultimate aim of improving patient outcomes in this vulnerable population.
Acknowledgments
Funding: None.
Footnote
Reporting Checklist: The authors have completed the TRIPOD reporting checklist. Available at https://jtd.amegroups.com/article/view/10.21037/jtd-23-554/rc
Data Sharing Statement: Available at https://jtd.amegroups.com/article/view/10.21037/jtd-23-554/dss
Peer Review File: Available at https://jtd.amegroups.com/article/view/10.21037/jtd-23-554/prf
Conflicts of Interest: All authors have completed the ICMJE uniform disclosure form (available at https://jtd.amegroups.com/article/view/10.21037/jtd-23-554/coif). The authors have no conflicts of interest to declare.
Ethical Statement: The authors are accountable for all aspects of the work in ensuring that questions related to the accuracy or integrity of any part of the work are appropriately investigated and resolved. The study was conducted in accordance with the Declaration of Helsinki (as revised in 2013). The study was approved by ethics board of First Hospital of Quanzhou Affiliated to Fujian Medical University (No. K052) and individual consent for this retrospective analysis was waived.
Open Access Statement: This is an Open Access article distributed in accordance with the Creative Commons Attribution-NonCommercial-NoDerivs 4.0 International License (CC BY-NC-ND 4.0), which permits the non-commercial replication and distribution of the article with the strict proviso that no changes or edits are made and the original work is properly cited (including links to both the formal publication through the relevant DOI and the license). See: https://creativecommons.org/licenses/by-nc-nd/4.0/.
References
- Reed GW, Rossi JE, Cannon CP. Acute myocardial infarction. Lancet 2017;389:197-210. [Crossref] [PubMed]
- Johansen MC, Ye W, Gross A, et al. Association Between Acute Myocardial Infarction and Cognition. JAMA Neurol 2023;80:723-31. [Crossref] [PubMed]
- Sarullo FM, Fazio G, Brusca I, et al. Cardiopulmonary Exercise Testing in Patients with Chronic Heart Failure: Prognostic Comparison from Peak VO2 and VE/VCO2 Slope. Open Cardiovasc Med J 2010;4:127-34. [Crossref] [PubMed]
- Vanhees L, Fagard R, Thijs L, et al. Prognostic significance of peak exercise capacity in patients with coronary artery disease. J Am Coll Cardiol 1994;23:358-63. [Crossref] [PubMed]
- Chaumont M, Forton K, Gillet A, et al. How Does the Method Used to Measure the VE/VCO(2) Slope Affect Its Value? A Cross-Sectional and Retrospective Cohort Study. Healthcare (Basel) 2023;11:1292. [Crossref] [PubMed]
- Kitzman DW, Groban L. Exercise intolerance. Cardiol Clin 2011;29:461-77. [Crossref] [PubMed]
- Nemati S, Yavari T, Tafti F, et al. An Index for Evaluating Exercise Capacity Improvement After Cardiac Rehabilitation in Patients After Myocardial Infarction. J Cardiovasc Nurs 2023; Epub ahead of print. [Crossref]
- Fletcher GF, Balady GJ, Amsterdam EA, et al. Exercise standards for testing and training: a statement for healthcare professionals from the American Heart Association. Circulation 2001;104:1694-740. [Crossref] [PubMed]
- Weber KT, Janicki JS. Cardiopulmonary exercise testing for evaluation of chronic cardiac failure. Am J Cardiol 1985;55:22A-31A. [Crossref] [PubMed]
- Koizumi T, Miyazaki A, Komiyama N, et al. Improvement of left ventricular dysfunction during exercise by walking in patients with successful percutaneous coronary intervention for acute myocardial infarction. Circ J 2003;67:233-7. [Crossref] [PubMed]
- Wisløff U, Loennechen JP, Currie S, et al. Aerobic exercise reduces cardiomyocyte hypertrophy and increases contractility, Ca2+ sensitivity and SERCA-2 in rat after myocardial infarction. Cardiovasc Res 2002;54:162-74. [Crossref] [PubMed]
- Puhl SL, Müller A, Wagner M, et al. Exercise attenuates inflammation and limits scar thinning after myocardial infarction in mice. Am J Physiol Heart Circ Physiol 2015;309:H345-59. [Crossref] [PubMed]
- Xu X, Wan W, Powers AS, et al. Effects of exercise training on cardiac function and myocardial remodeling in post myocardial infarction rats. J Mol Cell Cardiol 2008;44:114-22. [Crossref] [PubMed]
- Lima MSM, Dalçóquio TF, Abduch MCD, et al. Influence of Physical Training after a Myocardial Infarction on Left Ventricular Contraction Mechanics. Arq Bras Cardiol 2023;120:e20220185. [Crossref] [PubMed]
- Albouaini K, Egred M, Alahmar A, et al. Cardiopulmonary exercise testing and its application. Postgrad Med J 2007;83:675-82. [Crossref] [PubMed]
- Ross R, Blair SN, Arena R, et al. Importance of Assessing Cardiorespiratory Fitness in Clinical Practice: A Case for Fitness as a Clinical Vital Sign: A Scientific Statement From the American Heart Association. Circulation 2016;134:e653-99. [Crossref] [PubMed]
- Ferguson B. ACSM’s guidelines for exercise testing and prescription 9th Ed. 2014. J Can Chiropr Assoc 2014;58:328.
- Gulati M, Black HR, Shaw LJ, et al. The prognostic value of a nomogram for exercise capacity in women. N Engl J Med 2005;353:468-75. [Crossref] [PubMed]
- Woodson RD. Hemoglobin concentration and exercise capacity. Am Rev Respir Dis 1984;129:S72-5. [Crossref] [PubMed]
- Ferrari M, Manea L, Anton K, et al. Anemia and hemoglobin serum levels are associated with exercise capacity and quality of life in chronic obstructive pulmonary disease. BMC Pulm Med 2015;15:58. [Crossref] [PubMed]
- Higginbotham MB, Morris KG, Williams RS, et al. Physiologic basis for the age-related decline in aerobic work capacity. Am J Cardiol 1986;57:1374-9. [Crossref] [PubMed]
- Arena R, Sietsema KE. Cardiopulmonary exercise testing in the clinical evaluation of patients with heart and lung disease. Circulation 2011;123:668-80. [Crossref] [PubMed]
- Tashiro H, Tanaka A, Ishii H, et al. Reduced exercise capacity and clinical outcomes following acute myocardial infarction. Heart Vessels 2020;35:1044-50. [Crossref] [PubMed]
- Sato T, Morishita S, Ono M, et al. Peak exercise oxygen uptake and changes in renal function in patients after acute myocardial infarction. Heart Lung 2023;57:277-82. [Crossref] [PubMed]
- Samuel TJ, Kitzman DW, Haykowsky MJ, et al. Left ventricular diastolic dysfunction and exercise intolerance in obese heart failure with preserved ejection fraction. Am J Physiol Heart Circ Physiol 2021;320:H1535-42. [Crossref] [PubMed]
- Barmeyer A, Müllerleile K, Mortensen K, et al. Diastolic dysfunction in exercise and its role for exercise capacity. Heart Fail Rev 2009;14:125-34. [Crossref] [PubMed]
- Fontes-Carvalho R, Sampaio F, Teixeira M, et al. Left ventricular diastolic dysfunction and E/E' ratio as the strongest echocardiographic predictors of reduced exercise capacity after acute myocardial infarction. Clin Cardiol 2015;38:222-9. [Crossref] [PubMed]
(English Language Editor: J. Gray)