Effects of human umbilical cord blood mononuclear cells on ovalbumin-induced asthma in mice
Highlight box
Key findings
• Intravenous administration of human umbilical cord blood mononuclear cells (UCMNCs) can inhibit airway hyperresponsiveness, T-helper type 2 inflammation, and airway injury in mice with ovalbumin (OVA)-induced asthma.
What is known and what is new?
• Mononuclear cells from umbilical cord blood and bone marrow have broad immune-modulation effects, which may be helpful for treating asthma.
• UCMNCs can inhibit the OVA-induced asthma in mouse, but the effects are not fully synchronized with the treatment dose.
What is the implication, and what should change now?
• UCMNCs at appropriate dose may be a potential solution for treating asthma.
Introduction
Asthma is a chronic airway disease characterized by variable symptoms such as wheezing, coughing, and reversible airflow limitation (1). It was estimated that 262 million people were affected by asthma in 2019 (2), and the rate of incidence is increasing. Over 50% of asthma patients have high type 2 inflammation, which modulates immune mechanisms that are important in asthma and contributes to eosinophilic inflammation, airway hyperresponsiveness (AHR), and airway remodeling (3). Inhaled and systemic corticosteroids are the cornerstone treatments for asthma, but long-term use of corticosteroids may induce multiple complications (4). Thus, novel asthma treatments targeting inflammation are needed.
Mononuclear cell (MNC) populations in bone marrow and umbilical cord blood (UCB) contain high levels of progenitor cells such as mesenchymal stem cells (MSCs), hematopoietic cells, endothelial progenitor cells, immune cells, and various cytokines produced by these cells (5). MNCs can be easily separated from bone marrow and UCB and used in clinical routines without further in vitro manipulation. Compared with MNCs obtained from adult bone marrow MNCs (BMMNCs), umbilical cord blood MNCs (UCMNCs) have higher cell viability and lower reaction to cytotoxic T cells and T-helper (Th)1 cells (5). The therapeutic potential of UCMNCs has been reported in many animal models involving bronchopulmonary dysplasia (6), neonatal lung injury (7), and acute myocardial infarction (8), and in some clinical studies for diseases such as multiple system atrophy (9) and spastic cerebral palsy (10). The non-invasiveness, high accessibility, and few ethical constraints of UCMNCs make them a suitable alternative to BMMNCs. Some pre-clinical studies have demonstrated that systemic or intratracheal administration of BMMNCs has anti-asthma effects such as reducing eosinophil infiltration, alleviating airway remodeling, and decreasing the level of asthmatic inflammatory factors in mice with ovalbumin (OVA)-induced asthma (11,12). Various other studies have reported anti-asthmatic effects of UCMSCs (13,14).
Although it seems that UCMNCs could be an option for asthma treatment, there is little research on this use so far. Our study aimed to expand the knowledge by investigating the effects of human UCMNCs on AHR, inflammation, and tissue injury in an experimental asthma model of mice induced by OVA. We present this article in accordance with the ARRIVE reporting checklist (available at https://jtd.amegroups.com/article/view/10.21037/jtd-22-1669/rc).
Methods
Animals
The experiments were approved by the Institutional Animal Care and Use Committee at Guangdong Laboratory Animals Monitoring Institute (No. IACUC2019076), in compliance with institutional guidelines for the care and use of animals. Specific pathogen-free pregnant and 6-week-old male BALB/c mice (20–23 g) were provided by Vital River Laboratory Animal Technology Co., Ltd. (Beijing, China). Mice were kept under standard conditions (12-h light and 12-h dark cycle, 23±3 °C, humidity 40–70%, 4–6 mice per cage), and fed with sterilized water and food ad libitum. One hundred and forty male mice were randomly divided into 7 groups according body weight interval (n=20 per group): control group, model group (OVA-induced asthma + saline treatment), dexamethasone group [DEX group: OVA-induced asthma + 1 mg/kg DEX (orally)], UCMNC treatment groups [low-dose UCMNC (UCMNCL), medium-dose UCMNC (UCMNCM), and high-dose UCMNC (UCMNCH) groups: OVA-induced asthma + UCMNCs (intravenous) at 4×105 cells per mouse, 2×106 cells per mouse, and 107 cells per mouse, respectively], and a fetal mouse blood MNCs (FMMNCs) treatment group [FMMNC group: OVA-induced asthma + FMMNCs (intravenous) at 2×106 cells per mouse]. The dose gradient of UCMNCs was set based on preliminary experiments and literatures (12). Some animals died during modeling or tail vein injection operations, resulting in differences in the number of animals in different groups at the end of experiment.
Human and fetal mouse MNCs
Human UCMNC preparation
The study was conducted in accordance with the Declaration of Helsinki (as revised in 2013). The good manufacturing practice (GMP)-grade human UCMNCs were provided by Cedicine Bio-Tech Co., Ltd. (Guangzhou, China). Following neonatal delivery, UCB was collected with maternal informed consent using a blood collection bag containing anticoagulant (Baxter, Deerfield, IL, USA) (15). Within 24 hours after collection, the blood was tested for pathogens including human immunodeficiency virus (HIV), cytomegalovirus, hepatitis B and C, and Treponema pallidum, and then MNCs were separated from the UCB by Ficoll-Paque PLUS (Amersham, Uppsala, Sweden). As assessed by flow cytometry, CD34+ and CD133+ cells both exceeded 1% in the prepared human UCMNCs. Then, the human UCMNCs were washed twice in DMEM and cryopreserved in sterile cryovials with 10% dimethyl sulfoxide (DMSO) in a concentration of 3×107 cells/mL.
FMMNC preparation
The pregnancy of mice was confirmed by the presence of a vaginal plug. The pregnant BALB/c mice were euthanized by CO2 at the E18. Fetal mouse blood was harvested from jugular vein of 10 fetal mice as previously described (16) and mixed in a 50 mL centrifuge tube containing a citrate anticoagulant. Then, we used Ficoll-Paque PLUS solution (Amersham) to separate the MNCs from the blood. The FMMNCs of CD34+ and CD133+ cells both exceeded 1% of the total.
Cell counts and viability of human UCMNCs and the FMMNCs were assessed again with 0.4% trypan blue dye before use. The concentrations for intravenous injection were then adjusted as follows: 4×103 cells/µL for the UCMNCL group, 2×104 cells/µL for the UCMNCM group, 105 cells/µL for the UCMNCH group, and 2×104 cells/µL for the FMMNC group.
OVA sensitization and challenge
Mice were intraperitoneally injected with 25 µg OVA per mouse with equal volumes of Imject Alum adjuvant (Thermo Fisher, Waltham, MA, USA) in 200 µL saline on days 1, 7, and 14, and intranasally instilled with 50 µg OVA in 10 µL saline per mouse during isoflurane anesthesia on days 21, 22, 23, and 24. The control group was administered the same amount of normal saline for sensitization and challenge. As most of intravenously injected exogenous cells can be enriched at lung capillary network for up to several days (17), mice in the treatment groups were intravenously injected via tail vein in 30 s with 100 µL of cell suspension per mouse with low, medium, or high doses of MNCs on days 7 and 20, while the control group and model group were treated with normal saline at the same time. DEX was administered to the DEX group for 5 days, from days 20 to 24.
Airway responsiveness
The airway responsiveness of the mice was measured using Buxco small animal whole-body plethysmography (WBP) (DSI, New Brighton, MN, USA) 24 hours after the last intranasal provocation. Each animal was unrestrained and conscious in WBP for mice and challenged by nebulized methacholine (Sigma, St. Louis, MO, USA) in increasing concentrations of 0, 1.56, 3.125, 6.25, and 12.5 mg/mL for 30 s at each concentration. Enhanced pause (Penh) is a dimensionless index based on the expiratory wave shape in WBP system, which is correlated with airway resistance (18). Average of Penh during the 3 min after each methacholine challenge was calculated and the change of Penh was used to reflect the airway responsiveness (13).
Analysis of bronchoalveolar lavage fluid (BALF) and serum
The BALF and serum were collected from the mice not challenged by methacholine after CO2 euthanasia. In total, 0.7 mL of saline per time for three times was used to lavage the lung. The lavage was then pooled and centrifuged at 2,000 rpm for 10 min. The serum was obtained by cardiac puncture. We used Luminex liquid phase chip technology (R&D Systems, Minneapolis, MN, USA) to measure the interleukin (IL)-4, IL-5, IL-13, and IL-17 levels in the supernatant of centrifuged BALF and serum samples. Differential leukocyte counts of BALF were analyzed with the Sysmex animal hematology analyzer (XN-1000V, Sysmex Corporation, Kobe, Japan) (19,20). The cell pallets from the BALF were re-suspended with 100 µL of cold Hank’s balanced salt solution (HBSS) with 5% fetal bovine serum, and incubated with antibodies anti-mouse CD45-APC-Cy7, anti-mouse CD3-FITC, anti-mouse CD4-APC, and anti-mouse CD8-Percp-Cy5.5 (BD, Franklin Lakes, NJ, USA) at the manufacturer’s recommended concentration at 4 °C for 30 min. Then the cells were washed with fluorescence-activated cell sorting (FACS) buffer and assayed by flow cytometry (FACS Canto2, BD) to measure CD4 and CD8 T cell subsets.
Histopathological analysis
Mice were euthanized by CO2 after measuring airway responsiveness, and the lung tissues were collected for histopathological examination. The left and right lungs were fixed with 10% each of neutral formalin and Carnoy’s solution. The largest longitudinal section of the lung lobes was stained using hematoxylin and eosin (HE). The airway inflammation was semi-scored from 0 (normal) to 4 (severe) based on inflammatory cell infiltration around the airway.
Statistical analysis
The mice accidentally died before the endpoint measurements or failed in sampling were not included in the data analysis. Statistical analysis was performed using SPSS Statistics 22.0 (IBM, Armonk, NY, USA). One-way analysis of variance (ANOVA) was used for variance analysis followed by a post-hoc test for multiple comparisons. For the data failed Levene’s homogeneity of variance test, Kruskal-Wallis test and Mann-Whitney U test were used. A P value <0.05 was taken as significant.
Results
Airway responsiveness
Compared with the control group, airway responsiveness was significantly higher in the model group, which could be markedly suppressed by DEX administration (all had a P value <0.01 at 1.56–12.5 mg/mL of methacholine). Compared with the model group, only the UCMNCL group had a significant decrease in airway responsiveness to a challenge of 1.56–6.25 mg/mL methacholine, while the FMMNC group showed a somewhat but not significant decrease of airway responsiveness (Figure 1).
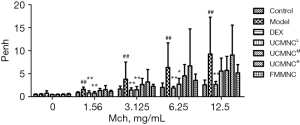
Leukocyte counts in BALF
Compared with control group, the counts of total leukocytes, as well as eosinophils, neutrophils, and lymphocytes in the BALF were significantly higher in the model group (P<0.05), while DEX administration showed marked inhibition on the total and differential leukocyte counts. A somewhat but not significant decrease of eosinophils was shown in the UCMNCM group and the FMMNC group (Figure 2).
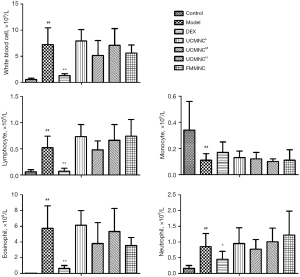
Histopathological evaluation of airway inflammation
Histopathological examination and semi-scoring of sections stained with HE showed a significant increase of airway inflammatory infiltration in all mice with OVA-induced asthma. The inflammation scores were significantly decreased only in the DEX group and UCMNCM group (P<0.05) (Figure 3).
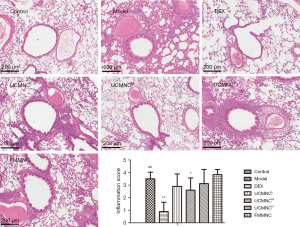
Cytokine levels in BALF and serum
Compared with the control group, levels of IL-4, IL-5, and IL-13 in BALF, and levels of IL-5, IL-13, and IL-17 in serum were significantly increased in the model group. The DEX group showed significant suppression of IL-4, IL-5, and IL-13 levels in BALF, and of IL-5 and IL-13 levels in serum. The UCMNCL group had significant inhibition of only IL-5 in BALF. In the UCMNCM group, IL-5 and IL-13 were significantly reduced in BALF, and IL-5 and IL-13 were somewhat but not significantly reduced in serum (both P<0.1). The UCMNCH had significant inhibition of only IL-17 in the serum. The suppressive effects of UCMNCs on IL-5 in BALF and IL-13 in serum for the UCMNCH group were slight but not significant (both P<0.1). In the allograft control (FMMNC) group, a medium dose of treatment significantly reduced IL-13 and IL-17 in serum, with slight inhibition of IL-5 in BALF (P<0.1). These results were similar to the effects of a high dose of UCMNCs (Figures 4,5).
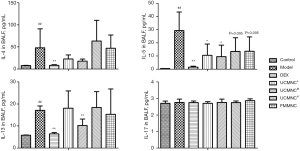
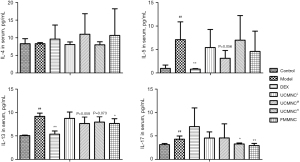
CD4 and CD8 T cell subsets
Compared with the control group, the CD4−CD8− T cells in BALF were increased and the CD4+CD8− T cells and CD4/CD8 ratio were decreased in the model group. Compared with the model group, DEX treatment significantly increased the CD4−CD8− T cells and decreased the CD4+CD8− T cells. UCMNC treatments dose-dependently reduced the CD4−CD8− T cells and increased the CD4+CD8− T cells in BALF of the asthmatic mice, with statistically significant effects in the UCMNCH group. Additionally, CD4+CD8+ T cells were significantly reduced in the UCMNCL group. FMMNCs modulated T cell subsets of asthmatic mice airways in a pattern that differed from treatment with UCMNCs and DEX. The FMMNC group had markedly increased CD4+CD8+ and CD4−CD8+ T cells and decreased CD4−CD8− and CD4+CD8− T cells, and the CD4/CD8 ratio was reversed (Figure 6).
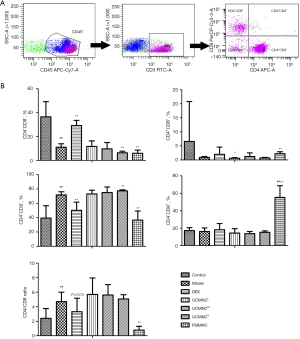
Discussion
Asthma is a chronic airway disease with symptoms including reversible airflow restriction and AHR. Allergy-induced Th1/Th2 immune imbalance and eosinophilic inflammation are the key pathological mechanisms. In present research, it was showed that UCMNCs could significantly reduce levels of IL-5, IL-13, and eosinophilic infiltration, and suppress AHR in airways of asthmatic mice. These suggest a potential use for UCMNCs in treating asthma.
Elevation of Th2-related cytokines IL-4, IL-5, and IL-13 can induce expression of immunoglobulins in B cells, attraction and activation of eosinophils, metaplasia of epithelial goblet cells, and hyperplasia of smooth muscle, resulting in airway inflammation, mucus hypersecretion, and AHR (21). Several therapies targeting IL-4, IL-5, and IL-13 have shown encouraging results in clinical trials for severe asthma (22,23). In this study, intravenous injection of UCMNCs showed inhibitory effect on IL-5 in BALF of asthmatic mice, with the strongest inhibition produced in the UCMNCM group (2×106 UCMNCs per mouse). In addition, the same dose of UCMNCs also had a significant inhibitory effect on the IL-13 in BALF and showed an inhibitory trend (though not statistically significant) in the IL-5 and IL-13 in serum. One possible reason may be that the injected exogenous UCMNCs tend to be enriched in the capillary network of lung (24), which may alter the effect degrees of intravenously injected UCMNC on the inflammation cytokines in lung (BALF) and in peripheral blood (serum). However, this down-regulation in cytokines is consistent with the suppression of airway inflammation revealed by histology and the eosinophil inflammation in cytology analysis in this study. Our results suggest that human UCMNC administration can reduce Th2-related cytokines such as IL-5 and IL-13 and inhibit asthmatic airway inflammation and injury. However, besides the Th2 cells, IL-5 and IL-13 can be also produced by various cells such as type 2 innate lymphoid cells and mast cells. Thus, the target immune cells modulated by UCMNC in treating OVA-induced asthma in mouse model are still unclear in present study.
IL-17 is considered to be associated with neutrophilic inflammation and corticoid resistance in severe asthma (25). Although the OVA challenge does not always elevate airway IL-17 levels in mice, as shown in the present study and one other report (26), the significant increase in neutrophil infiltration in BALF and IL-17 level in serum suggests neutrophilic inflammation in the asthmatic mice. Both high-dose human UCMNCs and FMMNCs significantly reduced IL-17 levels in asthmatic mouse serum, but they did not decrease neutrophil infiltration in the mouse airways. Thus, the exact mechanism and effect of UCMNC on neutrophilic inflammation in asthma has not yet been determined. Asthma animal models using house dust mite or toluene diisocyanate, which have been reported to induce more typical airway neutrophilic inflammation (27,28), may be helpful in examining this.
AHR is one of the main physiological symptoms of asthma. Airway inflammation, airway remodeling, and dysfunction of peripheral nerve control are all involved in AHR (29,30). Interestingly, the inhibitory effect of human UCMNCs on airway responsiveness to methacholine occurred only in the UCMNCL group, while their inhibitory effect on type 2 inflammation and eosinophil infiltration appeared in higher doses. This suggests that the effect of UCMNCs on inhibiting AHR is independent of their anti-inflammatory effect. A relative dissociation between AHR and inflammatory infiltration has been reported in asthmatic patients, indicating that airway wall remodeling or autonomic nerve dysfunction may be more closely related to AHR (31). However, a low dose of UCMNCs did not significantly affect airway wall remodeling in our study. Thus, it is worth exploring the effect of UCMNCs on airway neurological dysfunction in asthma, which has been found as an important target in radio-frequency ablation therapy for severe asthma and chronic obstructive pulmonary disease (32,33).
It has been reported that xenograft and allograft can produce different effect on the immune functions of recipient (34). Our study used mice injected with FMMNCs as an approximate allogenic control to discover possible xenogeneic interference of human cell administration (35). We found some similarity between the FMMNC group and the human UCMNC groups in effects on AHR and inflammatory cytokines. However, effects of allograft MNC on the airway CD4/CD8 T cell subsets in mice were quite different from those of human UCMNC xenograft. Our results showed that high dose of UCMNCs significantly increased the airway CD4+CD8− Th cells in asthmatic mice, while FMMNCs significantly increased the CD4−CD8+ T-killer cells and decreased the CD4+CD8− Th cells. In a study of mouse xenograft heart transplantation, it was found that the rejection of recipient mice to xenograft rat heart mainly depends on CD4 T cells, and the presentation of donor antigens by the mouse’s major histocompatibility complex (MHC) II is crucial in xenograft rejection (36). In present study, human UCMNC intravenously injected into mice was a xenograft and also caused a significant increase in CD4 T cells, which may also be related to the presentation of human UCMNC cell antigens by the MHC II of mouse antigen presentation cells. But it should be noted that the mechanism for rejection in one transplant model do not necessarily work for another transplant model. The details about the rejection of human UCMNC allograft in asthma patients should be accessed in future clinical research. This suggests that species difference should be considered when applying the pre-clinical findings from mice in our study to future asthma patients.
UCMNCs consist of hematopoietic stem cells, MSCs, and immune cells (5). MSCs have multiple immunomodulatory effects on Th2 cells, type 2 innate lymphoid cells, and regulatory T (Treg) cells, and anti-asthmatic effects of cultured pure MSCs have been broadly reported in preclinical animal studies (37). Besides, CD11b+ cells (monocytes, macrophages, dendritic cells) in MNCs can also contribute to the anti-asthmatic effects possibly by promoting M2 macrophages and Treg cells (12). But in this study, the best effects of human MNC administration on airway inflammation and AHR did not occur at the highest dose, but instead at low or medium doses. This untypical dose-response relationship is not rare in studies of cell therapy (14,38). One possible reason is that a high dose of cell injection may cause pulmonary microvascular obstruction (39). Also, hematopoietic stem cells in MNC may be enriched in airways after intravenous injection and differentiate into eosinophils under the induction of an allergic inflammatory microenvironment (40). These conditions may interfere the therapeutic effect of leukocytes and MSCs on asthmatic airway inflammation (12). Therefore, cell dose and risk-benefit balance might need to be carefully considered when using UCMNCs to treat asthma. It’s better to choose a relatively low dose in future preclinical or clinical researches and intratracheally instilling UCMNC might help reduce the risk and enhance the effect (14). Besides, the intervention protocol of UCMNC might be further optimized in the administration route, frequency, and treatment timing through specially designed experiments in future research.
Conclusions
The present study shows that intravenous administration of human UCMNC can inhibit AHR, Th2 inflammation, and airway injury in mice with OVA-induced asthma. UCMNCs at the appropriate dose may be a potential solution for treating asthma.
Acknowledgments
Funding: This work was supported by
Footnote
Reporting Checklist: The authors have completed the ARRIVE reporting checklist. Available at https://jtd.amegroups.com/article/view/10.21037/jtd-22-1669/rc
Data Sharing Statement: Available at https://jtd.amegroups.com/article/view/10.21037/jtd-22-1669/dss
Peer Review File: Available at https://jtd.amegroups.com/article/view/10.21037/jtd-22-1669/prf
Conflicts of Interest: All authors have completed the ICMJE uniform disclosure form (available at https://jtd.amegroups.com/article/view/10.21037/jtd-22-1669/coif). YLL reports funding from the Basic Research Foundation of Guangzhou (No. 202102020916). CQH reports funding from the Zhongnanshan Medical Foundation of Guangdong Province (No. ZNSA-2020013). SYL reports funding from the University Innovation Team Project of Guangdong Province (No. 2021KCXTD028), the Science and Technology Program of Guangzhou (No. 202102010358), and the Zhongnanshan Medical Foundation of Guangdong Province (Nos. ZNSA-2020013 and ZNSA-2020003). The other authors have no conflicts of interest to declare.
Ethical Statement: The authors are accountable for all aspects of the work in ensuring that questions related to the accuracy or integrity of any part of the work are appropriately investigated and resolved. The experiments were approved by the Institutional Animal Care and Use Committee at Guangdong Laboratory Animals Monitoring Institute (No. IACUC2019076), in compliance with institutional guidelines for the care and use of animals. The study was conducted in accordance with the Declaration of Helsinki (as revised in 2013). The good manufacturing practice (GMP)-grade human umbilical cord blood mononuclear cells were provided by Cedicine Bio Tech Co., Ltd. (Guangzhou, China). Following neonatal delivery, umbilical cord blood was collected with maternal informed consent using a blood collection bag containing anticoagulant.
Open Access Statement: This is an Open Access article distributed in accordance with the Creative Commons Attribution-NonCommercial-NoDerivs 4.0 International License (CC BY-NC-ND 4.0), which permits the non-commercial replication and distribution of the article with the strict proviso that no changes or edits are made and the original work is properly cited (including links to both the formal publication through the relevant DOI and the license). See: https://creativecommons.org/licenses/by-nc-nd/4.0/.
References
- Papi A, Brightling C, Pedersen SE, et al. Asthma. Lancet 2018;391:783-800. [Crossref] [PubMed]
- Global burden of 369 diseases and injuries in 204 countries and territories, 1990-2019: a systematic analysis for the Global Burden of Disease Study 2019. Lancet 2020;396:1204-22. [Crossref] [PubMed]
- Price D, Wilson AM, Chisholm A, et al. Predicting frequent asthma exacerbations using blood eosinophil count and other patient data routinely available in clinical practice. J Asthma Allergy 2016;9:1-12. [Crossref] [PubMed]
- Sobieraj DM, Baker WL, Nguyen E, et al. Association of Inhaled Corticosteroids and Long-Acting Muscarinic Antagonists With Asthma Control in Patients With Uncontrolled, Persistent Asthma: A Systematic Review and Meta-analysis. JAMA 2018;319:1473-84. [Crossref] [PubMed]
- Paloczi K. Immunophenotypic and functional characterization of human umbilical cord blood mononuclear cells. Leukemia 1999;13:S87-9. [Crossref] [PubMed]
- Chen J, Chen Y, Du X, et al. Integrative Studies of Human Cord Blood Derived Mononuclear Cells and Umbilical Cord Derived Mesenchyme Stem Cells in Ameliorating Bronchopulmonary Dysplasia. Front Cell Dev Biol 2021;9:679866. [Crossref] [PubMed]
- Mills DR, Mao Q, Chu S, et al. Effects of human umbilical cord blood mononuclear cells on respiratory system mechanics in a murine model of neonatal lung injury. Exp Lung Res 2017;43:66-81. [Crossref] [PubMed]
- Henning RJ, Shariff M, Eadula U, et al. Human cord blood mononuclear cells decrease cytokines and inflammatory cells in acute myocardial infarction. Stem Cells Dev 2008;17:1207-19. [Crossref] [PubMed]
- Yu H, Yuan X, Zhao M, et al. Efficacy of Human Umbilical Cord Blood-Mononuclear Cell Transplantation for MSA Treatment and Its Effects on Changes in T-Cell Subsets in Peripheral Blood and Inflammatory Factors. Dis Markers 2021;2021:5290766. [Crossref] [PubMed]
- Zarrabi M, Akbari MG, Amanat M, et al. The safety and efficacy of umbilical cord blood mononuclear cells in individuals with spastic cerebral palsy: a randomized double-blind sham-controlled clinical trial. BMC Neurol 2022;22:123. [Crossref] [PubMed]
- Abreu SC, Antunes MA, Maron-Gutierrez T, et al. Bone marrow mononuclear cell therapy in experimental allergic asthma: intratracheal versus intravenous administration. Respir Physiol Neurobiol 2013;185:615-24. [Crossref] [PubMed]
- Cruz FF, Borg ZD, Goodwin M, et al. CD11b+ and Sca-1+ Cells Exert the Main Beneficial Effects of Systemically Administered Bone Marrow-Derived Mononuclear Cells in a Murine Model of Mixed Th2/Th17 Allergic Airway Inflammation. Stem Cells Transl Med 2016;5:488-99. [Crossref] [PubMed]
- Kang SY, Park DE, Song WJ, et al. Immunologic regulatory effects of human umbilical cord blood-derived mesenchymal stem cells in a murine ovalbumin asthma model. Clin Exp Allergy 2017;47:937-45. [Crossref] [PubMed]
- Shin JW, Ryu S, Ham J, et al. Mesenchymal Stem Cells Suppress Severe Asthma by Directly Regulating Th2 Cells and Type 2 Innate Lymphoid Cells. Mol Cells 2021;44:580-90. [Crossref] [PubMed]
- Rubinstein P, Dobrila L, Rosenfield RE, et al. Processing and cryopreservation of placental/umbilical cord blood for unrelated bone marrow reconstitution. Proc Natl Acad Sci U S A 1995;92:10119-22. [Crossref] [PubMed]
- Wen C, Liu XY, Wan WQ, et al. Effects of Fetal and Neonatal Murine Peripheral Blood Mononuclear Cells Infusion on MicroRNA-145 Expression in Renal Vascular Smooth Muscle Cells in MRL/lpr Mice. Transplant Proc 2015;47:2523-7. [Crossref] [PubMed]
- Xu XP, Huang LL, Hu SL, et al. Genetic Modification of Mesenchymal Stem Cells Overexpressing Angiotensin II Type 2 Receptor Increases Cell Migration to Injured Lung in LPS-Induced Acute Lung Injury Mice. Stem Cells Transl Med 2018;7:721-30. [Crossref] [PubMed]
- Lomask M. Further exploration of the Penh parameter. Exp Toxicol Pathol 2006;57:13-20. [Crossref] [PubMed]
- Mathers RA, Evans GO, Bleby J, et al. Total and differential leucocyte counts in rat and mouse bronchoalveolar lavage fluids using the Sysmex XT-2000iV. Comparative Clinical Pathology 2007;16:29-39.
- Poitout-Belissent F, Grant SN, Tepper JS. Aspiration and Inspiration: Using Bronchoalveolar Lavage for Toxicity Assessment. Toxicol Pathol 2021;49:386-96. [Crossref] [PubMed]
- Fahy JV. Type 2 inflammation in asthma--present in most, absent in many. Nat Rev Immunol 2015;15:57-65. [Crossref] [PubMed]
- Ramonell RP, Iftikhar IH. Effect of Anti-IL5, Anti-IL5R, Anti-IL13 Therapy on Asthma Exacerbations: A Network Meta-analysis. Lung 2020;198:95-103. [Crossref] [PubMed]
- Kardas G, Kuna P, Panek M. Biological Therapies of Severe Asthma and Their Possible Effects on Airway Remodeling. Front Immunol 2020;11:1134. [Crossref] [PubMed]
- Cruz FF, Rocco PRM. The potential of mesenchymal stem cell therapy for chronic lung disease. Expert Rev Respir Med 2020;14:31-9. [Crossref] [PubMed]
- Chesné J, Braza F, Mahay G, et al. IL-17 in severe asthma. Where do we stand? Am J Respir Crit Care Med 2014;190:1094-101. [Crossref] [PubMed]
- Hanashiro J, Muraosa Y, Toyotome T, et al. Schizophyllum commune induces IL-17-mediated neutrophilic airway inflammation in OVA-induced asthma model mice. Sci Rep 2019;9:19321. [Crossref] [PubMed]
- Wada T, Hirahara K, Aoki A, et al. An optimized protocol for the analysis of house dust mite (Dermatophagoides farinae)-induced neutrophil-dominant airway inflammation. J Immunol Methods 2019;465:53-60. [Crossref] [PubMed]
- Chen R, Zhang Q, Chen S, et al. IL-17F, rather than IL-17A, underlies airway inflammation in a steroid-insensitive toluene diisocyanate-induced asthma model. Eur Respir J 2019;53:1801510. [Crossref] [PubMed]
- Wang KCW, Le Cras TD, Larcombe AN, et al. Independent and combined effects of airway remodelling and allergy on airway responsiveness. Clin Sci (Lond) 2018;132:327-38. [Crossref] [PubMed]
- Wilder JA, Collie DD, Wilson BS, et al. Dissociation of airway hyperresponsiveness from immunoglobulin E and airway eosinophilia in a murine model of allergic asthma. Am J Respir Cell Mol Biol 1999;20:1326-34. [Crossref] [PubMed]
- Crimi E, Spanevello A, Neri M, et al. Dissociation between airway inflammation and airway hyperresponsiveness in allergic asthma. Am J Respir Crit Care Med 1998;157:4-9. [Crossref] [PubMed]
- Luo YL, Cheng YQ, Zhou ZQ, et al. A clinical and canine experimental study in small-airway response to bronchial thermoplasty: Role of the neuronal effect. Allergol Int 2022;71:66-72. [Crossref] [PubMed]
- Slebos DJ, Klooster K, Koegelenberg CF, et al. Targeted lung denervation for moderate to severe COPD: a pilot study. Thorax 2015;70:411-9. [Crossref] [PubMed]
- Masuda J, Shigehiro T, Matsumoto T, et al. Cytokine Expression and Macrophage Localization in Xenograft and Allograft Tumor Models Stimulated with Lipopolysaccharide. Int J Mol Sci 2018;19:1261. [Crossref] [PubMed]
- Huang CP, Chen CC, Shyr CR. Xenogeneic cell therapy provides a novel potential therapeutic option for cancers by restoring tissue function, repairing cancer wound and reviving anti-tumor immune responses. Cancer Cell Int 2018;18:9. [Crossref] [PubMed]
- Plenter RJ, Grazia TJ, Doan AN, et al. CD4 T cells mediate cardiac xenograft rejection via host MHC Class II. J Heart Lung Transplant 2012;31:1018-24. [Crossref] [PubMed]
- Zhang LB, He M. Effect of mesenchymal stromal (stem) cell (MSC) transplantation in asthmatic animal models: A systematic review and meta-analysis. Pulm Pharmacol Ther 2019;54:39-52. [Crossref] [PubMed]
- Garbuzova-Davis S, Sanberg CD, Kuzmin-Nichols N, et al. Human umbilical cord blood treatment in a mouse model of ALS: optimization of cell dose. PLoS One 2008;3:e2494. [Crossref] [PubMed]
- Coppin L, Sokal E, Stéphenne X. Thrombogenic Risk Induced by Intravascular Mesenchymal Stem Cell Therapy: Current Status and Future Perspectives. Cells 2019;8:1160. [Crossref] [PubMed]
- Fischer KD, Agrawal DK. Hematopoietic stem and progenitor cells in inflammation and allergy. Front Immunol 2013;4:428. [Crossref] [PubMed]