Long-term outcomes of robotic versus video-assisted pulmonary lobectomy for non-small cell lung cancer: systematic review and meta-analysis of reconstructed patient data
Highlight box
Key findings
• Both robotic- and video-assisted pulmonary lobectomies offer equivalent overall and disease-free survival outcomes in patients with non-small cell lung cancer.
What is known and what is new?
• Evidence regarding long-term outcomes is lacking and the literature is conflicting.
• Analysis of the most up-to-date evidence highlighted that both approaches offer similar overall and disease-free survival.
What is the implication, and what should change now?
• While further evidence is warranted from high quality studies, utilization of one approach over the other should be tailored according to the surgeon’s and the center’s experience.
Introduction
Despite the current advancements in radiotherapy and the emergence of new systemic therapies targeting molecular pathways and the immune system, pulmonary resection remains the cornerstone of treatment for patients with early-stage non-small cell lung cancer (NSCLC) (1). Open thoracotomy has traditionally been considered the mainstay approach for pulmonary resection (2). In recent decades however, the clinical benefits of a minimally invasive approach have been clearly demonstrated over thoracotomy which is associated with prolonged hospital stay, postoperative pain, time to adjuvant therapy, morbidity, and mortality. This in turn has driven growth in minimally invasive thoracic surgical approaches (2-4). Video-assisted thoracoscopic surgery (VATS) was introduced in the early 1990s and found to be feasible and safe for pulmonary lobectomy as compared to thoracotomy and its adoption has slowly spread worldwide (5-7). Robotic-assisted thoracoscopic surgery (RATS) was first described in 2002 and has been regarded as an alternative to VATS since the early 2000s (8). Of note, both VATS and RATS have been shown to offer equivalent oncologic outcomes to open thoracotomy, with significantly lower intra- and post-operative morbidity as well as shorter recovery periods (9,10). It is in this setting the thoracic surgery space has seen a steady increase in minimally invasive approaches inclusive of VATS and RATS compared to open thoracotomy, especially in recent years (3,9).
While the body of evidence demonstrating the superiority of minimally invasive approaches for pulmonary resection is growing, the debate regarding which minimally invasive approach is superior has not been settled (11). The literature regarding the early outcomes following RATS versus VATS lobectomy is conflicted (12,13) and unfortunately evidence regarding long-term outcomes is lacking. More specifically, only a few single institutional studies comparing RATS versus VATS with a focus on long-term outcomes are present in the literature but are limited by small sample size while prospective randomized clinical trials on this topic began recruiting patients only recently and therefore lack long-term data (12,14). In addition, studies utilizing large databases cannot assess disease-free survival (DFS) because such databases do not capture cancer recurrence rates (15,16). In order to clarify the role of RATS versus VATS in patients undergoing lobectomy for NSCLC, we performed a systematic review and meta-analysis of the current literature and compared both the short- and long-term outcomes between the two surgical approaches. Aiming to maximize the robustness of our study and provide the best evidence synthesis to date, we reconstructed patient-level time-to-event data from all the individual studies to calculate the crude overall survival (OS) and DFS rates (17). We present this article in accordance with the PRISMA reporting checklist (available at https://jtd.amegroups.com/article/view/10.21037/jtd-23-582/rc) (18).
Methods
Study design and inclusion/exclusion criteria
This systematic review and meta-analysis were prospectively registered in the PROSPERO database (registration number: CRD42022376311). We applied the PICO (Population/Participants, Intervention, Comparison and Outcome) criteria to define our research question:
- Population/participants: adult patients undergoing lobectomy for NSCLC;
- Intervention: RATS;
- Comparison group: VATS;
- Outcomes: the primary assessed outcomes were long-term OS. The secondary outcomes assessed were the DFS postoperative complications (including postoperative bleeding, pneumonia, pulmonary embolism, chylothorax, wound infection, cardiopulmonary failure, renal insufficiency, and gastrointestinal bleeding), prolonged air leak, conversion to open thoracotomy, operative time, length of hospital stay, and number of dissected lymph nodes.
Retrospective or prospective studies reporting on the outcomes of interest in patients with NSCLC undergoing lobectomy via RATS versus VATS were included. Exclusion criteria were defined as follows: (I) studies including small cell lung cancer or benign lung tumors; (II) studies published in a language other than English; (III) non-comparative studies; (IV) studies reporting only early outcomes and not long-term outcomes; (V) meta-analyses, systematic reviews, editorials, letters to the editor; (VI) studies with unextractable long-term data. In cases where multiple studies reported on the same population, only the best quality of data was selected for the present meta-analysis.
Search strategy
We searched the MEDLINE (via PubMed), Scopus, and Cochrane Library databases (last search: December 16th, 2022) using the algorithm: (robot-assisted OR robot-assisted thoracic surgery OR robot OR robotic OR computer-assisted surgery OR da Vinci) AND (video-assisted OR video-assisted thoracic surgery OR video OR thoracoscopic) AND (non-small cell lung cancer OR lung cancer OR lung carcinoma). No time restrictions were applied to our search. Title and abstract screening and full text eligibility were assessed by two independent investigators (Tasoudis PT and Diehl JN). Any disagreement was resolved after discussion with a third reviewer (Long JM). We also hand-searched for potentially eligible studies using the snowball methodology (19). The Covidence reference and article manager software was used for all stages of the database search and study selection (20).
Data extraction and assessment of risk of bias
Two investigators independently extracted the data into a pre-designed table. Patients’ baseline characteristics as well as peri-operative data and Kaplan-Meier curves were collected. The Risk of Bias in Non-Randomized Studies of Interventions tool (ROBINS-I) was systematically used to assess included studies for risk of bias in the included studies (21). The papers and their characteristics were classified into low, moderate, serious, or critical risk of bias with ROBINS-I tool. Two independent reviewers assessed risk for bias (Tasoudis PT and Diehl JN). When there was disagreement, a third reviewer checked the data and made the final decision (Long JM).
Statistical analysis
Data pooling and meta-analysis
Continuous variables were summarized using means and standard deviations, while categorical variables using frequencies and percentages. The Hozo et al. and the Wan et al. methods were used to estimate the means and standard deviations of continuous variables whenever medians and ranges (22) and median and interquartile ranges were provided (23), respectively. Data were extracted and entered into tables and the outcomes were analyzed cumulatively.
To compare the secondary outcomes, we used the odds ratio (OR) and 95% confidence interval (95% CI). An OR greater than 1 indicated that the outcome was more frequently present in the RATS arm. Continuous variables were analyzed using the standardized mean difference (SMD) and 95% CI, and an SMD >0 corresponded to larger values in the RATS arm. Random-effects models (DerSimonian-Laird) were adopted to balance inherent clinical heterogeneity between the included studies. Forest plots were generated to display results. Between-study statistical heterogeneity was assessed with the Cochran Q statistic and by estimating I2. High heterogeneity was confirmed with a significance level of P<0.10 and I2 ≥ 50%. Publication bias was assessed via funnel plots and Egger’s test for each outcome of interest and P<0.10 was considered statistically significant. Statistical analysis was performed using Stata/SE version 17 (Stata Corp, College Station, TX, USA).
Reconstruction of individual patient survival data
We used the methods described by Wei et al. to reconstruct IPD from the Kaplan-Meier curves of all eligible studies for the long-term survival outcomes (24). The Kaplan-Meier survival curves, presented as raster and vector images, underwent preprocessing and digitization using an online software called WebPlotDigitizer. This process enabled the extraction of specific time points along with their corresponding survival and mortality data. Whenever supplementary information such as number-at-risk tables or total number of events was accessible, it was utilized to enhance the precision of the time-to-events. In order to identify and rectify deviations from a monotonic pattern, isotonic regression was employed, and any remaining inconsistencies were resolved using a pool-adjacent-violators algorithm (24,25). In order to validate the accuracy of the recorded failure event timings, we conducted a meticulous examination to ensure consistency with the survival or mortality data reported in the original publications.
One-stage meta-analysis
For both OS and DFS calculations, we utilized the Kaplan-Meier method. To assess differences between the groups, we employed the Cox proportional hazards regression model. In this model, it is assumed that every patient within each individual study has a similar likelihood of experiencing failure compared to other patients in that study. To ensure the validity of the Cox models, we conducted a comprehensive assessment of the proportional hazards assumption. This involved plotting scaled Schoenfeld residuals, log-log survival plots, and comparing predicted versus observed survival functions. Survival curves were generated using the Kaplan-Meier product limit method, and we calculated the hazard ratios (HRs) and their corresponding 95% CIs for each group.
Two-stage survival meta-analysis
As a sensitivity analysis, we calculated summary HRs and 95% CIs for all individual studies based on the reconstructed IPD and the results were verified through evaluation of the manuscript and tables of the included studies. Following that the HRs were pooled under and the conventional “two-step” meta-analysis for both OS and DFS. HRs and 95% confidence intervals (CIs) were calculated using the DerSimonian Laird random-effects model (26). A forest plot for each outcome was used to display the pooled estimates graphically. A P value <0.05 was considered significant. Between-study heterogeneity was assessed through Cochran Q statistic and by estimating I2. I2 greater than 50% and P<0.1 indicated significant heterogeneity. Publication bias was assessed via funnel plots and Egger’s test for each outcome of interest and P<0.10 was considered statistically significant. Leave-one-out sensitivity analyses were performed for OS and DFS two-stage analyses. Pre-specified random-effects meta-regression analyses were conducted to examine the impact of moderator variables on outcomes. Specifically, we attempted to assess the effect of age, gender, side, and stage of the tumor on the OS and DFS.
Results
Study and patient characteristics
The literature search yielded 680 potentially eligible articles. After removal of all duplicate records and 591 articles with irrelevant titles or abstracts, 80 potentially eligible studies remained for evaluation. These studies along with two additional articles identified through the snowball method, underwent full-text evaluation. From this pool, five studies were excluded due to overlapping populations [four database studies (15,16,27,28) and one single institutional study (29)] and two studies were excluded due to inability to extract to extract their long-term data (30,31). After full-text review, 10 studies met our eligibility criteria, as summarized in the PRISMA flowchart (Figure 1) (32-41). A total of 2,045 patients undergoing lobectomy for NSCLC were identified. Among them, 1,231 patients underwent VATS, and 814 patients underwent RATS. The baseline characteristics of the included studies and patients are summarized in Table 1 and Table S1, respectively. The tumor characteristics are presented in Tables S2,S3.
Table 1
Study | Journal | Center | Country | Design | Study period | Follow-up VATS (months) | Follow-up RATS (months) |
Total no. of patients | VATS no. of patients | RATS no. of patients |
---|---|---|---|---|---|---|---|---|---|---|
Huang et al., 2019 (38) | Journal of Thoracic Disease | Duke University Medical Center, Durham | USA | Retrospective cohort | Dec 2010–Jun 2015 | 18.2 | 18.2 | 166 | 105 | 61 |
Merritt et al., 2022 (37) | Journal of Robotic Surgery | Ohio State University, Columbus | USA | Retrospective cohort | Mar 2014–May 2018 | 36.7±2.3 | 36.7±2.3 | 200 | 100 | 100 |
Worrell et al., 2019 (35) | Journal of Robotic Surgery | University of Michigan, Ann Arbor | USA | Retrospective cohort | Nov 2010–Mar 2012 | 63±4 | 63±4 | 98 | 73 | 25 |
Yang et al., 2017 (34) | Annals of Surgery | Memorial Sloan Kettering Cancer Center, New York | USA | Retrospective cohort | Jan 2002–Dec 2012 | 59.6±23.3 | 59.6±23.3 | 344 | 172 | 172 |
Lee et al., 2015 (40) | Annals of Thoracic Surgery | The Valley Hospital/Valley Health System, Ridgewood | USA | Retrospective cohort | 2009–2014 | 20.9 | 20.9 | 211 | 158 | 53 |
Casiraghi et al., 2022 (33) | Journal of Clinical Medicine | IEO, Milan | Italy | Retrospective cohort | Jan 2011–Dec 2017 | 60 | 60 | 108 | 36 | 72 |
Haruki et al., 2020 (41) | General Thoracic and Cardiovascular Surgery | Tottori University, Yonago | Japan | Retrospective cohort | Apr 2011–Dec 2018 | 41 | 41 | 98 | 49 | 49 |
Montagne et al., 2022 (32) | Cancers | Rouen University Hospital, Rouen | France | Retrospective cohort | 2012–2020 | 28.2±34.8 | 28.2±34.8 | 670 | 436 | 234 |
Park et al., 2017 (36) | Journal of Thoracic Disease | Yonsei University College of Medicine, Seoul | Republic of Korea | Retrospective cohort | Mar 2011–Feb 2013 | 48.9±9.5 | 48.9±9.5 | 29 | 17 | 12 |
Li et al., 2019 (39) | Translational lung cancer research | Shanghai Chest Hospital, Shanghai | China | Retrospective cohort | Jan 2014–Jan 2017 | 34.6±10.5 | 34.6±10.5 | 121 | 85 | 36 |
Values are reported as mean ± standard deviation when available. VATS, video-assisted thoracoscopic surgery; RATS, robotic-assisted thoracoscopic surgery; IEO, European Institute of Oncology.
Individual patient data and Kaplan-Meier curves reconstruction
Overall, nine unadjusted Kaplan-Meier curves of OS (32-40) reporting on 814 patients undergoing RATS and 1,261 patients undergoing VATS, as well as nine Kaplan-Meier curves of unadjusted DFS (32,34,36-41) reporting on 717 patients undergoing RATS and 1,120 patients undergoing VATS were processed, digitalized, and reconstructed. Using the previously described methodology, we extracted the IPD from these curves.
One-stage meta-analysis
We used the Cox proportional hazards model for our main analysis of OS and DFS since we did not detect any violation of the proportionality-of-hazards assumption by visualizing scaled Schoenfeld residuals, log-log survival plots, and predicted versus observed survival curves (Figures S1-S6).
OS
The pooled OS curve of all patients undergoing RATS versus VATS is presented in Figure 2. The OS at 1-, 5- and 10-year of follow up in the RATS group was 95.5%, 71.8% and 64.5%, respectively and the median follow-up time was 51.6 months [interquartile range (IQR): 26.0–59.6]. Regarding the VATS approach the OS at 1-, 5- and 10-year of follow up was 94.8%, 73.6% and 58.6%, respectively and the median follow-up time was 51.8 months (IQR: 28.5–59.6). Our survival analysis revealed that RATS and VATS are comparable regarding long-term OS (HR: 1.05, 95% CI: 0.88–1.27, P=0.538).
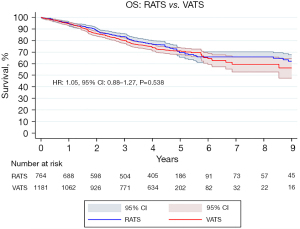
DFS
The pooled DFS curve of all patients undergoing RATS versus VATS is presented in Figure 3. The DFS at 1-, 5- and 10-year of follow up in the RATS group was 88.7%, 58.9% and 47.3%, respectively. Concerning the VATS approach the DFS at 1-, 5- and 10-year of follow up was 88.1%, 58.0% and 34.2%, respectively. Our analysis demonstrated that RATS and VATS are comparable regarding long-term DFS (HR: 1.07, 95% CI: 0.92–1.25, P=0.371).
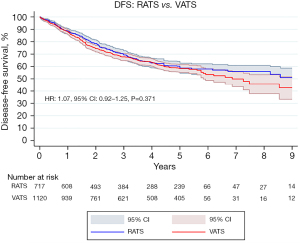
Two-stage meta-analysis
In the cumulative two-stage meta-analysis, the RATS group had equivalent hazard for long-term mortality (HR: 1.27, 95% CI: 0.85–1.90, P=0.24, I2=68.50%) compared to the VATS group verifying our one-stage meta-analysis findings (Figure 4A). Regarding DFS, two-stage meta-analysis once again revealed no statistically significant difference between the two groups (HR: 1.05, 95% CI: 0.85–1.30, P=0.67, I2=28.27%) (Figure 4B). In order to further increase the robustness of our findings we performed a leave-one-out sensitivity analysis and meta-regression analyses for both OS and DFS (Figures S7-S22). None of the performed analyses yielded a statistically significant result.
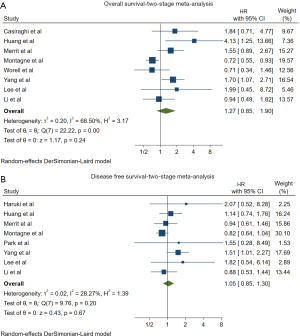
Secondary outcomes
Both VATS and RATS were found to have equivalent outcomes in terms of postoperative complications (OR: 1.07, 95% CI: 0.72–1.58, P=0.75, I2=55.35%), prolonged air leak rates (OR: 1.64, 95% CI: 0.90–2.98, P=0.11, I2=21.56%), conversion to open thoracotomy rates (OR: 1.25, 95% CI: 0.52–3.00, P=0.62, I2=61.12%) (Figures S23-S25). In terms of operative time, no statistically significant difference was observed between the two groups (SMD: 0.16, 95% CI: −0.58, 0.91, P=0.67, I2=96.77%) (Figure S26). RATS was associated with significantly shorter length of hospital stay compared to VATS (SMD: −0.42, 95% CI: −0.79, −0.06, P=0.02, I2=89.77%) (Figure 5A). Finally, RATS was also associated with higher number of intraoperative dissected lymph nodes compared to VATS (SMD: 0.63, 95% CI: 0.18, 1.09, P=0.01, I2=90.95%) (Figure 5B).

All the results of our study are summarized in Table 2.
Table 2
Analyses | HR/OR/SMD (95% CI) | P | Heterogeneity | |
---|---|---|---|---|
I2, % | P | |||
One-stage analysis | ||||
OS | 1.05† (0.88, 1.27) | 0.538 | N/A | N/A |
DFS | 1.07† (0.92, 1.25) | 0.371 | N/A | N/A |
Two-stage analysis | ||||
OS | 1.27† (0.85, 1.90) | 0.24 | 68.5 | <0.01 |
DFS | 1.05 (0.85, 1.30) | 0.67 | 28.3 | 0.20 |
Categorical outcomes | ||||
Postoperative complications | 1.07‡ (0.72, 1.58) | 0.75 | 55.4 | 0.03 |
Prolonged air leak | 1.64‡ (0.90, 2.98) | 0.11 | 21.6 | 0.27 |
Conversion to open thoracotomy | 1.25‡ (0.52, 3.00) | 0.62 | 61.1 | 0.04 |
Continuous outcomes | ||||
Operative time | 0.16§ (−0.58, 0.91) | 0.67 | 97.0 | <0.01 |
Length of hospital stay | −0.42§ (−0.79, −0.06) | 0.02 | 89.8 | <0.01 |
Number of dissected lymph nodes | 0.63§ (0.18, 1.09) | 0.01 | 90.1 | <0.01 |
†, HR; ‡, OR; §, SMD. HR, hazard ratio; OR, odds ration; SMD, standardized mean difference; CI, confidence interval; OS, overall survival; N/A, not applicable; DFS, disease-free survival.
Publication bias assessment
All studies were subjected to quality assessment through the ROBINS-I. Detailed ROBINS-I quality assessment for each of the eligible studies is shown in Figure S27.
Egger’s test was performed in all outcomes that were reported in over eight of the included studies and revealed publication bias in the funnel plots regarding two-stage OS and DFS analysis but no publication bias in the funnel plot regarding postoperative complications (Figures S28-S30). Heterogeneity was not significant in any of the two stage analyses performed nor the secondary outcomes’ meta-analyses except for the operative time, operative time and number of intraoperative dissected lymph nodes were considerable heterogeneity was observed.
Discussion
The findings of this systematic review and meta-analysis suggest that RATS and VATS are equivalent in terms of OS and DFS for early-stage NSCLC inclusive of Stage I–III, and these findings were consistent in both the one-stage and two-stage sensitivity analysis. In addition, our meta-analyses revealed that RATS and VATS are also equivalent in terms of postoperative complications, prolonged air leak, conversion to open thoracotomy, and intraoperative times when performing a lobectomy for NSCLC. RATS was associated with shorter length of hospital stay as well as increased intraoperative number of dissected lymph nodes.
Although our findings with regard to OS are in congruence with prior meta-analyses, our results in terms of DFS are not, and we believe that this discrepancy is attributed to the different inclusion criteria and statistical methods we used to compose our evidence (42-45). First, the most contemporary study identified a total of 11,247 patients undergoing VATS or RATS for NSCLC and reported that RATS offers a better 5-year DFS, however some of the included studies were found to have overlapping populations, meaning that some patients were included redundantly two or three times in the final analyses (15,16,39,46). Another contemporary meta-analysis supporting the superiority of RATS in terms of DFS (43), in addition to exhibiting the same population overlap bias, may have incorporated patients with both benign and malignant lung lesions, increasing the risk of confounding bias on their results (47). The meta-analysis by Ma et al. reported results that differ from our study, i.e., that RATS and VATS have equivalent OS and DFS outcomes, however, studies with overlapping populations were also noted and several studies that we identified in our search were not included in this study (44). Moreover, all prior meta-analyses have pooled studies and patients undergoing lobectomy and segmentectomy under the same analyses, which have potentially increased the risk of bias in their findings (46,48). A summary of the findings of all the prior meta-analyses in our topic is presented in Table S4.
We opted to exclude the studies utilizing large databases not only to avoid the population overlap bias with the institutional reports, but also to avoid the biases that are inherently associated with performing a meta-analysis using data from database studies. In addition, both the National Cancer Database (NCDB) and the Surveillance, Epidemiology, and End Results (SEER) databases do not capture cancer recurrence rates and therefore cannot be used to assess the DFS outcomes. Regarding the statistical models used, in our study instead of comparing study-level effect estimates, we opted for a more granular strategy and analyzed reconstructed individual patient-level data. This offered us the opportunity to perform our analyses with mathematically robust and flexible survival models that we believe offer the best available evidence on the long-term outcomes following RATS versus VATS lobectomy to date (17).
The current literature is also conflicting on short-term outcomes when comparing RATS versus VATS (42-45). Most studies, in congruence with our findings, suggest that the number of dissected lymph nodes and length of stay are superior following RATS compared to VATS (44,45). This finding might be attributed to fact that part of the RATS operation arguably necessitates a better lymph node dissection to perform an anatomic lung resection. The fact that RATS is associated with a better lymph node harvest may potentially lead to an improved rate of upstaging of occult nodal disease. It is worth noting that inadequate lymph node sampling, defined as less than 10 lymph nodes according to the Commission on Cancer-defined quality measures, is observed in ~70% of NSCLC and it was significantly associated with worse OS (49,50). Contemporary reports suggest that RATS is equivalent to open thoracotomy and significantly better than VATS in terms of lymph node dissection and pathologic nodal upstaging (51-53). One might infer this would contribute to improved OS and DFS due to more complete pathologic staging and therefore provision of adjuvant therapy, although this notion is not supported by the data in our manuscript or the literature thus far (54,55). Unfortunately, the disease upstaging rates were reported in only four of our included studies and thus further analyses on this topic were deferred (33,37,38,40).
Of note, the aforementioned benefits of RATS seem to come at an expense with longer operative times and higher total operative costs (56,57). Operative costs, however, may be offset by shorter length of stay (58). Interestingly, the LOS was noted to be shorter in the RATS group despite similar post-operative complications. We hypothesize that this difference might be to the fact that the institutions while adopting the new robotic technologies might have also adopted different discharge protocols including ERAS that facilitated earlier discharge in patients undergoing RATS. Another potential explanation regarding the LOS could be that hospitals utilizing RATS may attempt to offset the surgical costs with the hospitalization costs. Finally, it is worth noting that even though former studies have suggested that RATS offers lower early mortality rates compared to VATS (42), more recent reports demonstrate that both RATS and VATS are equivalent in terms of early mortality and complications (27,44,45). In addition, in contrast to our findings, prior reports have suggested that RATS is associated with lower conversion rates to open thoracotomy (42,44,45). Overall, we reason that all the discrepancies between the individual studies regarding the intra- and post- operative morbidity and mortality might imply that the surgeons’ or centers’ experience utilizing each approach could significantly influence the outcomes following RATS or VATS lobectomies.
Although, pulmonary lung resections were traditionally performed via an open thoracotomy, the emergence of minimally invasive thoracic approaches in the recent era has led to a paradigm swift. In fact, minimally invasive thoracic surgery has taken over the traditional open thoracotomy and has become standard in many centers over the last years (3,9). Contemporary studies outline that currently VATS is more commonly utilized than open thoracotomy and interestingly the number of RATS operations has increased from 1% of total lobectomy volumes in 2008 to ~20% in the year 2014, and from 20% in 2015 to 34% in 2018 (3,9,59,60). Regardless of approach, minimally invasive pulmonary resection is the gold standard for operable NSCLC and quality long-term data is paramount to help guide decision making and provision of high-quality surgical care to our patients.
There are several limitations in this study that should be acknowledged. First, there are inherent limitations to a study-level meta-analysis as we did not have access to patient-level covariates which could confound our findings. As such no sensitivity analyses examining the impact of certain variables on OS and/or DFS could be synthesized besides meta-regression analyses. It should be clarified that all the operations reported in our study were performed by thoracic surgeon and for that reason our findings may not be generalizable to surgeons who have had different training backgrounds and this might explain the significant heterogeneity noted in some of our results. In addition, all of the included studies were retrospective cohorts and thus impart high risk for confounding biases. It should also be acknowledged that our results regarding the OS, LOS and number of dissected lymph nodes displayed substantial heterogeneity and therefore should be interpreted with cation. Moreover, all the procedures in the included studies were performed in different centers by different operators. This fact, along with differences in the follow-up schemes across the studies, might have affected the external validity of our results.
Conclusions
Accounting for the conflicting evidence in terms of early outcomes and for our findings indicating that RATS associated with shorter LOS and improved lymph node dissection and that both RATS and VATS are comparable regarding the long-term OS and DFS in patients undergoing lobectomy for NSCLC, we conclude that the utilization of RATS over VATS should be tailored according to the surgeon’s and the center’s experience and requires individualized patient selection considering each patient’s personal preferences. Future research should aim to reconcile conflicting short-term outcomes, optimize postoperative care, explore the impact of lymph node dissection, and assess the role of surgeon training on outcomes. Incorporating patient-centered outcomes, such as patient satisfaction, quality of life, and functional status, in future analyses would add another dimension to the comparison of RATS and VATS. By addressing these areas, surgical techniques can be refined and patient outcomes can be improved.
Acknowledgments
Funding: None.
Footnote
Reporting Checklist: The authors have completed the PRISMA reporting checklist. Available at https://jtd.amegroups.com/article/view/10.21037/jtd-23-582/rc
Peer Review File: Available at https://jtd.amegroups.com/article/view/10.21037/jtd-23-582/prf
Conflicts of Interest: All authors have completed the ICMJE uniform disclosure form (available at https://jtd.amegroups.com/article/view/10.21037/jtd-23-582/coif). The authors have no conflicts of interest to declare.
Ethical Statement: The authors are accountable for all aspects of the work in ensuring that questions related to the accuracy or integrity of any part of the work are appropriately investigated and resolved.
Open Access Statement: This is an Open Access article distributed in accordance with the Creative Commons Attribution-NonCommercial-NoDerivs 4.0 International License (CC BY-NC-ND 4.0), which permits the non-commercial replication and distribution of the article with the strict proviso that no changes or edits are made and the original work is properly cited (including links to both the formal publication through the relevant DOI and the license). See: https://creativecommons.org/licenses/by-nc-nd/4.0/.
References
- NCCN Clinical Practice Guidelines in Oncology. Non-Small Cell Lung Cancer. Version 1.2023. Available online: https://www.nccn.org/guidelines/recently-published-guidelines
- Wong MKH, Sit AKY, Au TWK. Minimally invasive thoracic surgery: beyond surgical access. J Thorac Dis 2018;10:S1884-91. [Crossref] [PubMed]
- Alwatari Y, Khoraki J, Wolfe LG, et al. Trends of utilization and perioperative outcomes of robotic and video-assisted thoracoscopic surgery in patients with lung cancer undergoing minimally invasive resection in the United States. JTCVS Open 2022;12:385-98. [Crossref] [PubMed]
- Mangiameli G, Cioffi U, Testori A. Lung Cancer Treatment: From Tradition to Innovation. Front Oncol 2022;12:858242. [Crossref] [PubMed]
- Coco D, Leanza S. Current perspective on uniportal and multiportal video-assisted thoracic surgery during lobectomy for lung cancer. Kardiochir Torakochirurgia Pol 2022;19:146-51. [Crossref] [PubMed]
- Kirby TJ, Mack MJ, Landreneau RJ, et al. Initial experience with video-assisted thoracoscopic lobectomy. Ann Thorac Surg 1993;56:1248-52; discussion 1252-3. [Crossref] [PubMed]
- Swanson SJ, Herndon JE 2nd, D'Amico TA, et al. Video-assisted thoracic surgery lobectomy: report of CALGB 39802--a prospective, multi-institution feasibility study. J Clin Oncol 2007;25:4993-7. [Crossref] [PubMed]
- Melfi FM, Menconi GF, Mariani AM, et al. Early experience with robotic technology for thoracoscopic surgery. Eur J Cardiothorac Surg 2002;21:864-8. [Crossref] [PubMed]
- Subramanian MP, Liu J, Chapman WC Jr, et al. Utilization Trends, Outcomes, and Cost in Minimally Invasive Lobectomy. Ann Thorac Surg 2019;108:1648-55. [Crossref] [PubMed]
- Yun J, Lee J, Shin S, et al. Video-assisted thoracoscopic lobectomy versus open lobectomy in the treatment of large lung cancer: propensity-score matched analysis. J Cardiothorac Surg 2022;17:2. [Crossref] [PubMed]
- Raman V, Christopher JK, Jawitz OK, et al. Robot- vs Video-Assisted Thoracoscopic Lobectomy for Early Lung Cancer. JNCI Cancer Spectr 2020;4:pkaa031. [Crossref] [PubMed]
- Jin R, Zheng Y, Yuan Y, et al. Robotic-assisted Versus Video-assisted Thoracoscopic Lobectomy: Short-term Results of a Randomized Clinical Trial (RVlob Trial). Ann Surg 2022;275:295-302. [Crossref] [PubMed]
- Reddy RM, Gorrepati ML, Oh DS, et al. Robotic-Assisted Versus Thoracoscopic Lobectomy Outcomes From High-Volume Thoracic Surgeons. Ann Thorac Surg 2018;106:902-8. [Crossref] [PubMed]
- Patel YS, Hanna WC, Fahim C, et al. RAVAL trial: Protocol of an international, multi-centered, blinded, randomized controlled trial comparing robotic-assisted versus video-assisted lobectomy for early-stage lung cancer. PLoS One 2022;17:e0261767. [Crossref] [PubMed]
- Sesti J, Langan RC, Bell J, et al. A Comparative Analysis of Long-Term Survival of Robotic Versus Thoracoscopic Lobectomy. Ann Thorac Surg 2020;110:1139-46. [Crossref] [PubMed]
- Hennon MW, DeGraaff LH, Groman A, et al. The association of nodal upstaging with surgical approach and its impact on long-term survival after resection of non-small-cell lung cancer. Eur J Cardiothorac Surg 2020;57:888-95. [Crossref] [PubMed]
- Riley RD, Lambert PC, Abo-Zaid G. Meta-analysis of individual participant data: rationale, conduct, and reporting. BMJ 2010;340:c221. [Crossref] [PubMed]
- Page MJ, McKenzie JE, Bossuyt PM, et al. The PRISMA 2020 statement: an updated guideline for reporting systematic reviews. BMJ 2021;372: [Crossref] [PubMed]
- Association for Computing Machinery. Guidelines for Snowballing in Systematic Literature Studies and a Replication in Software Engineering. London, England: Proceedings of the 18th International Conference on Evaluation and Assessment in Software Engineering; 2014.
- Covidence systematic review software, Veritas Health Innovation, Melbourne, Australia. Available online: www.covidence.org
- Sterne JA, Hernán MA, Reeves BC, et al. ROBINS-I: a tool for assessing risk of bias in non-randomised studies of interventions. BMJ 2016;355:i4919. [Crossref] [PubMed]
- Hozo SP, Djulbegovic B, Hozo I. Estimating the mean and variance from the median, range, and the size of a sample. BMC Med Res Methodol 2005;5:13. [Crossref] [PubMed]
- Wan X, Wang W, Liu J, et al. Estimating the sample mean and standard deviation from the sample size, median, range and/or interquartile range. BMC Med Res Methodol 2014;14:135. [Crossref] [PubMed]
- Wei Y, Royston P. Reconstructing time-to-event data from published Kaplan-Meier curves. Stata J 2017;17:786-802.
- Guyot P, Ades AE, Ouwens MJ, et al. Enhanced secondary analysis of survival data: reconstructing the data from published Kaplan-Meier survival curves. BMC Med Res Methodol 2012;12:9. [Crossref] [PubMed]
- DerSimonian R, Laird N. Meta-analysis in clinical trials. Control Clin Trials 1986;7:177-88. [Crossref] [PubMed]
- Shagabayeva L, Fu B, Panda N, et al. Open, Video- and Robot-Assisted Thoracoscopic Lobectomy for Stage II-IIIA Non-Small Cell Lung Cancer. Ann Thorac Surg 2023;115:184-90. [Crossref] [PubMed]
- Cui Y, Grogan EL, Deppen SA, et al. Mortality for Robotic- vs Video-Assisted Lobectomy-Treated Stage I Non-Small Cell Lung Cancer Patients. JNCI Cancer Spectr 2020;4:pkaa028. [Crossref] [PubMed]
- Kneuertz PJ, D'Souza DM, Richardson M, et al. Long-Term Oncologic Outcomes After Robotic Lobectomy for Early-stage Non-Small-cell Lung Cancer Versus Video-assisted Thoracoscopic and Open Thoracotomy Approach. Clin Lung Cancer 2020;21:214-224.e2. [Crossref] [PubMed]
- Nelson DB, Mehran RJ, Mitchell KG, et al. Robotic-Assisted Lobectomy for Non-Small Cell Lung Cancer: A Comprehensive Institutional Experience. Ann Thorac Surg 2019;108:370-6. [Crossref] [PubMed]
- Veluswamy RR, Whittaker Brown SA, Mhango G, et al. Comparative Effectiveness of Robotic-Assisted Surgery for Resectable Lung Cancer in Older Patients. Chest 2020;157:1313-21. [Crossref] [PubMed]
- Montagne F, Chaari Z, Bottet B, et al. Long-Term Survival Following Minimally Invasive Lung Cancer Surgery: Comparing Robotic-Assisted and Video-Assisted Surgery. Cancers (Basel) 2022;14:2611. [Crossref] [PubMed]
- Casiraghi M, Mariolo AV, Mohamed S, et al. Long-Term Outcomes of Robotic-Assisted, Video-Assisted and Open Surgery in Non-Small Cell Lung Cancer: A Matched Analysis. J Clin Med 2022;11:3363. [Crossref] [PubMed]
- Yang HX, Woo KM, Sima CS, et al. Long-term Survival Based on the Surgical Approach to Lobectomy For Clinical Stage I Nonsmall Cell Lung Cancer: Comparison of Robotic, Video-assisted Thoracic Surgery, and Thoracotomy Lobectomy. Ann Surg 2017;265:431-7. [Crossref] [PubMed]
- Worrell SG, Dedhia P, Gilbert C, et al. The cost and quality of life outcomes in developing a robotic lobectomy program. J Robot Surg 2019;13:239-43. [Crossref] [PubMed]
- Park SY, Suh JW, Narm KS, et al. Feasibility of four-arm robotic lobectomy as solo surgery in patients with clinical stage I lung cancer. J Thorac Dis 2017;9:1607-14. [Crossref] [PubMed]
- Merritt RE, Abdel-Rasoul M, D'Souza DM, et al. Comparison of the long-term oncologic outcomes of robotic-assisted and video-assisted thoracoscopic lobectomy for resectable non-small cell lung carcinoma. J Robot Surg 2022;16:1281-8. [Crossref] [PubMed]
- Huang L, Shen Y, Onaitis M. Comparative study of anatomic lung resection by robotic vs. video-assisted thoracoscopic surgery. J Thorac Dis 2019;11:1243-50. [Crossref] [PubMed]
- Li C, Hu Y, Huang J, et al. Comparison of robotic-assisted lobectomy with video-assisted thoracic surgery for stage IIB-IIIA non-small cell lung cancer. Transl Lung Cancer Res 2019;8:820-8. [Crossref] [PubMed]
- Lee BE, Shapiro M, Rutledge JR, et al. Nodal Upstaging in Robotic and Video Assisted Thoracic Surgery Lobectomy for Clinical N0 Lung Cancer. Ann Thorac Surg 2015;100:229-33; discussion 233-4. [Crossref] [PubMed]
- Haruki T, Kubouchi Y, Takagi Y, et al. Comparison of medium-term survival outcomes between robot-assisted thoracoscopic surgery and video-assisted thoracoscopic surgery in treating primary lung cancer. Gen Thorac Cardiovasc Surg 2020;68:984-92. [Crossref] [PubMed]
- Liang H, Liang W, Zhao L, et al. Robotic Versus Video-assisted Lobectomy/Segmentectomy for Lung Cancer: A Meta-analysis. Ann Surg 2018;268:254-9. [Crossref] [PubMed]
- Wu H, Jin R, Yang S, et al. Long-term and short-term outcomes of robot- versus video-assisted anatomic lung resection in lung cancer: a systematic review and meta-analysis. Eur J Cardiothorac Surg 2021;59:732-40. [Crossref] [PubMed]
- Ma J, Li X, Zhao S, et al. Robot-assisted thoracic surgery versus video-assisted thoracic surgery for lung lobectomy or segmentectomy in patients with non-small cell lung cancer: a meta-analysis. BMC Cancer 2021;21:498. [Crossref] [PubMed]
- Zhang J, Feng Q, Huang Y, et al. Updated Evaluation of Robotic- and Video-Assisted Thoracoscopic Lobectomy or Segmentectomy for Lung Cancer: A Systematic Review and Meta-Analysis. Front Oncol 2022;12:853530. [Crossref] [PubMed]
- Zhou Q, Huang J, Pan F, et al. Operative outcomes and long-term survival of robotic-assisted segmentectomy for stage IA lung cancer compared with video-assisted thoracoscopic segmentectomy. Transl Lung Cancer Res 2020;9:306-15. [Crossref] [PubMed]
- Demir A, Ayalp K, Ozkan B, et al. Robotic and video-assisted thoracic surgery lung segmentectomy for malignant and benign lesions. Interact Cardiovasc Thorac Surg 2015;20:304-9. [Crossref] [PubMed]
- Zhang Y, Chen C, Hu J, et al. Early outcomes of robotic versus thoracoscopic segmentectomy for early-stage lung cancer: A multi-institutional propensity score-matched analysis. J Thorac Cardiovasc Surg 2020;160:1363-72. [Crossref] [PubMed]
- Odell DD, Feinglass J, Engelhardt K, et al. Evaluation of adherence to the Commission on Cancer lung cancer quality measures. J Thorac Cardiovasc Surg 2019;157:1219-35. [Crossref] [PubMed]
-
. Available online: https://www.facs.org/quality-programs/cancer-programs/cancer-surgery-standards-program/Cancer Surgery Standards Program - Tang A, Raja S, Bribriesco AC, et al. Robotic Approach Offers Similar Nodal Upstaging to Open Lobectomy for Clinical Stage I Non-small Cell Lung Cancer. Ann Thorac Surg 2020;110:424-33. [Crossref] [PubMed]
- Zirafa C, Aprile V, Ricciardi S, et al. Nodal upstaging evaluation in NSCLC patients treated by robotic lobectomy. Surg Endosc 2019;33:153-8. [Crossref] [PubMed]
- Kneuertz PJ, Cheufou DH, D'Souza DM, et al. Propensity-score adjusted comparison of pathologic nodal upstaging by robotic, video-assisted thoracoscopic, and open lobectomy for non-small cell lung cancer. J Thorac Cardiovasc Surg 2019;158:1457-1466.e2. [Crossref] [PubMed]
- Wilson JL, Louie BE, Cerfolio RJ, et al. The prevalence of nodal upstaging during robotic lung resection in early stage non-small cell lung cancer. Ann Thorac Surg 2014;97:1901-6; discussion 1906-7. [Crossref] [PubMed]
- Toosi K, Velez-Cubian FO, Glover J, et al. Upstaging and survival after robotic-assisted thoracoscopic lobectomy for non-small cell lung cancer. Surgery 2016;160:1211-8. [Crossref] [PubMed]
- Keeney-Bonthrone TP, Frydrych LM, Karmakar M, et al. Robot-assisted vs. video-assisted thoracoscopic lobectomy: a systematic review of cost effectiveness. Video-assist Thorac Surg 2020;5:4.
- Singer E, Kneuertz PJ, D'Souza DM, et al. Understanding the financial cost of robotic lobectomy: calculating the value of innovation?. Ann Cardiothorac Surg 2019;8:194-201. [Crossref] [PubMed]
- Shanahan B, Kreaden US, Sorensen J, et al. Is robotic lobectomy cheaper? A micro-cost analysis. J Robot Surg 2022;16:1441-50. [Crossref] [PubMed]
- Dezube AR, Hirji S, Shah R, et al. Pre-COVID-19 National Mortality Trends in Open and Video-Assisted Lobectomy for Non-Small Cell Lung Cancer. J Surg Res 2022;274:213-23. [Crossref] [PubMed]
- Napolitano MA, Sparks AD, Werba G, et al. Video-Assisted Thoracoscopic Surgery Lung Resection in United States Veterans: Trends and Outcomes versus Thoracotomy. Thorac Cardiovasc Surg 2022;70:346-54. [Crossref] [PubMed]