Neutrophil bactericidal activity and host defenses in cystic fibrosis: a narrative review
Introduction
Cystic fibrosis (CF) is an autosomal recessive disorder that causes damage to the lungs, digestive system, and other organs. It is a result of a mutation in the cystic fibrosis transmembrane conductance regulator (CFTR), a cyclic adenosine monophosphate stimulated chloride channel, gene located on chromosome seven and affects the transport of Cl ions (Cl−) across epithelial membranes. The most common type of mutation is a Phe508del mutation, but there are hundreds of other mutations (e.g., Gly551Asp). By allowing Cl− to move across the membrane, water and salt (NaCl) remain in balance, thereby lubricating the mucosal epithelium. However, in CF, Cl− is trapped in the cell, and this disrupts the osmotic balance and transforms the mucus from a well-hydrated form to a dehydrated form that reduces the function of the mucociliary apparatus (1). The viscous mucus accumulates in the bronchi, and this leads to damage, obstruction, and infections.
Neutrophils are granulocytic cells of the innate immune system that constitute 60% to 70% of peripheral blood leukocytes (2). Their primary function is to trap and kill pathogens by phagocytosis and subsequent degranulation of toxic enzymes contained in lysosomes (Figure 1) (3,4). Several granules (primary, secondary, and tertiary) that contain different enzymes are involved in this process. Phagocytosis engulfs the pathogen into the cell interior and forms a phagosome. The phagosomes then fuse with lysosomes to form phagolysosomes, and this initiates pathogen killing. Neutrophils use both oxygen-dependent and oxygen-independent processes to destroy pathogens. The oxygen-dependent pathway is termed “oxidative burst” and requires the enzyme nicotinamide adenine dinucleotide phosphate (NADPH) oxidase. This enzyme converts oxygen (O2) to superoxide (Step 1). Superoxide is then converted to hydrogen peroxide (H2O2) by superoxide dismutase (Step 2). Myeloperoxidase (MPO) adds a chloride ion to the H2O2 to form hypochlorous acid (HOCl) that destroys the pathogen (Step 3) (Figure 2) (5).
- Step 1. O2 + NADPH → O2− + NADP+
- Step 2. O2− → H2O2
- Step 3. H2O2 + Cl− → HOCl

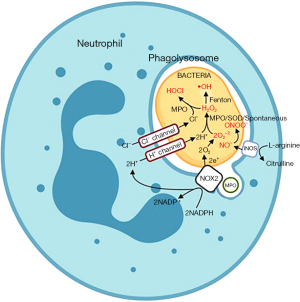
Patients with CF have increased susceptibility to respiratory tract infections from microbes, such as Staphylococcus aureus (S. aureus), Haemophilus influenzae, and Pseudomonas aeruginosa (P. aeruginosa) (6). Because of the thick mucus and the reduced periciliary fluid layer, the cilia in the lungs can no longer beat normally, the infection cannot be cleared mechanically, and this allows the bacteria to establish a state of chronic infection (7). The innate immune system responds by increasing pro-inflammatory cytokines, such interleukin-1, tumor necrosis factor (TNF), interleukin-6, and interleukin-8. These cytokines recruit neutrophils to the lungs to clear the pathogens, but these neutrophils have reduced bactericidal activity (8). Neutrophils persist in respiratory secretions and release toxic molecules, enzymes, and extracellular traps that can injure host tissue and can potentially lead to cell death; bacteria also persist in the lung and can cause both direct injury through the release of enzymes and indirect injury to the lung by eliciting increased host defense responses (8,9).
CFTR modulators, such as elexacaftor, tezacaftor, and ivacaftor, are relatively new drugs used in the treatment of CF. These medications modify the CFTR transmembrane protein to facilitate adequate flux of Cl− out of the cell (10). Ivacaftor, in particular, is a potentiator of the CFTR protein that modifies the protein to remain open; the other drugs help the protein fold into the right three-dimensional (3D) conformation (11). Both mechanisms of action increase the production of normal epithelial secretions and increase the clearance of pathogens from the lungs.
The reduced bacterial clearance in CF airways may reflect abnormal mucociliary function resulting in chronic inflammation and airway damage. Neutrophils recruited into these airways may not have normal bactericidal activity secondary to the complex environment, which includes biofilms. Finally, it is possible that the CFTR mutations also affect neutrophils and reduce their bactericidal activity.
The key questions in this review include: (I) do CFTR mutations alter neutrophil function? (II) Do CFTR modulator medications improve neutrophil function? We present this article in accordance with the Narrative Review reporting checklist (available at https://jtd.amegroups.com/article/view/10.21037/jtd-23-846/rc).
Methods
The PubMed database was initially searched on February 10, 2023; an expanded search was repeated on July 23, 2023 to compile a more comprehensive list of clinical and experimental studies. Table 1 highlights the second search conducted using the combination of the MeSH terms: “cystic fibrosis”, and “Neutrophils/physiology”. Table 2 summarizes a second search using the MeSH terms: “Cystic fibrosis transmembrane conductance regulator” and “neutrophils”.
Table 1
Items | Specification |
---|---|
Date of search | 02/10/2023 & 07/23/2023 |
Databases and other sources searched | PubMed |
Search terms used | Search terms: “Cystic Fibrosis [MeSH]” AND “Neutrophils/physiology [MAJR]”. Filters: date range set from 1990 to 2023; English |
Timeframe | 1990 to 2023 |
Inclusion and exclusion criteria | Inclusion criteria: experimental design; English language; investigates the physiology underlying defective neutrophils. |
Exclusion criteria: literature reviews; case studies; discuss only the role of neutrophils in cystic fibrosis without mentioning possible dysfunction; does not investigate a defect in neutrophils | |
Selection process | The selection process was conducted independently by the authors and was approved by a board-certified pulmonologist. The search revealed 195 articles each of which were carefully reviewed per the inclusion and exclusion criteria. This yielded 27 articles that were then limited to those discussing the oxidative burst pathway and other microbiocidal activity |
Table 2
Items | Specification |
---|---|
Date of search | 02/10/2023 & 07/23/2023 |
Databases and other sources searched | PubMed |
Search terms used | Search terms: “Cystic Fibrosis Transmembrane Conductance Regulator [MeSH]” and “Neutrophils [MeSH]”. Filters: English |
Timeframe | 1990 to 2023 |
Inclusion and exclusion criteria | Inclusion criteria: experimental design; discussed CFTR and its relationship with neutrophils; mentioned the effects of therapeutics on CFTR and neutrophils. |
Exclusion criteria: literature reviews; case studies; only mentioned CFTR and neutrophils without investigating the connection between the two | |
Selection process | The selection process was conducted independently by the authors and was approved by a board-certified pulmonologist. The search produced 92 articles that were examined according to the inclusion and exclusion criteria; this reduced the total to 25 articles. Then, we narrowed to the list to those that discussed the specific CFTR modulator therapy |
CFTR, cystic fibrosis transmembrane conductance regulator.
Discussion
CF neutrophils
Most studies with neutrophils isolated from CF patients use in vitro assays that allow measurement of the neutrophil functions or steps needed for bactericidal activity (Figure 1).
Painter et al. hypothesized that neutrophils from CF patients may have limited transfer of Cl− into phagolysosomes and that this reduces chlorination (i.e., the formation of HOCl) and killing of ingested bacteria (Figure 2) (12). After isolating neutrophils from peripheral blood using the Percoll method, conducting CFTR and MPO immunofluorescence studies to identify proteins, inducing neutrophil ingestion of green fluorescent labeled P. aeruginosa (PA01) performing RNA reverse transcription-PCR, and analyzing Western blots, these investigators determined that CFTR was expressed on phagolysosome membranes post phagocytosis and that CFTR potentially had an important role in neutrophil function. Neutrophil chlorination activity in CF patients was then investigated. 13C9-L-tyrosine labeled serum-opsonized PAO1 was introduced into normal and CF neutrophils. Gas chromatography and mass spectrometry were then used to detect the PAO1-derived chlorotyrosine and tyrosine. Compared to the normal neutrophils, CF neutrophils had a significantly lower than normal ratio of PAO1-derived chlorotyrosine to PAO1 tyrosine. Normal neutrophils had a ratio of 1.61±0.38; CF neutrophils had a ratio of 0.35±0.23, consistent with a chlorination defect in CF neutrophils. To confirm that there was defective HOCl production in phagolysosomes, phagocytic function and extracellular HOCl formation by CF neutrophils were tested and were similar to control neutrophils. Therefore, this study concluded that CF neutrophils had decreased levels of phagolysosomal chlorination due to decreased transfer of Cl− into vesicles, and this reduces bacterial killing.
Houston et al. studied the sputum specimens and peripheral blood from 31 CF patients (13). The samples were divided into “acute” (collected during the first week of IV antibiotic treatment) and “post-acute” (collected during second week after marked clinical improvement). Isolated neutrophils were labeled with hydroethidine (HE) and 2',7'-dichlorodihydrofluorescein diacetate (DCFH-DA), which are fluorescent markers that selectively bind superoxide radicals and H2O2, respectively. After direct stimulation with phorbol 12-myristate 13-acetate (PMA), NADPH oxidase produced superoxide radicals, which are converted to H2O2 by superoxide dismutase. The samples were then analyzed with flow cytometry and revealed a unimodal graph in sputum neutrophils and a bimodal graph in peripheral blood neutrophils, probably representing some populations with different levels of oxidative activity. In addition, the resting index (RI) and stimulation index (SI) were measured to approximate respiratory burst and to reduce variation between samples. The RI is the ratio of the mean fluorescence intensity of spontaneous reactive oxygen species (ROS) production to unstained background ROS production, and SI is the ratio of stimulated to spontaneous ROS production. Each ratio measures the amount of a specific fluorescent marker (HE or DCFH-DA). While RIHE and RIDCFH-DA showed a slight reduction in sputum neutrophil ROS production, the SIHE and SIDCFH-DA of sputum neutrophils had a more significant decrease. These authors interpreted these results to suggest that the neutrophils had functional exhaustion secondary to residence in regions in the lung with chronic inflammation. Table 3 details the SI and RI results from blood and sputum samples taken at acute or post-acute times.
Table 3
ROS index | Sample type | Acute | Post-acute |
---|---|---|---|
RIHE | Blood | 35.9 | 38.2 |
Sputum | 26.6 | 25.1 | |
SIHE | Blood | 6.8 | 5.9 |
Sputum | 1.1 | 1.0 | |
RIDCFH-DA | Blood | 149 | 123 |
Sputum | 66.2 | 47.1 | |
SIDCFH-DA | Blood | 21.0 | 19.6 |
Sputum | 2.0 | 1.7 |
Blood and sputum samples were collected from CF patients during the acute phase of an exacerbation and during the recovery phase. RI is the resting index, and SI is the stimulated index using markers for superoxide (HE) and for H2O2 (DCFH). The numerical information represents the resting florescence compared to unstained background florescence in the RI assays, and stimulated florescence compared to spontaneous florescence in the SI assays. Adapted with permission from Houston et al. (13). H2O2, hydrogen peroxide; ROS, reactive oxygen species; RI, resting index; HE, hydroethidine (marker for superoxide); SI, stimulated index; DCFH-DA, 2’,7’-dichlorodihydrofluorescein diacetate (marker for hydrogen peroxide); CF, cystic fibrosis.
In 2018, Robledo-Avila et al. published a paper investigating the possible role of calcium in defective antimicrobial responses (14). CFTR dysfunction was first investigated by incubating bacteria and neutrophils from wildtype (WT) and CFTR-deficient mice and neutrophils from non-CF and CF human donors. After lysing the neutrophils and performing serial dilutions, it was determined that CFTR-deficient murine and CF human neutrophils had decreased bactericidal activity. Next, highly reactive oxygen species (hROS), including hypochlorite (ClO−), hydroxyl radical (•OH), and peroxynitrite (ONOO−), were tagged with aminophenyl fluorescein (APF) in non-CF neutrophils, non-CF neutrophils treated with pyrimido-pyrrolo-quinoxalinedione (PPQ-102: a CFTR inhibitor), and CF neutrophils. When stimulated with PMA, the non-CF-PPQ-102 inhibited neutrophils and CF neutrophils had reduced production of hROS. The non-CF-PPQ-102 inhibited and CF neutrophils had an hROS production of approximately 3.8×104 and 4.2×104 relative fluorescent units (RFU), respectively, when stimulated with PMA; normal neutrophils had an average hROS production of 6.0×104 RFU when stimulated with PMA.
Extracellular trap formation (NETosis) by non-CF neutrophils, non-CF-PPQ-102 inhibited neutrophils, and CF neutrophils was studied by stimulation by PMA and fluorescent labeling of the extruded DNA. Compared to non-CF neutrophils, the non-CF plus PPQ-102 inhibitor neutrophils and CF neutrophils produced fewer neutrophil extracellular traps (NETs). Fluo-4 AM is a probe that attaches to calcium to indicate the calcium activity within a neutrophil. Stimulated CFTR-deficient neutrophils from mice had an increase in calcium activity compared to WT controls. To identify the source of the calcium response, the chelating agent, egtazic acid (EGTA), was used to trap extracellular calcium, and the activity was still increased in CFTR-deficient neutrophils. In addition, calcium activity in p47phox−/− neutrophils (i.e., NADPH oxidase deficient) was also increased. Non-CF neutrophils were then incubated with diphenyleneiodonium chloride (DPI), a NADPH oxidase inhibitor, and an increase in calcium activity was noted, and results were similar to those with CF neutrophils. This relative calcium over loading may also contribute to decreased bacterial killing by neutrophils through the inhibition of NET release.
Painter et al. studied the relationship between chloride and CFTR on P. aeruginosa killing by neutrophils (15). Bactericidal activity against P. aeruginosa was dependent on the amount of Cl− in the medium. Normal neutrophils were placed in solutions with varying concentrations of Cl−, and the neutrophils intubated in the Cl− depleted solution had reduced antimicrobial activity (a rate constant of 2.85% per minute); neutrophils incubated in a Cl− rich solution (i.e., physiological level) had increased antimicrobial activity (a rate constant of 6.05% per minute). Moreover, a Cl− rich solution increased NADPH oxidase activity by 15% to 20% compared to the Cl− depleted environment. Extracellular Cl− also affected HOCl production. Using normal neutrophils, aminobenzoic acid hydrazide (ABAH), an MPO inhibitor, treated neutrophils, and fluorescently labeled yeast cell walls (zymosan-A particles), it was demonstrated that the normal cells had decreased fluorescence in an increasing Cl− environment. Conversely, the ABAH-treated neutrophils had no significant fluorescent changes in varying Cl− environments. Moreover, fluorescence had an inverse relationship with Cl− levels; this decreased fluorescence reflects oxidative activity in the cells.
To analyze the specific mechanisms, NADPH oxidase, MPO, and CFTR were inhibited with DPI, ABAH and GlyH-101, respectively, and placed in physiological and depleted Cl− solutions. These modified neutrophils had decreased killing rate constants of ~3.76%, ~3.66%, and ~3.84% per min, respectively, compared to a normal rate of ~5.27 per min in physiological Cl− solutions. In the absence of Cl−, the inhibited neutrophils still had residual killing activity. The normal neutrophils in Cl− depleted environments had a rate constant of ~2.45%; neutrophils inhibited with DPI, ABAH, and GlyH-101 had rate constants of ~2.22%, 2.29%, and 1.95% per min, respectively. These experiments suggested that NADPH oxidase, MPO, CFTR and extracellular chloride all contribute to bactericidal activity of neutrophils, but there was residual bactericidal activity after inhibition. CF neutrophils, in particular, had lower rate constants in Cl− rich (6.55%) and Cl− deficient solutions (2.98%) compared to normal neutrophils (Cl− rich solutions: 8.96%±0.22%; Cl− depleted solutions: 4.91%±0.63%). These results suggest that the decreased chloride concentrations in respiratory secretions could potentially reduce neutrophil bactericidal activity.
Bakalović et al. studied the functional and phenotypic changes in neutrophils in children with CF (16). This study included 19 CF children (ages 5–17 years) and 14 age-matched healthy children. The production of ROS by neutrophils from CF children stimulated with PMA was significantly lower than the production by neutrophils from healthy children. CF neutrophils had a significantly lower rate of spontaneous apoptosis than control neutrophils. The addition of the P. aeruginosa to the cultures of CF neutrophils further decreased the apoptosis rate in comparison to unstimulated cultures. Patients with moderate to severe CF had a higher rate of stimulated NET production. Neutrophils from CF patients had similar rates of Pseudomonas phagocytosis and killing when compared to neutrophils from healthy control subjects but had reduced neutrophil elastase release. These studies indicate that neutrophils from CF patients have differences in function that could reduce bacterial killing (reduced ROS production, reduced elastase release) and increase lung injury (delayed apoptosis).
Kelk et al. isolated neutrophils from both children and adults with CF. These neutrophils were stimulated with PMA, and the amount and rate of ROS were measured (17). Some of the patients were hospitalized with acute exacerbation of CF. The rate of ROS production was lower in patients. There was no increase during exacerbation nor a decrease during clinical improvement. These authors suggest that these results do not support a role for exaggerated respiratory burst activity contributing to neutrophil associated inflammation in CF lung disease. Alternatively, the reduced rate of ROS production might suggest abnormal host defense responses, which contributes to delayed clearance of infections.
In summary, these experiments demonstrate that CFTR channel function has an important role in bacterial killing by neutrophils. These channels transfer chloride into phagolysosomes, which allows the production of ROS, which in turn kill the bacteria. In addition, chloride concentrations in respiratory secretions likely influence bacterial killing in this inflammatory environment. However, proteolytic enzymes in phagolysosomes also contribute to bacterial killing.
Ivacaftor and CF neutrophil function using in vitro assays
CFTR modulator drugs have important effects in patients with CF (18). Ivacaftor is an oral CFTR potentiator that improves the function of the CFTR protein and improves mucus quality in vivo. These drugs slow the decline in pulmonary function, reduce the exacerbation rate, and improve the quality of life, based on questionnaires. Several studies have evaluated the effect of ivacaftor on neutrophil function (Table 4); results from four of the most pertinent studies are detailed below.
Table 4
Author, year (reference) | Drug and protocol | Drug effect |
---|---|---|
Zhou et al., 2013 (19) | Neutrophils and experimental cell line with CF mutation; CFTR corrector compound VRT-325 | CFTR transferred to phagosomes during particle ingestion |
Pohl et al., 2014 (20) | Patients treated with ivacaftor; in vitro studies with neutrophil | Corrected Rab27a activation and increased degranulation of secondary granules and bacterial killing |
Bratcher et al., 2016 (21) | Patients treated with ivacaftor; in vitro studies of neutrophil | Decreased cell surface markers on CF neutrophils to normal levels and decreased inflammatory response |
White et al., 2017 (22) | Patients treated with ivacaftor; in vitro studies of neutrophil | Increased cholesterol in neutrophil membranes, decreased cytosolic Ca2+ levels |
Guerra et al., 2017 (23) | Patients treated with ivacaftor; in vitro studies of neutrophil | Increased respiratory burst in neutrophil |
Hayes et al., 2020 (24) | Patients treated with ivacaftor; in vitro studies on neutrophil from HC and CF patients | Corrected the dysregulated degranulation of CF neutrophils and decreased the pH in phagolysosomes |
Hardisty et al., 2021 (25) | Patients treated with ivacaftor; in vitro studies of neutrophil | Altered neutrophil phenotype and decreased inflammatory and activation markers |
CFTR, cystic fibrosis transmembrane conductance regulator; CF, cystic fibrosis; VRT-325, experimental corrector drug; HC, healthy control; Ca2+, calcium.
Zhou and co-authors introduced CF mutations into a promyelocytic cell line, which could be differentiated into neutrophil-like cells (19). These studies then demonstrated that the CFTR protein was transferred to phagosomes created during phagocytosis. A CFTR corrector drug increased mutant CFTR targeting to phagosomes.
Hayes et al. published a study in 2020 that reported that CFTR potentiator therapy (i.e., ivacaftor) corrected defective degranulation of antimicrobial enzymes in CF neutrophils (24). Circulating neutrophils from healthy control subjects and from CF patients with the Phe508del mutation had similar phagocytic activities. However, staphylococcal killing was significantly reduced by the neutrophils from patients with CF. Degranulation was dysregulated in these CF patients, and there was increased fusion of primary granules and decreased fusion of secondary and tertiary granules with phagosomes. These investigators then studied the effect of ivacaftor on neutrophils patients with Gly551Asp mutations, which is a gating CFTR mutation. This CFTR potentiator decreased primary granule fusion and increased secondary and tertiary granule fusions with phagolysosomes in patients with CF. Consequently, neutrophils from treated patients were similar to neutrophils from healthy control subjects. This study also demonstrated that the pH in phagolysosomes from patients with CF was more alkaline than normal, and this created suboptimal conditions for bacterial killing. Ivacaftor reduced the pH in these phagosomes, and this likely increased bactericidal activity.
Guerra et al. investigated the effects of ivacaftor on neutrophil ROS production in CF neutrophils (23). These investigators studied pediatric patients at intervals throughout the course of the treatment (baseline, 1 to 3 months, and 6 months). ROS production was determined by fluorescence, mass spectrometry, and quantitative real time polymerase chain reaction (qtPCR). Hydrogen voltage gated channel 1 (HVCN1) function correlates with the amount of ROS production since H+ is required for superoxide formation. Consequently, the level of HVCN1 mRNA should correlate with ROS production. This study reported that oxidative burst activity and HVCN1 mRNA correlated throughout the course of the study, which indicates that HVCN1 was synthesized after drug exposure. Both increased between 1 to 3 months and decreased at 6 months. The significance of this decrease is uncertain.
Pohl et al. studied the effect of ivacaftor on Rab27a function in neutrophils (20). Rab27a is a small GTPase that regulates the degranulation of secondary and tertiary granules (26). First, these investigators confirmed that Cl− concentrations in CF neutrophils (from patients with Gly551Asp mutation) treated with ivacaftor were the same as neutrophils from healthy control subjects. Then Rab27a was labeled and measured in neutrophils from control subjects, patients with Gly551Asp mutations treated with ivacaftor, Phe508del patients, and untreated heterozygous patients. The neutrophils fromGly551Asp patients treated with ivacaftor had similar levels of Rab27a as neutrophils from healthy controls and had increased levels compared to the other groups. Further testing involving CD66b, a secondary granule protein, upregulation confirmed these results. P. aeruginosa survival was 11% in in vitro assays using extracellular supernatants from TNF-α stimulated control neutrophils; survival was 23% and 20% in assays using neutrophils isolated from Phe508del homozygous and heterozygous patients, respectively. Assays of neutrophils from ivacaftor-treated patients had 10% bacterial survival, a result similar to assays with healthy control neutrophils. Other experiments demonstrated that the proteins from primary granules were increased, and proteins from secondary and tertiary granules were decreased on neutrophil membranes. In addition, the extracellular release of MPO was increased in neutrophil stimulated by TNF-α, and proteins from secondary and tertiary granules were decreased. Intracellular concentrations of chloride and sodium were increased in neutrophils from patients with CF, and magnesium levels were decreased. Similar results were observed in control neutrophils treated with the CFTR inhibitors.
Overall, these studies demonstrate that ivacaftor has definite effects on neutrophils isolated from treated patients. In particular, these studies indicated that this drug corrected dysregulated degranulation in neutrophils, increased the respiratory burst, and decreased the pH in phagolysosomes. These effects should increase bacterial killing in phagolysosomes.
CFTR modulator effects on airway inflammation using sputum collection studies
Several investigators have studied the effect of Trikafta® on airway inflammation in patients with CF (Table 5). This combination drug includes two CFTR correctors (elexacaftor, tezacaftor) and one potentiator (ivacaftor) This study periods ranged from 1 to 12 months and the study samples included both sputum and blood.
Table 5
Author, year | Drug and protocol | Results |
---|---|---|
Schaupp et al., 2023 (27) | ETI for 12 months; sputum samples | Increased elastic modulus and viscous modulus of sputum, decreased Pseudomonas number, increased microbiome diversity, reduced free NE |
Casey et al., 2023 (28) | ETI for 12 months sputum and plasma samples | Decreased NE, IL-1B, IL-8, Pseudomonas and neutrophils in the sputum; decreased IL-6, CRP, soluble TNF receptor 1 in plasma |
Lepissier et al., 2023 (29) | ETI for 1 month; sputum and blood samples | Decreased NE, IL-1B, IL-8 in sputum; Decreased neutrophil and CRP in blood |
Sheikh et al., 2023 (30) | Patients treated with ETI; serum samples for cytokines | Decreased levels of pro-inflammatory cytokines (IL-6, IL-8, IL-13, IL-17A); decreased % circulating neutrophils |
CFTR, cystic fibrosis transmembrane conductance regulator; ETI, elexacaftor, tezacaftor, ivacaftor; NE, neutrophil elastase; IL, interleukin; CRP, C-reactive protein; TNF, tumor necrosis factor.
These 4 studies demonstrate that combination therapy with CFTR modulators reduces inflammation in CF sputum with a reduction in neutrophil numbers, neutrophil elastase, Pseudomonas numbers, and inflammatory cytokines (27-30). These drugs improved sputum mechanical properties, which should facilitate sputum and bacterial clearance by the mucociliary apparatus. They also reduced systemic inflammatory responses with reductions in neutrophil numbers and cytokines in peripheral blood. These results may reflect drug effects on neutrophils in vivo or drug effects on airway epithelium, which improves mucociliary function and reduces inflammation and the number of neutrophils in airway secretions.
Clinical considerations based on drug and bacteria characteristics
The CFTR protein is found in intracellular organelles, such as endosomes, lysosomes, phagosomes, and mitochondria (31). Consequently, mutations in this protein have the potential to cause dysregulation of intracellular signaling pathways other than phagolysosome formation and bacterial killing. Mutant proteins in mitochondria inhibit electron transport chain activity, reduce adenosine triphosphate production, and decrease mitochondrial membrane potentials. It also decreases calcium uptake in the mitochondria, which can trigger extracellular calcium release and activate inflammation. Ivacaftor was the first drug approved to treat CFTR protein abnormalities; it is a quinolone that potentiates abnormal CFTR proteins and inhibits several cytochrome P450 enzymes. Its chemical formula is C24H28N2O3, and its molecular weight is 392.5 g/mol. Ivacaftor may have antibacterial effects independent of its effect on the CFTR regulator.
Reznikov et al. reported that this drug decreased bioluminescence of S. aureus cultures and decreased the number of colony-forming units in cultures (32). The combination of ivacaftor and vancomycin had a greater effect on these parameters then either drug alone. Ivacaftor also had a similar effect on P. aeruginosa after the outer membrane of the bacteria had been permeabilized before exposure.
Jennings et al. analyzed the effect of varying concentrations of HOCL on bacterial pathogens associated with CF and bacterial pathogens typically not associated with CF (33). P. aeruginosa and S. aureus survived at a higher concentration of HOCL than did Streptococcus pneumoniae, Klebsiella pneumoniae, and Escherichia coli. In addition, neutrophils derived from cells with a CFTR mutation killed Pseudomonas less efficiently than wild type cells. This study indicated that the bacteria associated with CF exacerbation are more resistant to oxidative injury than other bacteria, and this likely contributes to their role in chronic and recurrent CF infections.
Consequently, the combination of ivacaftor and antibiotics in airway secretions may increase bacterial killing independent of this drug’s effect on neutrophil function.
Conclusions
These studies demonstrate that CF mutations have important effects on neutrophil function and response to infections (34), which contribute to the susceptibility of CF patients to recurrent infections with S. aureus, Haemophilus influenzae, and P. aeruginosa (6). Experimental studies have investigated isolated neutrophils from CF patients and demonstrated that there is a defect in the oxygen-dependent killing pathway. The defective CFTR channels in the phagolysosome membrane in CF patients decreases the transfer of Cl− into phagolysosomes (12). This reduces the production of HOCl and reduces neutrophil bactericidal activity since HOCl formation is the final step in the oxygen-dependent killing pathway.
Chloride ion concentrations also influence the function of normal neutrophils. Normal neutrophils placed in high Cl− environments have increased Pseudomonas bactericidal activity, whereas neutrophils in low Cl− environments had decreased bactericidal activity, which supports a Cl− dependent mechanism of killing. However, inhibition of NADPH oxidase, MPO, and CFTR in neutrophils does not completely inhibit killing activity, and this indicates that there must be other non-oxidant dependent pathway(s) for killing. This residual bactericidal activity likely involves proteolytic enzymes in phagolysosomes. Another measure of the oxygen-dependent pathway is the production of the intermediates, superoxide radicals and H2O2 (ROS). Using fluorescence and mass spectrometry, the ROS production in CF neutrophils obtained from peripheral blood and sputum can be measured. Sputum neutrophils from CF patients had decreased ROS production compared to the blood neutrophils. This not only indicates that there is an inherent defect in the oxygen-dependent pathway but also a difference between blood and sputum neutrophils. Neutrophils recovered from sites of chronic inflammation may have reduced functional activity secondary to reduced availability of metabolic substrates (functional exhaustion).
CF treatment with ivacaftor normalizes respiratory secretions in CF patients, and it improves neutrophil function. Ivacaftor corrects the dysregulated degranulation process in CF neutrophils. In particular, the increased levels of MPO (a crucial enzyme in the oxygen-dependent pathway) in CF neutrophils and the decreased degranulation of secondary and tertiary granules are corrected. HVCN1 is a proton channel in the phagolysosome membrane that transfers hydrogen ions into the vesicle to form superoxide. Ivacaftor upregulates HVCN1, thereby increasing oxidative burst and CF neutrophil bactericidal activity. Finally, Rab27a regulates the degranulation of secondary and tertiary granules, and in CF neutrophils treated with ivacaftor, this protein level is comparable to the level in normal neutrophils.
In summary, neutrophils from CF patients have reduced bactericidal activity, which contributes to recurrent infections in CF patients. Recent studies indicate that there is a significant defect in the oxygen-dependent killing (19-25). Ivacaftor improved CFTR function and provides important benefits for CF patients through its effect on the respiratory epithelium. It also increases neutrophil bactericidal activity by upregulating the oxidative pathway and restoring degranulation function. These studies suggest that measuring neutrophil function in CF patients can help classify host defense abnormalities in these patients, which may depend on the exact mutation and that neutrophils provide a readily available biological tissue to study the effects of CFTR potentiator drugs.
However, studies on host defenses and lung injury in patients with CF are complicated by several confounding factors. Peripheral neutrophils may not have the same activity as neutrophils in lung tissue and in lung secretions. Current drugs used to treat CF have effects on both the respiratory epithelium and on neutrophils. Consequently, determining the predominant effect in patients is quite difficult. The most important drug effect involves the CFTR mutant proteins, but these drugs may also have direct effects and synergistic effects with antibiotics on bacterial killing. In addition, the drug effect will depend on the exact mutation. Research on the pathogenesis and management of CF lung disease will continue to require basic laboratory studies and clinical studies to identify potentially treatable features of this disease.
Acknowledgments
Funding: None.
Footnote
Reporting Checklist: The authors have completed the Narrative Review reporting checklist. Available at https://jtd.amegroups.com/article/view/10.21037/jtd-23-846/rc
Peer Review File: Available at https://jtd.amegroups.com/article/view/10.21037/jtd-23-846/prf
Conflicts of Interest: Both authors have completed the ICMJE uniform disclosure form (available at https://jtd.amegroups.com/article/view/10.21037/jtd-23-846/coif). KN serves as an unpaid editorial board member of Journal of Thoracic Disease from August 2021 to July 2023. The other author has no conflicts of interest to declare.
Ethical Statement: The authors are accountable for all aspects of the work in ensuring that questions related to the accuracy or integrity of any part of the work are appropriately investigated and resolved.
Open Access Statement: This is an Open Access article distributed in accordance with the Creative Commons Attribution-NonCommercial-NoDerivs 4.0 International License (CC BY-NC-ND 4.0), which permits the non-commercial replication and distribution of the article with the strict proviso that no changes or edits are made and the original work is properly cited (including links to both the formal publication through the relevant DOI and the license). See: https://creativecommons.org/licenses/by-nc-nd/4.0/.
References
- Hanssens LS, Duchateau J, Casimir GJ. CFTR Protein: Not Just a Chloride Channel? Cells 2021;10:2844. [Crossref] [PubMed]
- Tahir N, Zahra F. Neutrophilia. In: StatPearls [Internet]. Treasure Island (FL): StatPearls Publishing; 2023.
- Naish E, Wood AJ, Stewart AP, et al. The formation and function of the neutrophil phagosome. Immunol Rev 2023;314:158-80. [Crossref] [PubMed]
- Cowland JB, Borregaard N. Granulopoiesis and granules of human neutrophils. Immunol Rev 2016;273:11-28. [Crossref] [PubMed]
- Respiratory burst. Wikipedia. Available online: https://en.wikipedia.org/wiki/Respiratory_burst. Accessed 7-20-2023.
- Lipuma JJ. The changing microbial epidemiology in cystic fibrosis. Clin Microbiol Rev 2010;23:299-323. [Crossref] [PubMed]
- Kiedrowski MR, Bomberger JM. Viral-Bacterial Co-infections in the Cystic Fibrosis Respiratory Tract. Front Immunol 2018;9:3067. [Crossref] [PubMed]
- Wang G, Nauseef WM. Neutrophil dysfunction in the pathogenesis of cystic fibrosis. Blood 2022;139:2622-31. [Crossref] [PubMed]
- Nichols D, Chmiel J, Berger M. Chronic inflammation in the cystic fibrosis lung: alterations in inter- and intracellular signaling. Clin Rev Allergy Immunol 2008;34:146-62. [Crossref] [PubMed]
- Middleton PG, Mall MA, Dřevínek P, et al. Elexacaftor-Tezacaftor-Ivacaftor for Cystic Fibrosis with a Single Phe508del Allele. N Engl J Med 2019;381:1809-19. [Crossref] [PubMed]
- CFTR. CFTR modulator types. Cystic Fibrosis Foundation. Available online: https://www.cff.org/cftr-modulator-types
- Painter RG, Valentine VG, Lanson NA Jr, et al. CFTR Expression in human neutrophils and the phagolysosomal chlorination defect in cystic fibrosis. Biochemistry 2006;45:10260-9. [Crossref] [PubMed]
- Houston N, Stewart N, Smith DS, et al. Sputum neutrophils in cystic fibrosis patients display a reduced respiratory burst. J Cyst Fibros 2013;12:352-62. [Crossref] [PubMed]
- Robledo-Avila FH, Ruiz-Rosado JD, Brockman KL, et al. Dysregulated Calcium Homeostasis in Cystic Fibrosis Neutrophils Leads to Deficient Antimicrobial Responses. J Immunol 2018;201:2016-27. [Crossref] [PubMed]
- Painter RG, Bonvillain RW, Valentine VG, et al. The role of chloride anion and CFTR in killing of Pseudomonas aeruginosa by normal and CF neutrophils. J Leukoc Biol 2008;83:1345-53. [Crossref] [PubMed]
- Bakalović G, Bokonjić D, Mihajlović D, et al. Dysfunctions of Neutrophils in the Peripheral Blood of Children with Cystic Fibrosis. Biomedicines 2023;11:1725. [Crossref] [PubMed]
- Kelk D, Logan J, Andersen I, et al. Neutrophil respiratory burst activity is not exaggerated in cystic fibrosis. J Cyst Fibros 2022;21:707-12. [Crossref] [PubMed]
- Duckers J, Lesher B, Thorat T, et al. Real-World Outcomes of Ivacaftor Treatment in People with Cystic Fibrosis: A Systematic Review. J Clin Med 2021;10:1527. [Crossref] [PubMed]
- Zhou Y, Song K, Painter RG, et al. Cystic fibrosis transmembrane conductance regulator recruitment to phagosomes in neutrophils. J Innate Immun 2013;5:219-30. [Crossref] [PubMed]
- Pohl K, Hayes E, Keenan J, et al. A neutrophil intrinsic impairment affecting Rab27a and degranulation in cystic fibrosis is corrected by CFTR potentiator therapy. Blood 2014;124:999-1009. [Crossref] [PubMed]
- Bratcher PE, Rowe SM, Reeves G, et al. Alterations in blood leukocytes of G551D-bearing cystic fibrosis patients undergoing treatment with ivacaftor. J Cyst Fibros 2016;15:67-73. [Crossref] [PubMed]
- White MM, Geraghty P, Hayes E, et al. Neutrophil Membrane Cholesterol Content is a Key Factor in Cystic Fibrosis Lung Disease. EBioMedicine 2017;23:173-84. [Crossref] [PubMed]
- Guerra L, D'Oria S, Favia M, et al. CFTR-dependent chloride efflux in cystic fibrosis mononuclear cells is increased by ivacaftor therapy. Pediatr Pulmonol 2017;52:900-8. [Crossref] [PubMed]
- Hayes E, Murphy MP, Pohl K, et al. Altered Degranulation and pH of Neutrophil Phagosomes Impacts Antimicrobial Efficiency in Cystic Fibrosis. Front Immunol 2020;11:600033. [Crossref] [PubMed]
- Hardisty GR, Law SM, Carter S, et al. Ivacaftor modifies cystic fibrosis neutrophil phenotype in subjects with R117H residual function CFTR mutations. Eur Respir J 2021;57:2002161. [Crossref] [PubMed]
- Herrero-Turrión MJ, Calafat J, Janssen H, et al. Rab27a regulates exocytosis of tertiary and specific granules in human neutrophils. J Immunol 2008;181:3793-803. [Crossref] [PubMed]
- Schaupp L, Addante A, Völler M, et al. Longitudinal effects of elexacaftor/tezacaftor/ivacaftor on sputum viscoelastic properties, airway infection and inflammation in patients with cystic fibrosis. Eur Respir J 2023;62:2202153. [Crossref] [PubMed]
- Casey M, Gabillard-Lefort C, McElvaney OF, et al. Effect of elexacaftor/tezacaftor/ivacaftor on airway and systemic inflammation in cystic fibrosis. Thorax 2023;78:835-9. [Crossref] [PubMed]
- Lepissier A, Bonnel AS, Wizla N, et al. Moving the Dial on Airway Inflammation in Response to Trikafta in Adolescents with Cystic Fibrosis. Am J Respir Crit Care Med 2023;207:792-5. [Crossref] [PubMed]
- Sheikh S, Britt RD Jr, Ryan-Wenger NA, et al. Impact of elexacaftor-tezacaftor-ivacaftor on bacterial colonization and inflammatory responses in cystic fibrosis. Pediatr Pulmonol 2023;58:825-33. [Crossref] [PubMed]
- Lukasiak A, Zajac M. The Distribution and Role of the CFTR Protein in the Intracellular Compartments. Membranes (Basel) 2021;11:804. [Crossref] [PubMed]
- Reznikov LR, Abou Alaiwa MH, Dohrn CL, et al. Antibacterial properties of the CFTR potentiator ivacaftor. J Cyst Fibros 2014;13:515-9. [Crossref] [PubMed]
- Jennings S, Hu Y, Wellems D, et al. Neutrophil defect and lung pathogen selection in cystic fibrosis. J Leukoc Biol 2023;113:604-14. [Crossref] [PubMed]
- Hayes E, Pohl K, McElvaney NG, et al. The cystic fibrosis neutrophil: a specialized yet potentially defective cell. Arch Immunol Ther Exp (Warsz) 2011;59:97-112. [Crossref] [PubMed]