Which bioabsorbable sheet is optimal for combined application with fibrin sealant against alveolar air leakage?
Highlight box
Key findings
• When the Rub + Soak B combination technique was used, the 0.15 mm-thick non-woven felt was the most effective in terms of the thickness and/or weaving pattern of the polyglycolic acid (PGA) sheet, and oxidised regenerated cellulose (ORC) was the least effective in terms of material quality.
What is known and what is new?
• The combination of fibrin sealant and synthetic bioabsorbable sheets is more effective than fibrin sealant alone in controlling alveolar air leakage. The Rub + Soak B combination technique is the most optimal technique for this combination with 0.15 mm-thick PGA felt.
• 0.15 mm-thick non-woven PGA felt is the most effective and meshed ORC is the least effective in the Rub + Soak B combination technique. TachoSil® combined with fibrin sealant was comparable to 0.15 mm-thick PGA felt.
What is the implication, and what should change now?
• As a routine practice in lung surgery, the Rub + Soak B technique utilizing a 0.15 mm-thick non-woven PGA felt is recommended.
Introduction
Background
Postoperative alveolar air leakage remains a major cause of morbidity after lung resection. This leads to prolonged chest tube drainage time, which is associated with an increased risk of empyema development and other serious complications (1). Since the early 1980s, fibrin sealant (FS) has been widely used as an effective tool to prevent pulmonary air leakage during lung surgery (1-4). The application of FS in combination with synthetic bioabsorbable sheets has been increasingly recognized as a more effective tool for the control of alveolar air leakage than the single use of FS (5), and this combination of a variety of techniques has been applied in pulmonary surgery (6-9). We previously conducted a comparative experimental study investigating the most optimal technique for the combined application of FS and 0.15 mm-thick non-woven polyglycolic acid (PGA) felt in an ex vivo porcine lung model, and demonstrated that the Rub + Soak B technique had the best sealing effect (10). The ex vivo porcine lung model is considered an appropriate model that closely reflects human biology at pulmonary surgery, as demonstrated in that study. In routine lung surgery, thoracic surgeons usually encounter air leaks from several sources in the lungs, such as (I) pleural defects; (II) lung parenchymal lacerations; (III) raw parenchymal stumps; and (IV) stapled or sutured lines. The author adopts “zero leak policy” that requires complete air leak control at the end of surgery. Meticulous mattress sutures with pledgets are frequently used as the first choice for controlling air leaks from the above sources, followed by repeated leak tests, and the sutured site is further reinforced by coverage with bioabsorbable sheet and FS after almost complete control of air leaks. The experimental model of the previous (10) and the present study was determined to bear such a scenario in mind.
Rationale and knowledge gap
Various types of synthetic bioabsorbable sheet have been recently made available for surgical use in terms of thickness (0.15, 0.3, or 0.5 mm non-woven PGA felt), weaving pattern (non-woven felt, woven, or knitted PGA), or quality material [PGA or oxidized regenerated cellulose (ORC)]. Although a 0.15 mm-thick non-woven PGA felt is popularly used in the combined application of FS for the control of alveolar air leakage, there is no evidence that it is the most suitable sheet for the Rub + Soak B technique, as proven by the best sealing effect. Therefore, in this study, we performed a comparative study with the same ex vivo porcine lung model as before (10) to investigate which sheet had the best sealing effect in the Rub + Soak B combination method among the various types of synthetic bioabsorbable sheets. The sealing effect of TachoSil® (CSL Behring Co., Tokyo, Japan), a ready-to-use 5 mm-thick collagen patch sponge that was surficially applied with dried FS, was also compared.
Objective
The objective of this study was to investigate which synthetic bioabsorbable sheet had the best sealing effect against alveolar air leakage when used in combination with FS in terms of material quality, weaving pattern, and/or thickness. While the ARRIVE reporting checklist is designed to improve the reporting of in vivo studies, we decide to follow the ARRIVE reporting checklist in this ex vivo study considering many applicable items for better reporting in the study design, statistical analysis and results (available at https://jtd.amegroups.com/article/view/10.21037/jtd-23-773/rc).
Methods
Animals
Male swine aged 3–4 months with weight of 32–46 kg raised in standard house and husbandry conditions by the breeder in Hokkaido, Japan were used in this study (n=14). After humane euthanization the heart-lung blocks were retrieved in a slaughterhouse, kept at 4 ℃, and used for experiments with no perfusion within 2 to 3 days after retrieval. The maximum warm ischemic time was approximately 15 min. No live animals were involved in experimental procedures, and all experiments were performed on ex vivo porcine lung tissue. All used heart-lung blocks were almost the same size with no remarkable abnormalities, such as pneumonia, showing good expansion, and no samples were excluded from the experiment. All the animals used in this study received humane care in accordance with the standard guidelines of the Science Council of Japan (http://www.scj.go.jp/en/animal/index.html).
Experimental setting
An ex vivo porcine lung model was used in this experiment as previously described (10). A protocol was prepared before the study without registration. Intubation was performed using a tracheal tube, which was fixed, and connected to a manometer. The contralateral main bronchus was alternately clamped and airway pressure was regulated by increasing the flow rate of nitrogen gas, which was monitored using a digital manometer with a graphical display demonstrating sequential linear pressure changes over time (Video 1). A standardized 20 mm × 30 mm pleural defect with a depth of 1.0 mm was created on the lung surface, with the lung fully expanded at a positive airway pressure of 10 cmH2O. Two pleural defects per lung in the upper and lower lobes and four pleural defects per heart-lung block were used in the experiment. This was performed with electric cautery in the coagulation mode at 35 W (ICC 350-MR; Erbe, Tübingen, Germany) by one operator through both the previous (10) and the present study with minimal variance. This standardized pleural defect almost constantly has minimal air leak, with lung blocks expanded with an airway pressure of 6 cmH2O. As soon as the pressure increased beyond 6 cmH2O, air leakage from the pleural defect began and increased more vigorously according to a gradual linear increase in airway pressure without exception (Video 1). The pleural defects were covered with different synthetic bioabsorbable sheets (three pieces of 15 mm × 30 mm in Groups 1, 2, 3, 4, 5, and 6; one piece of 40 mm × 30 mm in Groups 7, 8, and 9; Figure 1), with the left lung expanded and minimal air leakage from the pleural defect observed under an airway pressure of 6 cmH2O. The covered surface was maintained at rest for 5 min and then gently immersed in normal saline solution at room temperature (approximately 20 ℃), while the airway pressure was maintained at 6 cmH2O. In Groups 7 and 8, in which the TachoSil® sheet was used, the entire sheet was gently compressed onto the lung using gauze for 3 min immediately after the attachment of the sheet and kept at rest for 5 min. The airway pressure was slowly increased, and the minimum positive airway pressure causing air leakage from the defect [seal-breaking burst pressure (SBBP)] was recorded by visual assessment for each sample and then compared between the groups (Video 1). These procedures were performed by only one operator (the corresponding author) with procedural uniformity. The order of each experimental procedure was assigned randomly from one group to the other, not confined to only one group. When the amount of air leak from the first defect site was relatively large and the pressure increase was negatively affected in the sealing experiment for the second defect, the hilum of the lung proximal to the first defect site was partially clamped using clamping devices, and the air leak was controlled.
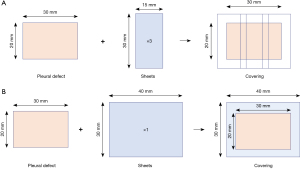
FS application
The FS used in the present study was manufactured by CSL Behring Co. (Beriplast® Combi-Set, Tokyo, Japan). It includes separate vials containing the individual agents that form fibrin clots when combined. The fibrinogen solution is a protein concentrate that comprises fibrinogen, aprotinin, a fibrinolysis inhibitor, and factor XIII, which stabilizes fibrin by intermolecular cross-linkage. The thrombin solution consists of thrombin reconstituted in calcium chloride solution. The mechanism of clot formation by FSs has been previously described (11-14). A total of 1.2 mL of each component solution was used in Groups 1, 4, 5, 6, 8, and 9, which was more than sufficient. In Groups that used thicker sheets, such as Groups 2 and 3, a total of 1.5 mL of each component solution was used for further impregnation of sheets.
The method for sheet covering of pleural defect
In Groups 1, 2, 3, 4, 5, and 6, three pieces of 15 mm × 30 mm sheets were attached, with their margins doubled to cover the 5 mm-width outer margin of the 20 mm × 30 mm pleural defects (Figure 1A). In Groups 7, 8, and 9, one piece of 40 mm × 30 mm sheet was attached to cover the 5 mm-width outer margin of 20 mm × 30 mm pleural defects (Figure 1B). In all Groups except for Groups 7 and 8, in which the TachoSil sheet was used, pleural defects were covered using the previously reported Rub + Soak B technique, which comprises the following steps. First, the fibrinogen solution was dripped and softly rubbed onto the pleural defect. Next, a bioabsorbable sheet that had been soaked in thrombin solution to the level of no remnant air left within its microframework was pasted to the area. The remaining fibrinogen and thrombin solutions (half the original quantity) were alternately spread over this area (Video 1). In Group 7, one 40 mm × 30 mm TachoSil sheet was pasted only with saline moistening. In Group 8, a 40 mm × 30 mm TachoSil sheet was pasted using the quasi-Rub + Soak B technique, which involves rubbing the fibrinogen solution on the pleural defect area and attaching the TachoSil sheet moistened with the thrombin solution only on the attaching aspect. In Group 9, one 40 mm × 30 mm Neoveil sheet (Gunze Co., Kyoto, Japan) was attached using the Rub + Soak B technique.
Experiment 1 (evaluation for the influence of PGA felt thickness)
A comparison of the PGA felt of three thickness (0.15, 0.3, and 0.5 mm) was performed in Experiment 1. The 20 mm × 30 mm standardized pleural defects were covered with 3 pieces of each thickness of 15 mm × 30 mm bioabsorbable sheet using the previously reported Rub + Soak B technique in Groups 1, 2, and 3: 0.15 mm-thick PGA felt sheet (Neoveil®) [Group 1 (PGA0.15), 5 defects]; 0.3 mm-thick Neoveil® sheet [Group 2 (PGA0.3), 7 defects]; 0.5 mm-thick Neoveil® sheet [Group 3 (PGA0.5), 7 defects] (Figure 1A).
Experiment 2 (evaluation for the influence of quality material and/or weave pattern)
A comparison of bioabsorbable sheets regarding their quality material (PGA and ORC) and weave pattern (woven PGA and knitted PGA) was performed in Experiment 2. The 20 mm × 30 mm standardized pleural defects were covered with 3 pieces of each type, regarding quality material and weave pattern of 15 mm × 30 mm bioabsorbable sheet using the previously-reported Rub + Soak B technique in Groups 1, 4, 5, and 6: 0.15 mm-thick PGA felt sheet (Neoveil®; Gunze Co., Kyoto, Japan) [Group 1 (PGA0.15), 5 defects]; 0.15 mm-thick coarsely-meshed ORC sheet (Surgicel Absorbable Hemostat®; Johnson & Johnson K. K., Tokyo, Japan) [Group 4 (ORC), 7 defects]; 0.11 mm-thick woven PGA sheet (Vicryl Mesh Woven®; Johnson & Johnson K. K., Tokyo, Japan) [Group 5 (W-PGA0.11), 7 defects]; and 0.18 mm-thick knitted PGA sheet (Vicryl Mesh Knitted®; Johnson & Johnson K. K., Tokyo, Japan) [Group 6 (K-PGA0.18), 7 defects] (Figure 1A).
Experiment 3 (evaluation for TachoSil with or without FS)
In Experiment 3, TachoSil with or without FS and 0.15 mm-thick PGA felt sheet were compared using a coverage technique with a single-piece sheet (Figure 1B). The 20 mm ×30 mm standardized pleural defects were covered with one piece of 40 mm × 30 mm bioabsorbable sheet in Groups 7, 8, and 9: saline-moistened TachoSil [Group 7 (TachoSil), 5 defects]; TachoSil combined with FS [Group 8 (FS + TachoSil), 6 defects]; and 0.15 mm-thick PGA felt sheet (Neoveil®) [Group 9 (1p-PGA0.15), 5 defects]. As TachoSil® cannot be applied one sheet over another because of its thickness of 5 mm, the 20 mm ×30 mm standardized pleural defects were covered not with three pieces of 30 mm ×15 mm TachoSil® but with one piece of 30 mm × 40 mm TachoSil® in Groups 7 (TachoSil) and 8 (FS + TachoSil). For valid comparison within Experiment 3, Group 9 (1p-PGA0.15) (one piece of 0.15 mm-thick PGA felt with a size of 40 mm × 30 mm) was added instead of Group 1 (PGA0.15) (three pieces of 0.15 mm-thick PGA felt with a size of 15 mm × 30 mm). In Group 7 (TachoSil), the pleural defects were covered with saline-moistened TachoSil® alone, and thrombin-solution-moistened TachoSil® after rubbing the fibrinogen solution on the pleural defect area in Group 8 (FS + TachoSil). The sample size of each group (n=5–7) was determined with reference to a previous study using the same ex vivo porcine lung model, which demonstrated that the Rub + Soak B technique had the best sealing effect (10).
A macroscopic photograph of the sealing technique applied to each group in all the experiments is shown in Figure 2. Table 1 demonstrates a summary of the group characteristics, including sheet type, thickness, and FS amount.
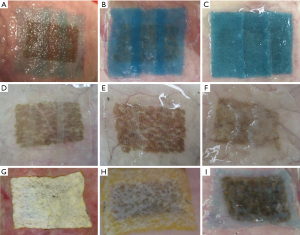
Table 1
Groups | Sheet | Thickness (mm) | Applied FS (cc) |
---|---|---|---|
1 (PGA0.15) | 0.15 mm-PGA felt | 0.15 | 1.2 |
2 (PGA0.3) | 0.3 mm-PGA felt | 0.3 | 1.5 |
3 (PGA0.5) | 0.5 mm-PGA felt | 0.5 | 1.5 |
4 (ORC) | ORC sheet | 0.15 | 1.2 |
5 (W-PGA0.11) | Vicryl Mesh Woven | 0.11 | 1.2 |
6 (K-PGA0.18) | Vicryl Mesh Knitted | 0.18 | 1.2 |
7 (TachoSil) | TachoSil | 5 | 0 |
8 (FS + TachoSil) | Fibrin sealant + TachoSil | 5 | 1.2 |
9 (1p-PGA0.15) | 0.15 mm-PGA felt (1 piece) | 0.15 | 1.2 |
FS, fibrin sealant; PGA, polyglycolic acid; ORC, oxidised regenerated cellulose.
Histological examinations
Because the fibrin seal breaks during the SBBP measurement, histological specimens of the coverage sites were obtained from lung samples representative of groups that had not been used for the SBBP measurement. Five minutes after the sealing procedure, the entire lung was fixed with 10% neutral formalin by immersion and gradual endobronchial injection until the lungs were wholly expanded. After fixation, each defect site was excised, embedded in paraffin, and processed to obtain 3 µm sections for hematoxylin-eosin and phosphotungstic acid hematoxylin (PTAH) staining, so that the fibrin clot could be better observed. The specimens were analyzed in the pathological division of the Uji Tokushu-kai Hospital.
Statistical analysis
Data were analyzed using the Kruskal-Wallis non-parametric test and Steel-Dwass multiple comparison post-hoc tests with comparisons among all pairs of columns (R version 4. 1. 2; R Foundation for Statistical Computing, Vienna, Austria). All data are expressed as mean ± standard deviation (SD). Statistical significance was set at P<0.05.
Results
Experiment 1 (evaluation of the influence of PGA felt thickness) (Figure 3A)
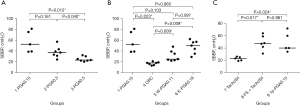
The SBBP required to cause air leakage from the covered pleural defect in the swine lung in Group 1 (PGA0.15) (57.1±20 cmH2O) was significantly higher than that in Group 3 (PGA0.5) (24.9±4.5 cmH2O) (P=0.012) and showed a trend toward higher pressure than Group 2 (PGA0.3) (37.7±11 cmH2O) (P=0.161). The SBBP in Group 2 (PGA0.3) was significantly higher than that in Group 3 (PGA0.5) (24.9±4.5 cmH2O) (P=0.040).
Experiment 2 (evaluation of the influence of material quality and/or weave patterns) (Figure 3B)
The SBBP in Group 4 (ORC) (17.0±2.8 cmH2O) was significantly lower than that in Groups 1 (PGA0.15) (57.1±20 cmH2O), 5 (W-PGA0.11) (30.9±10 cmH2O), and 6 (K-PGA0.18) (47.6±12 cmH2O), respectively. The SBBP in Group 6 (K-PGA0.18) (47.6±12 cmH2O) showed a trend toward higher pressure than in Group 5 (W-PGA0.11) (30.9±10 cmH2O) (P=0.097).
Experiment 3 (evaluation of TachoSil with or without FS) (Figure 3C)
The SBBP in Group 7 (TachoSil) (22.6±3.1 cmH2O) was significantly lower than that in Groups 8 (FS + TachoSil) (48.5±12 cm H2O) and 9 (1p-PGA0.15) (49.2±16 cm H2O) (Figure 4). There was no significant difference between Groups 8 (FS + TachoSil) and 9 (1p-PGA0.15) (P=0.981, Figure 5). When all groups across Experiments 1, 2, and 3 were compared, Group 4 (ORC) exhibited the lowest SBBP, followed by Groups 3 (PGA0.5), and 7 (TachoSil) (Figure 4).
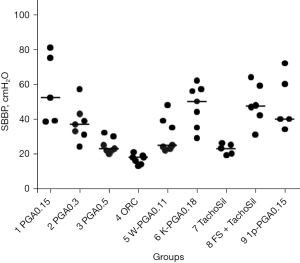
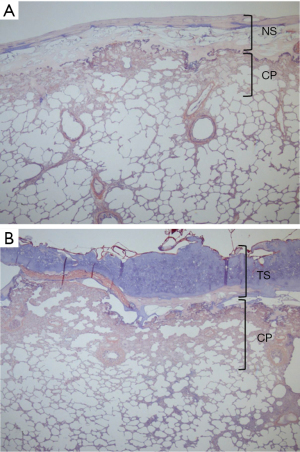
The SBBP (mean ± SD; mmHg) of each group in all experiments is summarized in Table 2.
Table 2
Groups | Sheet | SBBP (mmHg)† |
---|---|---|
1 (PGA0.15) | 0.15 mm-PGA felt | 57.1±20 |
2 (PGA0.3) | 0.3 mm-PGA felt | 37.7±11 |
3 (PGA0.5) | 0.5 mm-PGA felt | 24.9±4.5 |
4 (ORC) | ORC sheet | 17.0±2.8 |
5 (W-PGA0.11) | Vicryl Mesh Woven | 30.9±10 |
6 (K-PGA0.18) | Vicryl Mesh Knitted | 47.6±12 |
7 (TachoSil) | TachoSil | 22.6±3.1 |
8 (FS + TachoSil) | Fibrin sealant + TachoSil | 48.5±12 |
9 (1p-PGA0.15) | 0.15 mm-Neoveil (1 piece) | 49.2±16 |
†, mean ± standard deviation. SBBP, seal-breaking burst pressure; PGA, polyglycolic acid; ORC, oxidised regenerated cellulose; FS, fibrin sealant.
Histological assessment
The layers of fibrin and sheets covering the pleural defects and the penetration of the fibrin clot into the lung tissue, such as the foothold, were observed on histological assessment in all groups, except for Group 7. Histological findings of PTAH staining in Group 9 (1p-PGA0.15) (one piece of 0.15 mm-thick PGA felt with a size of 40 mm × 30 mm) (A) and Group 8 (FS + TachoSil) (TachoSil combined with FS) (B) are shown in Figure 5. The firmly fixed layers of fibrin and fabrics covering the pleural defects and the penetration of the fibrin clot into the lung tissue were demonstrated.
Discussion
Key findings
In the setting of the Rub + Soak B combination technique, the 0.15 mm non-woven felt was the most effective in terms of the thickness and/or weaving pattern of the PGA, and the meshed ORC was the least effective in terms of material quality. The sealing effect of TachoSil® combined with FS was comparable to the 0.15 mm-thick non-woven PGA felt in the Rub + Soak B combination technique.
Strengths and limitations
The original Morikawa’s combination method consists of rubbing one FS component on the lung tissue and impregnating the bioabsorbable sheet with the other component, followed by attaching the sheet to the rubbed area (6). In 2008, we reported the novel Rub + Soak B technique that we had modified and refined the Morikawa’s method by introducing footholds to anchor the upper sealing structure to the lung tissue and by utilizing the advantage of the physical properties of each FS component (10). We confirmed this to be the most optimal method among several other techniques for the combination of FS and a 0.15 mm-thick non-woven PGA felt by conducting a comparative experimental study with an ex vivo porcine lung model. The Rub + Soak B technique consists of three vital steps: (I) rubbing the more viscous fibrinogen solution (substrate for fibrin clot) over the pleural defect to achieve tissue penetration of the fibrin clot; (II) impregnation of the sheet with a less viscous thrombin solution (catalytic enzyme for fibrin clot formation) to produce consistent integration of the felt fiber into the clot layer; and (III) attaching this thrombin solution-impregnated sheet to the defect so that thrombin can be supplied directly and efficiently to the tissue. These three steps are essential for optimizing the lung tissue sealing. Two parameters determine the effectiveness of this combination: the secure sealant attachment to the lung tissue anchored by the fibrin clot foothold, and the consistency of the organic integration of the three components—the lung parenchyma, micro framework of the fabric fibers, and fibrin clot—with minimal retention of air in the integrated sheet layer. In this study, it was confirmed that a 0.15 mm-thick non-woven PGA felt was the most effective and meshed ORC was the least effective among the various bioabsorbable sheets in the Rub + Soak B combination technique.
This study has several limitations. First, the ex vivo porcine lung model lacks blood supply and has a lower temperature, which is different from the in vivo condition. Second, the sealing effect was evaluated by comparing the SBBPs with a progressive linear increase in airway pressure, which differs from the in vivo conditions of a lung subjected to cyclical ventilation. In routine lung surgery, however, intraoperative air leak tests (sealing tests) are usually performed by sustaining a higher intra-airway pressure that is manually increased by anesthesiologists as the lung expands. If a small air leak is detected, it is completely controlled using meticulous mattress sutures and repeated air leak tests. Lung coverage with a bioabsorbable sheet and FS is then added to these sites to prevent relapsing air leaks postoperatively. The author has adopted such “zero leak policy” that never permits any small air leak persisting at the end of surgery. In this scenario, all patients who undergo lung resection have no postoperative air leak, and their chest tubes are removed within three days. Therefore, the experimental protocol of the present study to measure SBBP along with a progressive linear increase in airway pressure might be clinically relevant, along with such “zero leak policy”. Third, this study did not include a time-serial assessment of the sealing effect, which was measured at only one time point. This study is based on the above-mentioned “zero leak policy” in clinical lung surgery practice, and its essential objectives do not focus on observing the in vivo time-serial process of persistent air leaks during or after surgery but on elucidating the pin-point minimum research question in which bioabsorbable sheets have higher SBBP and are optimal for combined application with FS against alveolar air leakage. In this sense, measurements at a single time point in the protocol of the present study are clinically relevant. Fourth, regarding the validity of using normal swine lungs in the present study instead of morbid lung model, with “zero leak policy” the first choice of air leak control is meticulous mattress suture, and then repeated leak tests and coverage with bioabsorbable sheet and FS on to the sutured site where air leak is completely controlled. Although air leak control in morbid lungs is generally more difficult, such basic handling of air leaks is not different between normal and morbid lungs. Considering that air leak control only by combined coverage with bioabsorbable sheet and FS is not aimed in the above “zero leak policy”, experiments using morbid lung model may not be mandatory.
These limitations should be considered when interpreting the results as a comparable human model, and further studies are required to determine the responses in vivo or in morbid lungs.
Comparison with similar research
There are some comparative data on the sealing effect only between 0.15 mm-thick PGA felt and ORC mesh sheet in combination with FS, demonstrating a better sealing effect of PGA felt compared to the ORC mesh sheet. This finding is partially consistent with the results of the present study. Yang et al. (15) reported that the combination of 0.15 mm-thick PGA felt and FS had significantly better sealing effect than that of 0.15 mm-thick meshed ORC sheet in ex vivo porcine lung segmentectomy model. Sakai et al. (16) also reported that the combination of 0.15 mm-thick PGA felt and FS had significantly better sealing effect than that of 0.15 mm-thick ORC mesh for 12 mm square pleural defect in the observation after up to 24 hours in vivo canine model.
Explanations of findings
In this study, we investigated which type of synthetic bioabsorbable sheet has the best sealing effect with the Rub + Soak B combination method in an ex vivo porcine lung model, comparing several types of sheets, which have recently been made available for surgical use. The basic characteristics of synthetic bioabsorbable sheet fabrics, such as the quality material, weaving pattern, and thickness, determine their softness, elasticity, adaptation to the lung surface, and the extent of fluid absorption within the sheet layer. PGA, ORC, and collagen sponge were compared in this study (Table 1). Variations of each material were studied: for PGA, it was the 0.15 mm-thick Neoveil® [Group 1 (PGA0.15)], 0.3 mm-thick Neoveil® [Group 2 (PGA0.3)], 0.5 mm-thick Neoveil® [Group 3 (PGA0.5)], Vicryl Mesh Woven® [Group 5 (W-PGA0.11)], and Vicryl Mesh Knitted® [Group 6 (K-PGA0.18)] forms; for ORC, it was the 0.15 mm-thick coarsely meshed Surgicel Absorbable Hemostat® [Group 4 (ORC)], and collagen, a fleece sponge coated with dried human fibrinogen and thrombin form [Groups 7 (TachoSil) and 8 (FS + TachoSil)].
In terms of the weaving pattern of the PGA products, Neoveil® is a non-woven fabric felt, Vicryl Mesh Woven® is tightly woven, and Vicryl Mesh Knitted® is roughly knitted. The 0.11 mm-thick Vicryl Mesh Woven® [Group 5 (W-PGA0.11)] had a relatively poorer SBBP, identical to that of the 0.3 mm-thick PGA felt [Group 2 (PGA0.3)]. This was presumably due to its stiffness and hardness, which may cause maladaptation to the fine ruggedness of pleural defects. It also appeared less able to retain fluid within the sheet layer, along with its tight weaving and small interfiber space. In contrast, Vicryl Mesh Knitted® [Group 6 (K-PGA0.18)], 0.18 mm-thick, roughly knitted, softer, mesh sheet, demonstrated the second-best SBBP behind Group 1 (PGA0.15), which was significantly higher than that of Group 4 (ORC). This was likely due to its softness and elasticity, which enabled it to fit meticulously to the finely rugged surface of pleural defects and its ability to retain fluid within the fabric layer. In terms of these characteristics, 0.18 mm-thick Vicryl Mesh Knitted® [Group 6 (K-PGA0.18)] is most similar to the 0.15 mm-thick non-woven PGA felt [Group 1 (PGA0.15)].
When impregnated with a less viscous thrombin solution, PGA functioned as a sponge-like retention device for the fluid. Given that the thrombin solution can easily spill over owing to its lower viscosity, this enables the entire thrombin solution to react efficiently with fibrinogen with minimal spillage (10). The ability to retain fluid within the sheet layer increases in proportion to the thickness of the Neoveil® PGA felt, possibly improving its sealing effect. Based on this hypothesis, the 0.3 mm-thick [Group 2 (PGA0.3)] and 0.5 mm-thick [Group 3 (PGA0.5)] Neoveil® PGA felt were compared with the original 0.15 mm-thick Neoveil® [Group 1 (PGA0.15)]. However, the SBBPs in Groups 2 (PGA0.3) and 3 (PGA0.5) were lower than those in Group 1 (PGA0.15). The reason for this is speculated as the 0.3 mm [Group 2 (PGA0.3)] and 0.5 mm [Group 3 (PGA0.5)] Neoveil® PGA felt are stiffer and bulkier, which made fine adaptation to the lung surface more difficult than in thin, elastic and plastic 0.15 mm-thick PGA felt [Group 1 (PGA0.15)]. Thus, the extent of adaptation of the sheets to the lung surface surpasses the ability to retain fluid within the sheet layer in sealing effect of Rub + Soak B combination method with Neoveil® PGA felt.
Surgicel Absorbable Hemostat® [Group 4 (ORC)] is a 0.15 mm-thick, roughly meshed sheet made of ORC. During hemostasis, the ORC sheet is saturated with blood and swells into a fragile brownish-black gelatinous mass that aids in clot formation, thereby serving as a hemostatic adjunct. When soaked in a fluid, its integrity deteriorates immediately, although it is brittle and fragile. This may explain why Group 4 (ORC) had the lowest SBBP among all groups. As such, ORC might be less suitable in combination with FS for the acute-phase control of air leaks.
Experiment 3 investigated the sealing effect of TachoSil®, with and without additional FS application. TachoSil® is a ready-to-use combination product of liquid and solid sealants—a 5 mm-thick collagen patch sponge coated with dried human fibrinogen and thrombin (17). Upon contact with the wet covering site, coagulation factors dissolve and are activated, initiating the final stage of fibrin clot formation. By compressing the sheet onto the tissue, the sheet transforms into a gelatinous, elastic, and thin patch that aids in the effective control of local hemorrhage or air leaks. TachoSil® has been shown to be effective in reducing perioperative air leaks, time to chest tube removal, and duration of hospital stay after lung surgery (18,19). In Experiment 3, the combination of TachoSil and FS [Group 8 (FS + TachoSil), 48.5±12 cmH2O] produced a significantly higher seal-breaking pressure than saline-moistened TachoSil® alone [Group 7 (TachoSil)], 22.6±3.1 cmH2O) (P=0.017; Figure 3C), which was almost equal to that of the 0.15 mm-thick Neoveil® (1 piece) [Group 9 (1p-PGA0.15), 49.2±16 cmH2O]. When all groups across Experiments 1, 2, and 3 were compared, TachoSil® alone [Group 7 (TachoSil)] produced the second lowest SBBP after Group 4 (ORC). The dried human fibrinogen and thrombin coat on the collagen patch of TachoSil® may not have been able to form a sufficiently secure foothold to anchor the patch to lung tissue by itself (15). Once the fibrinogen solution was rubbed onto the covering area and the TachoSil® surface was moistened with thrombin solution [Group 8 (FS + TachoSil)], TachoSil® demonstrated one of the best SBBPs, comparable to 0.15 mm-Neoveil®, either three pieces [Group 1 (PGA0.15)] or one piece [Group 9 (1p-PGA0.15)]. This suggests that TachoSil® functions as an excellent sealing material once fibrin clot foothold is added. Taken together, the foothold anchoring the upper sealing structure to the lung tissue appears to be essential for effective sealing against alveolar air leakage. This fibrin clot foothold was histologically significant in Groups 8 (FS + TachoSil) and 9 (1p-PGA0.15) (0.15 mm-thick Neoveil® of one piece), as demonstrated in Figure 5.
Implications and actions needed
This study demonstrated that the Rub + Soak B technique utilizing a 0.15 mm-thick non-woven PGA felt is recommended as a routine practice in lung surgery. Our Rub + Soak B method includes the following two parameters that determine the effectiveness of this combination: secure sealant attachment to the lung tissue anchored by the fibrin clot foothold and the consistency of the organic integration of the three components: the lung parenchyma, micro framework of the fabric fibers, and fibrin clot, with minimal retention of air in the integrated sheet layer. These two parameters might become key when next-generation combination sealants are developed, which comprise both solid (bioabsorbable fabrics) and liquid (FSs) states. In case of necessity, the combination of TachoSil® and FS can be an alternative to the Rub + Soak B technique utilizing a 0.15 mm-thick PGA felt.
Conclusions
The 0.15 mm-thick Neoveil® PGA felt is the optimal sheet for use in the Rub + Soak B combination technique of bioabsorbable sheets and FS. The second-best sheet was the Vicryl Mesh Knitted® PGA sheet. Similarly, the combination of TachoSil® and FS also demonstrated an excellent sealing effect equal to that of the 0.15 mm-thick Neoveil® PGA felt. We believe that the use of these sheets in the Rub + Soak B combination technique is promising and could be an important aid in pulmonary surgery.
Acknowledgments
The authors acknowledge Hiroshi Takahashi, BSc (Fujita Medical University, Aich, Japan) for supervising the biostatistical assessment of this study as a biostatistician and Takuji Yamada, MD (Division of Pathology, Uji Tokushu-kai Hospital) for histological assessment of lung samples as a pathologist. The author is also grateful to Ms. Nakamura, Inada, and Tamura (Division of Pathology, Uji Tokushu-kai Hospital) for histological processing and several personnel of CSL Behring Co. for setup, assistance, and observation of the experiment.
Funding: This study was supported by
Footnote
Reporting Checklist: The author has completed the ARRIVE reporting checklist. Available at https://jtd.amegroups.com/article/view/10.21037/jtd-23-773/rc
Data Sharing Statement: Available at https://jtd.amegroups.com/article/view/10.21037/jtd-23-773/dss
Peer Review File: Available at https://jtd.amegroups.com/article/view/10.21037/jtd-23-773/prf
Conflicts of Interest: The author has completed the ICMJE uniform disclosure form (available at https://jtd.amegroups.com/article/view/10.21037/jtd-23-773/coif). HI reports this study was supported by the CSL Behring Co., which has approved the design, analysis and reporting of the study. The author has no other conflicts of interest to declare.
Ethical Statement: The author is accountable for all aspects of the work in ensuring that questions related to the accuracy or integrity of any part of the work are appropriately investigated and resolved. No live animals were involved in experimental procedures, and all experiments were performed on
Open Access Statement: This is an Open Access article distributed in accordance with the Creative Commons Attribution-NonCommercial-NoDerivs 4.0 International License (CC BY-NC-ND 4.0), which permits the non-commercial replication and distribution of the article with the strict proviso that no changes or edits are made and the original work is properly cited (including links to both the formal publication through the relevant DOI and the license). See: https://creativecommons.org/licenses/by-nc-nd/4.0/.
References
- Serra-Mitjans M, Belda-Sanchis J, Rami-Porta R. Surgical sealant for preventing air leaks after pulmonary resections in patients with lung cancer. Cochrane Database Syst Rev 2005;CD003051. [Crossref] [PubMed]
- Türk R, Weidringer JW, Hartel W, et al. Closure of lung leaks by fibrin gluing. Experimental investigations and clinical experience. Thorac Cardiovasc Surg 1983;31:185-6. [Crossref] [PubMed]
- Mouritzen C, Drömer M, Keinecke HO. The effect of fibrin glueing to seal bronchial and alveolar leakages after pulmonary resections and decortications. Eur J Cardiothorac Surg 1993;7:75-80. [Crossref] [PubMed]
- Fabian T, Federico JA, Ponn RB. Fibrin glue in pulmonary resection: a prospective, randomized, blinded study. Ann Thorac Surg 2003;75:1587-92. [Crossref] [PubMed]
- Mizuno H, Hitomi S, Nakamura T, et al. Clinical experience of the combined use of polyglycolide non-woven felt with fibrin glue to prevent postoperative pulmonary fistula. Nihon Kyobu Geka Gakkai Zasshi 1995;43:1559-64.
- Morikawa T, Katoh H. Improved techniques of applying fibrin glue in lung surgery. Eur Surg Res 1999;31:180-6. [Crossref] [PubMed]
- Miyamoto H, Futagawa T, Wang Z, et al. Fibrin glue and bioabsorbable felt patch for intraoperative intractable air leaks. Jpn J Thorac Cardiovasc Surg 2003;51:232-6. [Crossref] [PubMed]
- Tokushima T, Fukuta M, Maeta H, et al. Clinical study of a new method of closing pulmonary fistula in lung surgery: A combination of PGA felt and frictional spraying of fibrin glue. The Journal of the Japanese Association for Chest Surgery 2005;19:798-804.
- Matsumura Y, Okada Y, Shimada K, et al. New surgical technique of pulmonary segmentectomy by ultrasonic scalpel and absorbable sealing materials. Kyobu Geka 2004;57:31-7.
- Itano H. The optimal technique for combined application of fibrin sealant and bioabsorbable felt against alveolar air leakage. Eur J Cardiothorac Surg 2008;33:457-60. [Crossref] [PubMed]
- Greer JP, Foerster J, Lukens JN, et al. Blood coagulation and fibrinolysis. In: Greer JP, Foerster J, Lukens JN, et al. editors. Wintrobe’s clinical hematology. 11th ed. Philadelphia: Lippincott Williams & Wilkins; 2003:721-5.
- Sierra DH. Fibrin sealant adhesive systems: a review of their chemistry, material properties and clinical applications. J Biomater Appl 1993;7:309-52. [Crossref] [PubMed]
- Sierra DH, O'Grady K, Toriumi DM, et al. Modulation of mechanical properties in multiple-component tissue adhesives. J Biomed Mater Res 2000;52:534-42. [Crossref] [PubMed]
- Radosevich M, Goubran HI, Burnouf T. Fibrin sealant: scientific rationale, production methods, properties, and current clinical use. Vox Sang 1997;72:133-43. [Crossref] [PubMed]
- Yang H, Dong Z, Wang H, et al. Efficacy of oxidized regenerated cellulose combined with fibrin glue in reducing pulmonary air leakage after segmentectomy in a porcine lung model. Front Bioeng Biotechnol 2022;10:1052535. [Crossref] [PubMed]
- Sakai T, Matsutani N, Kanai E, et al. Efficacy of a sheet combined with fibrin glue in repair of pleural defect at the early phase after lung surgery in a canine model. Gen Thorac Cardiovasc Surg 2018;66:103-7. [Crossref] [PubMed]
- Lang G, Csekeö A, Stamatis G, et al. Efficacy and safety of topical application of human fibrinogen/thrombin-coated collagen patch (TachoComb) for treatment of air leakage after standard lobectomy. Eur J Cardiothorac Surg 2004;25:160-6. [Crossref] [PubMed]
- Anegg U, Lindenmann J, Matzi V, et al. Efficiency of fleece-bound sealing (TachoSil) of air leaks in lung surgery: a prospective randomised trial. Eur J Cardiothorac Surg 2007;31:198-202. [Crossref] [PubMed]
- Marta GM, Facciolo F, Ladegaard L, et al. Efficacy and safety of TachoSil® versus standard treatment of air leakage after pulmonary lobectomy. Eur J Cardiothorac Surg 2010;38:683-9. [Crossref] [PubMed]