Delayed lung injury on the nonsurgical side increases mortality in patients after lung cancer surgery: a retrospective cohort study
Highlight box
Key findings
• Patients with postoperative delayed acute lung injury (ALI) on the nonsurgical side exhibited a high mortality rate.
What is known and what is new?
• The incidence of pulmonary complications following lung cancer surgery has declined recently; however, postoperative acute lung injury (PALI) is still common.
• The present study aimed to assess the prognosis of PALI after lung cancer surgery on different injury sides, describe its clinical characteristics and identify risk factors.
What is the implication, and what should change now?
• Respiratory effort in patients after lung cancer surgery should be closely monitored and controlled to minimize the risk of lung injury.
Introduction
Despite advancements in surgical techniques and perioperative management, the incidence of postoperative acute lung injury (PALI) after lung cancer surgery remains at 2–7% (1), with a mortality rate ranging from 20–70% (2,3). PALI after lung cancer surgery is typically classified into two subtypes (4): (I) primary acute lung injury (ALI), which is induced by surgery and occurs within 2 days of surgery; and (II) secondary ALI, which is associated with complications such as aspiration, bronchopleural fistula or bronchopneumonia and typically presents between Days 3–10 post-surgery. Nonsurgical-side lung injury is primarily influenced by one-lung ventilation (OLV), and surgical-side lung injury is typically related to ischemia-reperfusion injury and shear stress during reventilation (5,6). Due to the utilization of advanced surgical techniques and perioperative management (7-9), such as intra- and postoperative epidural anesthesia, protective lung ventilation, and goal-directed hemodynamic therapy, the incidence of surgery-induced ALI has decreased significantly in recent years (1).
The pathophysiology of secondary PALI subsequent to lung cancer surgery is multifactorial, resulting from injuries specific to both the ipsilateral (surgical) lung and the contralateral (nonsurgical) lung, in addition to the injuries common to both lungs (4,10). Following lung cancer surgery, there may be discrepancies in bilateral lung compliance or function. Whether this has an effect on the development and progression of PALI is not known. However, research pertaining to this topic is limited, and X-ray examinations remain a reliable diagnostic tool. Radiographic signs usually consist of unilateral or bilateral diffuse infiltrates and may precede or follow clinical symptoms after a time lag (11). Electrical impedance tomography (EIT) is an emerging noninvasive radiation-free technique that enables dynamic bedside monitoring of functional ventilation in lung volume changes (12). It provides images based on the tissue electrical conductivity of the chest and is able to accurately assess regional ventilation distribution (13).
In the present study, we aimed to assess the prognosis of PALI following lung cancer surgery on different injury sides. Additionally, our objectives included describing clinical patterns, identifying risk factors for PALI, and assessing the prognostic significance of ALI subsequent to lung cancer surgery. We present this article in accordance with the STROBE reporting checklist (available at https://jtd.amegroups.com/article/view/10.21037/jtd-23-822/rc).
Methods
Study design
This was a single-center retrospective study that occurred in a surgical intensive care unit (SICU) from January 2019 to December 2020. The study was conducted in accordance with the Declaration of Helsinki (as revised in 2013). The ethics review board of Zhongshan Hospital of Fudan University approved the study protocol (No. B2021-465). All participants or their legal representatives were contacted through telephone calls and signed informed consent.
Participants
Patients requiring respiratory support with severe hypoxemia [PaO2/FiO2 (P/F) ratio <200 mmHg] after thoracic surgery for lung resection were included in this study. Respiratory support included a high-flow nasal catheter (HFNC) device, noninvasive positive pressure ventilation (NPPV), and invasive mechanical ventilation (MV). Patients were excluded based on one or more of the following criteria: (I) esophagectomy; (II) mediastinal surgery; (III) pneumonectomy; (IV) bilateral thoracic surgery; or (V) transient hypoxemia, such as sputum blockage or pleural effusion, that improved immediately after treatment.
All the included patients experienced similar anesthetic processes following strategies of lung-protective ventilation to minimize lung trauma during OLV and reduce postoperative pulmonary complications (10,14). These strategies included epidural anesthesia, low tidal volume ventilation (VT =6 mL/kg), routine positive end-expiratory pressure (PEEP), low FiO2, alveolar recruitment maneuvers (ARMs), pressure-controlled ventilation (PCV), permissive hypercapnia, and perioperative fluid administration (3–10 mL/kg/h) (7,8,15).
Chest radiography was performed daily at the bedside. A computed tomography (CT) scan was performed by the intensive care unit (ICU) physician according to the patient’s condition. Fiberoptic bronchoscopy was performed to check for aspiration and sputum obstruction. Routine lung ultrasonography was performed on each patient. If the patient had moderate to massive pleural effusion (ultrasound showed a dark area of fluid >2 cm), thoracentesis was performed to drain the fluid. The clinical decision for the procedure was made by the ICU physician and respiratory therapists according to local practice.
Radiographic assessment of lung edema (RALE) score and RALE score ratio (RRALE)
The RALE score has been proposed as a noninvasive tool to assess the radiographic extent of lung edema in patients with acute respiratory distress syndrome (ARDS) (5,16,17). The RALE score provides a semiquantitative measure of the extent and density of alveolar opacities on chest radiographs. In the RALE scoring system, lung radiographs are divided into four quadrants (Figure S1): upper/lower right quadrants (Q1/Q2) and upper/lower left quadrants (Q3/Q4). Each quadrant is scored with two indicators (area score and density score). The area score is the extent of alveolar opacities in each quadrant (none: 0 points; <25%: 1 point; 25–50%: 2 points; 50–75%: 3 points; >75%: 4 points), and the density of alveolar opacities in each quadrant is scored as hazy (1 point), moderate (2 points), or dense (3 points). The area score × density score is the score of the specific quadrant, and the total score of the chest X-ray is the sum of the scores of the four quadrants (16). The more severe the lung injury is, the higher the RALE score is. The lung RALE score of the surgical or nonsurgical side is the sum of the scores of the two ipsilateral quadrants (5).
Two experienced investigators independently scored each chest film offline, and the average value was the RALE score for the chest film. They had no access to medical charts, and all the chest films were deidentified.
In this study, we calculated the total RALE and the respective scores of the surgical and nonsurgical lung sides (RALES and RALENS) on the day the patient underwent respiratory support. The RRALE was calculated as the RALE score of the surgical side divided by that of the nonsurgical side (RRALE = RALES/RALENS). RRALE <1 indicated severe lung injury on the nonsurgical side; RRALE ≥1 indicated severe lung injury on the surgical side or similar on both sides. Patients were divided into two groups according to the severity of different sides of lung injury, which was calculated based on the RRALE as described above: the nonsurgical-side lung injury group (RRALE <1) and others (RRALE ≥1).
EIT monitoring
Bedside EIT was utilized to measure the distribution of ventilation between the two sides of the lung. An EIT-dedicated belt containing 16 equally spaced electrodes was placed around the thorax at the fourth or fifth intercostal space and connected to an EIT monitor (PulmoVista 500, Dräger Medical GmbH, Lübeck, Germany) (12). Data were collected on the day the patient received respiratory support and were continuously recorded and analyzed offline.
Next, we selected four regions of interest (ROIs) within the chest contour (Figure S2): ROI 1 (right lung ventral), ROI 2 (left lung ventral), ROI 3 (right lung dorsal), and ROI 4 (left lung dorsal). Differences in tidal ventilation (TV) between the left and right lungs (or the surgical-side and nonsurgical-side lungs) were calculated (18). TVR = TVROI 1 + TVROI 3; TVL = TVROI 2 + TVROI 4. The coefficients TVS and TVNS represented the TV within the surgical and nonsurgical side of the lungs, respectively.
The parameter imbalance index (IMS-NS) was calculated to express the ratio of ventilation between the surgical and nonsurgical lungs: IMS-NS = (TVS − TVNS)/(TVS + TVNS) (18). IMS-NS ranged from +1 (only the surgical lung was ventilated; TVNS =0) to −1 (only the nonsurgical lung was ventilated; TVS =0).
Data collection
Clinical data (including history system, medical order system, nursing record list, respiratory record list, and anesthesia record list) were collected from the database of our institution. Clinical and imaging data were recorded from ICU admission to discharge including patient characteristics, medical history, Acute Physiology and Chronic Health Evaluation (APACHE) II scores, preoperative pulmonary function, operative and anesthetic data (site, side, time, fluid volume, OLV duration, VT, PEEP, and blood transfusion), perioperative fluid balance, vital signs, chest X-ray and CT images, EIT data, respiratory treatment data, and prognosis.
Outcomes
The primary outcome of our study was 90-day all-cause mortality. Secondary outcomes included in-hospital 28-day all-cause mortality, total ICU length of stay (LOS), total hospital LOS and survival at 6 months.
We also analyzed the proportion of patients requiring invasive MV, the duration of MV, and respiratory parameters.
Statistical analysis
Quantitative variables are reported as medians with interquartile ranges (IQRs; 25th–75th percentiles). The Mann-Whitney test was used for comparisons between groups. Cases and rates were used for categorical variables, and Fisher’s exact test was used for comparisons between groups. Survival analysis was performed using the Kaplan-Meier procedure (log-rank test). A P value <0.05 was considered to indicate statistical significance. GraphPad Prism 9 (GraphPad Software, San Diego, CA, USA) was used for statistical analysis and graphs.
Results
A total of 12,084 patients underwent thoracic surgery from January 2019 to December 2020 in our hospital. Of the 1,247 patients admitted to the ICU after surgery, 144 required respiratory support. Subsequently, 58 patients who met the inclusion criteria (P/F ratio <200 mmHg) were included, and 42 of them were excluded; finally, 16 patients were enrolled in this study (Figure 1).
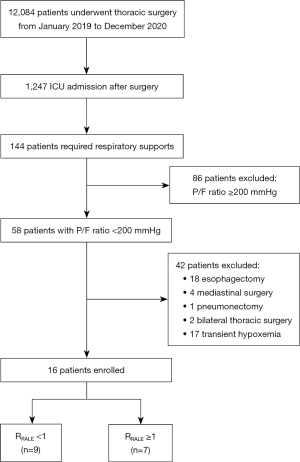
Grouping and RALE score
The RALE score was calculated for each patient, and the patients were categorized into two groups based on the RRALE: nine patients in the RRALE <1 group and seven in the RRALE ≥1 group (Figure 1). There was no significant difference in total RALE score, which was similar between the two groups (33 vs. 32, P=0.42). However, median RRALE was 0.60 (0.40–0.74) and 1.18 (1.13–2.00) in the RRALE <1 and RRALE ≥1 groups, respectively (P<0.001). Details of the RALE score are described in Table 1.
Table 1
Variables | RRALE <1 (n=9) | RRALE ≥1 (n=7) | P value |
---|---|---|---|
Age (years) | 69.0 [58.5, 76.0] | 73.0 [63.0, 75.0] | 0.70 |
Male | 8 (88.89) | 6 (85.71) | >0.99 |
BMI (kg/m2) | 22.2 [20.9, 25.4] | 23.7 [23.1, 25.9] | 0.29 |
Smoking | 3 (33.33) | 3 (42.86) | >0.99 |
Drinking | 3 (33.33) | 1 (14.29) | 0.59 |
Occupation | |||
Manual | 3 (33.33) | 1 (14.29) | 0.59 |
Non manual | 6 (66.67) | 6 (85.71) | |
APACHE II score | 15 [12, 18] | 12 [11, 22] | 0.98 |
Medical history | |||
Hypertension | 3 (33.33) | 5 (71.43) | 0.32 |
Diabetes | 0 | 2 (28.57) | 0.18 |
COPD | 0 | 0 | >0.99 |
Cardiovascular disease | 3 (33.33) | 0 | 0.21 |
Cerebrovascular disease | 0 | 3 (42.86) | 0.06 |
Extra-thoracic malignancy | 1 (11.11) | 0 | >0.99 |
Operation history | |||
Thoracic | 4 (44.44) | 0 | 0.09 |
Non-thoracic | 4 (44.44) | 3 (42.86) | >0.99 |
Preoperative therapy | |||
Chemotherapy, radiotherapy or targeted therapy | 3 (33.33) | 0 | 0.21 |
Anticoagulation | 1 (11.11) | 0 | >0.99 |
Antiplatelet | 1 (11.11) | 0 | >0.99 |
Operative side | |||
Right | 5 (55.56) | 6 (85.71) | 0.31 |
Types of surgery | |||
Bilobectomy | 1 (11.11) | 1 (14.29) | >0.99 |
Lobectomy | 7 (77.78) | 6 (85.71) | |
Segmentectomy | 0 | 0 | |
Wedge | 1 (11.11) | 0 | |
Surgical approaches | |||
VATS | 6 (66.67) | 4 (57.14) | >0.99 |
Thoracotomy | 3 (33.33) | 3 (42.86) | |
Pathological diagnosis | |||
Adeno-carcinoma | 5 (55.56) | 6 (85.71) | 0.31 |
Perioperative analgesia | |||
TEA | 5 (55.56) | 5 (71.43) | 0.63 |
Intraoperative conditions | |||
Operation time (min) | 152.0 [85.0, 215.5] | 160.0 [75.0, 325.0] | 0.59 |
OLV duration (min) | 110.0 [80.0, 132.5] | 72.5 [108.8, 213.8] | 0.06 |
OLV VT (mL/kg) | 5.37 [5.05, 6.41] | 5.21 [4.24, 6.66] | 0.61 |
PEEP (cmH2O) | 5 [3, 5] | 5 [5, 6] | 0.13 |
Fluid administration (mL/kg) | 19.30 [15.44, 24.40] | 21.74 [10.00, 447.95] | 0.76 |
Blood transfusion | 1 (11.11) | 0 | >0.99 |
Total RALE | 33 [31, 36] | 32 [30, 34] | 0.42 |
RALES | 12 [10, 16] | 20 [16, 20] | 0.03 |
RALENS | 21 [20, 24] | 12 [10, 16] | <0.001 |
RRALE | 0.60 [0.40, 0.74] | 1.18 [1.13, 2.00] | <0.001 |
Data are presented as medians [interquartile ranges] or n (%). RRALE, RALE score ratio, RRALE = RALES/RALENS; RALE, radiographic assessment of lung edema; RALES, RALE score of surgical side; RALENS, RALE score of non-surgical side; BMI, body mass index; APACHE, acute physiology and chronic health evaluation; COPD, chronic obstructive pulmonary disease; VATS, video assisted thoracoscopic surgery; TEA, thoracic epidural analgesia; OLV, one-lung ventilation; VT, tidal volume; PEEP, positive end-expiratory pressure.
Patient characteristics
No significant differences were observed in demographic characteristics, including age, sex, body mass index (BMI), occupation, history of smoking and alcohol consumption, medical history, APACHE II score at ICU admission, preoperative therapy (chemotherapy, radiotherapy, or targeted therapy) or anticoagulation or antiplatelet therapy between the two groups (Table 1). Four patients (44.44%) in the RRALE <1 group but none in the RRALE ≥1 group had a history of thoracic surgery (P=0.09); history of nonthoracic surgery was similar between the two groups (44.44% vs. 42.86%, P>0.99) (Table 1). No other significant differences were observed between the two groups in terms of operative side, types of surgery, surgical approaches, pathological diagnosis, perioperative analgesia, operation time, OLV duration, OLV tidal volume, PEEP, intraoperative fluid administration, or blood transfusion (Table 1).
Outcomes
The primary outcome was 90-day all-cause mortality. Approximately 66.67% of the patients died within 90 days in the RRALE <1 group; none of the patients in the RRALE ≥1 group died (P=0.01) (Table 2). No statistically significant difference was observed in 28-day in-hospital mortality between patients with RRALE <1 and RRALE ≥1 (22.22% vs. 0%, P=0.48) (Table 2). There were also no significant differences found in ICU LOS or hospital LOS between the two groups (12 vs. 7 days, P=0.34 and 28 vs. 20 days, P=0.36) (Table 2). However, survival at 6 months was significantly lower in the RRALE <1 group (33.33%) than in the RRALE ≥1 group (100.00%) [hazard ratio (HR): 8.62; 95% confidence interval (CI): 1.71–43.46; log-rank test: P=0.009], as shown in Figure 2.
Table 2
Variables | RRALE <1 (n=9) | RRALE ≥1 (n=7) | P value |
---|---|---|---|
Primary outcome | |||
90-day mortality | 6 (66.67) | 0 | 0.01 |
Secondary outcomes | |||
In hospital 28-day mortality | 2 (22.22) | 0 | 0.48 |
ICU LOS (days) | 12 [7, 18] | 7 [4, 14] | 0.34 |
Hospital LOS (days) | 28 [19, 40] | 20 [16, 28] | 0.36 |
Preoperative pulmonary function | |||
MVV% | 208.2 [143.4, 274.1] | 357.5 [274.9, 437.9] | 0.11 |
VC max% | 76.9 [66.6, 89.0] | 86.3 [27.0, 91.6] | 0.46 |
FVC% | 79.1 [70.0, 91.8] | 91.9 [85.0, 101.3] | 0.15 |
FEV1% | 76.9 [65.2, 91.1] | 93.5 [75.2, 114.1] | 0.28 |
P/F ratio (mmHg) | 410.0 [381.0, 414.0] | 398.0 [364.3, 452.8] | 0.93 |
PaCO2 (mmHg) | 40 [34, 44] | 39 [36, 43] | >0.99 |
Postoperative fluid balance | |||
Day 1 (mL/kg) | 6.09 [3.30, 12.67] | 1.53 [−1.86, 6.50] | 0.25 |
Day 2 (mL/kg) | 5.22 [−4.42, 10.43] | 5.66 [−7.17, 10.16] | >0.99 |
Day 3 (mL/kg) | 4.91 [−3.32, 10.43] | 2.32 [−2.63, 4.71] | 0.47 |
3 days total (mL/kg) | 16.76 [1.91, 23.44] | 9.29 [0.38, 19.64] | 0.61 |
Onset of hypoxemia after operation (days) | 6 [3, 8] | 3 [2, 4] | 0.28 |
Worst P/F ratio in ICU (mmHg) | 97.0 [84.0, 119.0] | 113.0 [95.0, 155.0] | 0.24 |
Lowest PaCO2 in ICU (mmHg) | 33.0 [30.8, 39.6] | 40.0 [31.5, 45.5] | 0.35 |
Respiratory rate (bpm) | 30.0 [24.5, 35.5] | 24.0 [20.0, 33.0] | 0.26 |
Body temperature | |||
Maximum (℃) | 38.7 [38.3, 38.9] | 37.6 [37.0, 37.8] | 0.03 |
≥38.5 ℃ | 7 (77.78) | 1 (14.29) | 0.04 |
Invasive MV | |||
Number | 7 (77.78) | 6 (85.71) | >0.99 |
Duration (h) | 167.5 [97.3, 556.3] | 35.5 [21.5, 164.0] | 0.11 |
NMBA using | 4 (44.44) | 1 (14.29) | 0.31 |
Data are presented as medians [interquartile ranges] or n (%). RRALE, RALE score ratio, RRALE = RALES/RALENS; RALE, radiographic assessment of lung edema; RALES, RALE score of surgical side; RALENS, RALE score of non-surgical side; ICU, intensive care unit; LOS, length of stay; MVV, maximal voluntary ventilation; VC max, maximum vital capacity; FVC, forced vital capacity; FEV1, forced expiratory volume in 1 second, FEV1% = (FEV1/FVC) × 100%; P/F, PaO2/FiO2; MV, mechanical ventilation; NMBA, neuromuscular blocking agent.
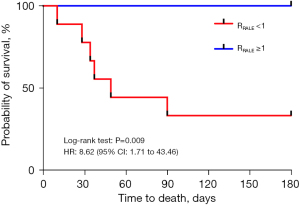
Respiratory parameters and postoperative conditions
No significant difference was observed in preoperative pulmonary function or the P/F ratio before surgery in either group (Table 2).
Postoperative fluid management was similar in both groups, with no significant differences in postoperative 3-day total fluid balance (P=0.61) (Table 2). The median duration of postoperative hypoxemia onset was similar in both groups [6 (3–8) vs. 3 (2–4) days, P=0.28] (Table 2). No significant differences were observed in the worst P/F ratio (97 vs. 113 mmHg, P=0.24), lowest PaCO2 (33 vs. 40 mmHg, P=0.35), or respiratory rates (30 vs. 24 bpm, P=0.26) between the two groups. Compared to those in the RRALE ≥1 group, the patients in the RRALE <1 group had a significantly higher maximum body temperature after surgery (38.7 vs. 37.6 ℃, P=0.03). Additionally, the RRALE <1 group comprised more patients with a body temperature ≥38.5 ℃ than the RRALE ≥1 group (77.78% vs. 14.29%, P=0.04) (Table 2).
No significant differences were noted in the number of patients requiring invasive MV between the two groups (77.78% vs. 85.71%, P>0.99), and the total invasive MV time did not differ significantly [167.5 (97.3–556.3) vs. 35.5 (21.5–164.0) h, P=0.11]. Additionally, the proportion of patients who needed neuromuscular blocking agents (NMBAs) was similar between the two groups (44.44% vs. 14.29%, P=0.31) (Table 2).
EIT monitoring
Due to limited conditions, EIT was not performed for all patients; five patients in the RRALE <1 group and four patients in the RRALE ≥1 group underwent EIT monitoring. The EIT parameters are reported in Table 3. Although the severe injury side differed between the two groups, there were no significant difference in the ratio of ventilation between the surgical and nonsurgical lungs in relation to EIT monitoring (IMS-NS: −0.43 vs. −0.38, P=0.73). More ventilation occurred on the nonsurgical side, and its distribution was similar in both groups.
Table 3
Variables | RRALE <1 (n=5) | RRALE ≥1 (n=4) | P value |
---|---|---|---|
TVS/TVNS | 0.40 (0.12, 0.60) | 0.46 (0.30, 0.66) | 0.56 |
TVS/TVTotal | 0.29 (0.10, 0.38) | 0.31 (0.24, 0.40) | 0.56 |
IMS-NS | −0.43 (−0.79, −0.20) | −0.38 (−0.53, −0.20) | 0.73 |
Data are presented as medians (interquartile ranges). EIT, electrical impedance tomography; RRALE, RALE score ratio, RRALE = RALES/RALENS; RALE, radiographic assessment of lung edema; RALES, RALE score of surgical side; RALENS, RALE score of non-surgical side; TV, tidal ventilation; TVS, the TV within the surgical; TVNS, nonsurgical side of the lungs; IMS-NS, imbalance coefficient.
Discussion
In the present study, patients with postoperative delayed ALI on the nonsurgical side (RRALE <1 group) exhibited a high mortality rate. The RRALE <1 group had a median time of 6 days for the onset of severe hypoxemia (ALI) after surgery, whereas the RRALE ≥1 group had a median time of 3 days. Although nonsurgical-side lung exudation increased in the RRALE <1 group, it remained the dominant area of EIT ventilation.
Our findings reveal that patients with severe lung injury on the non-surgical side are at a higher risk of mortality. The RRALE <1 group exhibited a considerably higher 90-day all-cause mortality rate compared to the RRALE ≥1 group. Furthermore, the survival rate at 6 months was significantly lower in the RRALE <1 group, suggesting that the severity of lung injury on the non-surgical side is closely linked to long-term outcomes. These results emphasize the importance of considering both surgical and non-surgical lung sides in the assessment of PALI after lung cancer surgery.
Although there was no significant difference, patients in the RRALE <1 group displayed a trend toward poorer preoperative pulmonary function. This suggests that preoperative respiratory function might influence the development of PALI. Additionally, patients in the RRALE <1 group had higher maximum body temperatures after surgery and were more likely to experience fever ≥38.5 ℃. While the exact relationship between fever and PALI development requires further investigation, it could be an indicator of systemic inflammatory response and potential complications.
Rather than a single risk factor, delayed PALI is likely that a cascade of deleterious events leads to alveolar epithelial and capillary endothelial injury, such as gastric aspiration, bronchopleural fistula, anastomotic fistula, or bronchopneumonia (19). In our study, the median time of presentation for severe hypoxemia after surgery was 6 days in the RRALE <1 group. Most cases were delayed or secondary after surgery. Perioperative management, such as analgesia, OLV, and fluid admission, was similar in the two groups. Therefore, delayed lung injury on the nonsurgical side correlates very little with surgically induced injury. The combined chest X-ray or CT films with EIT images showed that some patients with lung injury on the nonsurgical side had strong ventilation on the injured side, and radiological signs showed unilateral diffuse infiltrates on the nonsurgical lung (Figure 3). Based on the good ventilation at the deep injured lung, we speculated that patient self-inflicted lung injury (P-SILI) might be one of the reasons for this type of PALI after lung cancer surgery. Several studies have demonstrated that vigorous spontaneous breathing exacerbates preexisting lung injury (20-23). However, the lack of monitoring of ventilator variables in non-intubated patients has resulted in limited evidence for P-SILI. Overall, diagnosis and management of P-SILI remains controversial (24). Excessive transpulmonary energy delivery and uneven ventilation distribution are among the multiple mechanisms contributing to P-SILI (25).
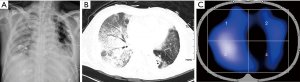
As described in the literature, causes of the high respiratory drive include hypercapnia (26), metabolic acidosis (22), hypoxemia (27), fever, pain, anxiety (28,29), and pulmonary or systemic inflammation (22,23,30). In our study, patients in the RRALE <1 group exhibited factors contributing to elevated respiratory drive including high-grade fever and severe hypoxemia. Most of the inhaled gas entered the nonsurgical side with good compliance, resulting in overinflation and eventual injury to this side of the lung. Although there was no significant difference, these patients displayed a trend toward poorer preoperative pulmonary function and approximately 44.44% had a history of thoracic surgery; thus, they might have preexisting lesions, rendering the lung more vulnerable to injury under high respiratory drive than the RRALE ≥1 group.
Nevertheless, the present study has several limitations. First, as the incidence of PALI after lung cancer surgery has been greatly reduced in recent years, the number of patients enrolled in this study was too small for further statistical analysis, which had a certain impact on the results. We were unable to perform further risk factor analysis. Second, due to previous conditions, certain respiratory parameters, such as driving pressure, could not be monitored during autonomous breathing, and only a small number of patients were monitored for esophageal pressure. Detailed ventilation parameters could not be compared between the two groups due to incomplete EIT data collection and CT screening. Thus, the causal relationship between ventilation and lung injury remains unclear. Third, although we employed a blinded method to mitigate subjective bias in the RALE score, differentiation of lung edema from atelectasis, pleural effusion or pulmonary infection on X-ray images remained a challenge. Furthermore, obesity and subcutaneous emphysema may affect the application of the RALE score. However, we routinely perform thoracentesis to drain moderate to massive pleural effusion, which can effectively mitigate the impact of effusion. Fourth, grouping patients based on RRALE may not be entirely accurate when lung injury occurs on both the surgical and nonsurgical sides.
Conclusions
The study showed increased postoperative mortality in patients with delayed ALI on the nonsurgical side after lung cancer surgery. The classification of PALI into distinct subtypes based on injury sides, along with the utilization of advanced techniques like EIT, offers new insights into this complex condition. Respiratory effort in these patients should be closely monitored and controlled to minimize the risk of lung injury. The findings emphasize the importance of comprehensive assessment and suggest directions for further studies with larger cohorts and longitudinal designs to validate and expand upon these observations. The insights gained from this study have the potential to guide interventions and strategies aimed at reducing PALI incidence and improving patient outcomes after lung cancer surgery.
Acknowledgments
The authors thank all the subjects for their participation in this study.
Funding: This work was supported by
Footnote
Reporting Checklist: The authors have completed the STROBE reporting checklist. Available at https://jtd.amegroups.com/article/view/10.21037/jtd-23-822/rc
Data Sharing Statement: Available at https://jtd.amegroups.com/article/view/10.21037/jtd-23-822/dss
Peer Review File: Available at https://jtd.amegroups.com/article/view/10.21037/jtd-23-822/prf
Conflicts of Interest: All authors have completed the ICMJE uniform disclosure form (available at https://jtd.amegroups.com/article/view/10.21037/jtd-23-822/coif). The authors have no conflicts of interest to declare.
Ethical Statement: The authors are accountable for all aspects of the work in ensuring that questions related to the accuracy or integrity of any part of the work are appropriately investigated and resolved. The study was conducted in accordance with the Declaration of Helsinki (as revised in 2013). The ethics review board of Zhongshan Hospital of Fudan University approved the study protocol (No. B2021-465). All participants or their legal representatives were contacted through telephone calls and signed informed consent.
Open Access Statement: This is an Open Access article distributed in accordance with the Creative Commons Attribution-NonCommercial-NoDerivs 4.0 International License (CC BY-NC-ND 4.0), which permits the non-commercial replication and distribution of the article with the strict proviso that no changes or edits are made and the original work is properly cited (including links to both the formal publication through the relevant DOI and the license). See: https://creativecommons.org/licenses/by-nc-nd/4.0/.
References
- Licker M, Fauconnet P, Villiger Y, et al. Acute lung injury and outcomes after thoracic surgery. Curr Opin Anaesthesiol 2009;22:61-7. [Crossref] [PubMed]
- Grichnik KP, D’Amico TA. Acute lung injury and acute respiratory distress syndrome after pulmonary resection. Semin Cardiothorac Vasc Anesth 2004;8:317-34. [Crossref] [PubMed]
- Licker M, de Perrot M, Spiliopoulos A, et al. Risk factors for acute lung injury after thoracic surgery for lung cancer. Anesth Analg 2003;97:1558-65. [Crossref] [PubMed]
- Eichenbaum KD, Neustein SM. Acute lung injury after thoracic surgery. J Cardiothorac Vasc Anesth 2010;24:681-90. [Crossref] [PubMed]
- Leng X, Onaitis MW, Zhao Y, et al. Risk of Acute Lung Injury after Esophagectomy. Semin Thorac Cardiovasc Surg 2022;34:737-46. [Crossref] [PubMed]
- Lohser J, Slinger P. Lung Injury After One-Lung Ventilation: A Review of the Pathophysiologic Mechanisms Affecting the Ventilated and the Collapsed Lung. Anesth Analg 2015;121:302-18. [Crossref] [PubMed]
- Kaufmann K, Heinrich S. Minimizing postoperative pulmonary complications in thoracic surgery patients. Curr Opin Anaesthesiol 2021;34:13-9. [Crossref] [PubMed]
- Durkin C, Schisler T, Lohser J. Current trends in anesthesia for esophagectomy. Curr Opin Anaesthesiol 2017;30:30-5. [Crossref] [PubMed]
- Okuda J, Suzuki T, Wakaizumi K, et al. Effects of Thoracic Epidural Anesthesia on Systemic and Local Inflammatory Responses in Patients Undergoing Lung Cancer Surgery: A Randomized Controlled Trial. J Cardiothorac Vasc Anesth 2022;36:1380-6. [Crossref] [PubMed]
- Della Rocca G, Coccia C. Acute lung injury in thoracic surgery. Curr Opin Anaesthesiol 2013;26:40-6. [Crossref] [PubMed]
- Park BJ. Respiratory failure following pulmonary resection. Semin Thorac Cardiovasc Surg 2007;19:374-9. [Crossref] [PubMed]
- Frerichs I, Amato MB, van Kaam AH, et al. Chest electrical impedance tomography examination, data analysis, terminology, clinical use and recommendations: consensus statement of the TRanslational EIT developmeNt stuDy group. Thorax 2017;72:83-93. [Crossref] [PubMed]
- Spinelli E, Mauri T, Fogagnolo A, et al. Electrical impedance tomography in perioperative medicine: careful respiratory monitoring for tailored interventions. BMC Anesthesiol 2019;19:140. [Crossref] [PubMed]
- Lipes J, Bojmehrani A, Lellouche F. Low Tidal Volume Ventilation in Patients without Acute Respiratory Distress Syndrome: A Paradigm Shift in Mechanical Ventilation. Crit Care Res Pract 2012;2012:416862. [Crossref] [PubMed]
- Gao S, Zhang Z, Brunelli A, et al. The Society for Translational Medicine: clinical practice guidelines for mechanical ventilation management for patients undergoing lobectomy. J Thorac Dis 2017;9:3246-54. [Crossref] [PubMed]
- Warren MA, Zhao Z, Koyama T, et al. Severity scoring of lung oedema on the chest radiograph is associated with clinical outcomes in ARDS. Thorax 2018;73:840-6. [Crossref] [PubMed]
- Jabaudon M, Audard J, Pereira B, et al. Early Changes Over Time in the Radiographic Assessment of Lung Edema Score Are Associated With Survival in ARDS. Chest 2020;158:2394-403. [Crossref] [PubMed]
- Trepte CJ, Phillips CR, Solà J, et al. Electrical impedance tomography (EIT) for quantification of pulmonary edema in acute lung injury. Crit Care 2016;20:18. [Crossref] [PubMed]
- Ware LB. Pathophysiology of acute lung injury and the acute respiratory distress syndrome. Semin Respir Crit Care Med 2006;27:337-49. [Crossref] [PubMed]
- Beloncle F, Mercat A. Approaches and techniques to avoid development or progression of acute respiratory distress syndrome. Curr Opin Crit Care 2018;24:10-5. [Crossref] [PubMed]
- Grieco DL, Menga LS, Eleuteri D, et al. Patient self-inflicted lung injury: implications for acute hypoxemic respiratory failure and ARDS patients on non-invasive support. Minerva Anestesiol 2019;85:1014-23. [Crossref] [PubMed]
- Vaporidi K, Akoumianaki E, Telias I, et al. Respiratory Drive in Critically Ill Patients. Pathophysiology and Clinical Implications. Am J Respir Crit Care Med 2020;201:20-32. [Crossref] [PubMed]
- Spinelli E, Mauri T, Beitler JR, et al. Respiratory drive in the acute respiratory distress syndrome: pathophysiology, monitoring, and therapeutic interventions. Intensive Care Med 2020;46:606-18. [Crossref] [PubMed]
- Yoshida T, Fujino Y, Amato MB, et al. Fifty Years of Research in ARDS. Spontaneous Breathing during Mechanical Ventilation. Risks, Mechanisms, and Management. Am J Respir Crit Care Med 2017;195:985-92. [Crossref] [PubMed]
- Silva PL, Ball L, Rocco PRM, et al. Physiological and Pathophysiological Consequences of Mechanical Ventilation. Semin Respir Crit Care Med 2022;43:321-34. [Crossref] [PubMed]
- Mitrouska J, Xirouchaki N, Patakas D, et al. Effects of chemical feedback on respiratory motor and ventilatory output during different modes of assisted mechanical ventilation. Eur Respir J 1999;13:873-82. [Crossref] [PubMed]
- Duffin J. Measuring the ventilatory response to hypoxia. J Physiol 2007;584:285-93. [Crossref] [PubMed]
- Tipton MJ, Harper A, Paton JFR, et al. The human ventilatory response to stress: rate or depth? J Physiol 2017;595:5729-52. [Crossref] [PubMed]
- Raux M, Ray P, Prella M, et al. Cerebral cortex activation during experimentally induced ventilator fighting in normal humans receiving noninvasive mechanical ventilation. Anesthesiology 2007;107:746-55. [Crossref] [PubMed]
- Dempsey JA, Smith CA. Pathophysiology of human ventilatory control. Eur Respir J 2014;44:495-512. [Crossref] [PubMed]