Performance of radial endobronchial ultrasound for peripheral pulmonary lesions without automation technology in tuberculous endemic region: real-world experience in a single institution over 6 years
Highlight box
Key findings
• The diagnostic yield of radial endobronchial ultrasound (rEBUS) without advanced automation technology in a real-world setting was 78.8% [95% confidence interval (CI): 75.1–82.1%] with direct bronchus sign and concentric orientation being the independent predictors for conclusive procedure.
• The overall diagnostic accuracy of rEBUS for malignant and tuberculous disease was 90.64% (95% CI: 87.74–93.04%) and 93.03% (95% CI: 90.44–95.10%) respectively.
What is known and what is new?
• rEBUS procedure is commonly performed with automation technology, such as virtual bronchoscopic navigation, electromagnetic navigational bronchoscopy and most recently, robotic bronchoscopy systems.
• In this study, we demonstrate that rEBUS without advanced automation technology is still feasible with equivalent diagnostic yield.
What is the implication and what should change now?
• rEBUS without automation technology remains relevant and useful in this era.
• In tuberculous endemic regions, rEBUS provides a rapid and safe histological and microbiological proof of disease.
Introduction
Peripheral pulmonary lesions (PPLs) can result from various causes, ranging from benign to malignant causes. Major guidelines recommend tissue sampling to establish a diagnosis for PPL with indeterminate to high risk for malignancy, as early detection of lung cancer is associated with better overall survival rates (1-3). Moreover, in regions with high tuberculosis incidence, PPL caused by tuberculosis presents a significant diagnostic challenge, as it can be difficult to distinguish from malignant nodules using conventional imaging alone (4,5). In the absence of positive sputum examination for Mycobacterium, many patients may receive empirical tuberculosis treatment since early diagnosis and treatment of tuberculosis is critical for preventing disease spread from a public health perspective—however, this may potentially also result in delay lung cancer diagnosis (4). Therefore, biopsy is essential in our region, to accurately determine the underlying cause of PPL to allow prompt initiation of appropriate treatment, particularly for tuberculosis and lung cancer.
In our region, the transthoracic approach via computed tomography (CT) thorax guidance is the most commonly used method for PPL biopsy which has a high diagnostic yield. However, meta-analyses have shown that it comes at the expense of a higher complication rate when compared to bronchoscopic biopsy (6). In contrast, radial endobronchial ultrasound (rEBUS) is a minimally invasive guided bronchoscopic technique that provides real-time verification of PPL location, allowing for accurate and precise biopsy without additional risk when compared to conventional bronchoscopy. Meta-analyses have revealed a diagnostic yield of approximately 70.6% for the rEBUS procedure, accompanied by a very low overall complication rate of only 2.8% (7). Over the years, rEBUS procedure has continued to evolve with the addition of more automation technology to enhance its performance, such as virtual bronchoscopic navigation (VBN) for pre-procedural planning, electromagnetic navigational bronchoscopy (ENB) for real-time tracking, and most recently, the use of robotic bronchoscopy systems (8-10). However, these advanced technologies are not readily available in all parts of the world.
Our center started performing rEBUS procedures in 2016 without advanced automation technology, particularly employing manual bronchial branch tracing for navigation and airway maneuvring using conventional flexible bronchoscopy. In this study, our main objectives are to evaluate the overall diagnostic yield and complication rate of our rEBUS procedures over 6 years and to identify factors associated with a better diagnostic yield. Additionally, as a subset analysis, we aim to describe the diagnostic accuracy (sensitivity, specificity, and predictive values) of rEBUS in malignant and tuberculous PPL and assess the impact of changes in rEBUS practices on service delivery during the study period. We present this article in accordance with the STROBE reporting checklist (available at https://jtd.amegroups.com/article/view/10.21037/jtd-23-979/rc).
Methods
Study design & setting
This is a retrospective chart review of all adult patients undergoing rEBUS-guided transbronchial biopsy for PPL in the respiratory care unit in Sarawak General Hospital, Malaysia over 6 years duration (October 1, 2016 to December 31, 2022). Patients with endobronchial lesions during initial airway examination were excluded. The study was conducted in accordance with the Declaration of Helsinki (as revised in 2013) (11). The study protocol was approved by the medical research and ethics committee of Ministry of Health Malaysia [NMRR-ID-23-00520-LMM (IIR)] and individual consent for this retrospective analysis was waived. In Malaysia, the estimated incidence rate of tuberculosis is 97 per 100,000 population, with a mortality rate of 6 per 100,000 population (12).
rEBUS procedure
Pre-procedural planning
CT scan of at least 2 mm thickness and below were performed for all patients from our center. Manual bronchial branch tracing method is then performed by analyzing subsegmental airway anatomy in multiplanar reconstruction from the CT scan in axial, coronal, and sagittal planes. The bronchus sign and bifurcations that led into the target lesion were analyzed meticulously. The classification of the bronchus sign is as follows: type A, when the airway is observed to lead directly into the target; type B, when the airway is located adjacent to the target; and type C, for target lesions devoid of a leading airway (13). Based on this analysis, we drew schematic diagrams of the planned road map representing the actual bronchoscopic vision of the segmental airway leading into the target lesion by hand. No additional navigational software was used in this study to assist with procedural planning (14). The characteristics and measurements of the target lesion were recorded by assessing the largest diameter of the target PPL on axial CT scan in lung window reconstruction. The target-to-pleural distance was determined by measuring the distance from the center of the target to the closest point on the parietal or visceral pleura in the direction of the biopsy (15).
Bronchoscopy and rEBUS procedure
The procedures were performed either by consultant pulmonologists with experience of flexible and rigid bronchoscopy, or by pulmonology fellows under direct supervision. Flexible bronchoscopies were performed under conscious sedation or total intravenous anesthesia as per unit protocol. Decision for advanced airways (i.e., rigid bronchoscope, endotracheal intubation, and laryngeal mask airway) was at the discretion of the bronchoscopist after considering the risk and benefit of the procedure to the patient. All patients had signed and dated informed consent prior to procedures.
Various flexible conventional (BF-1TH190, BF-H190, Olympus Medical, Tokyo, Japan) and thin bronchoscopes (BF-P190, BF-MP190-F; Olympus Medical) were used for the procedures. Olympus rEBUS system (EU-ME2 Premier Plus) was used in all cases. After ruling out endobronchial lesions, the 20 Hz 2.0- or 1.7-mm rEBUS probe (UM-S20-20R, UM-S20-17S; Olympus Medical) with or without a guide sheath (SG-201C, SG-200C; Olympus Medical) was inserted into the pre-planned target segment via the bronchoscope working channel. Upon identification of the lesion via rEBUS, the guide sheath was locked and rEBUS probe was removed. In selected cases, the guide sheath was not used, and subsequent placement of biopsy tools was guided by single planar fluoroscopy.
For transbronchial forceps biopsy, a standard fenestrated cup flexible forceps (FB231-D, FB233-D; Olympus Medical) was introduced into the guide sheath, and 5–10 biopsies were taken during each round of the procedure. For cryobiopsy, either the 1,050 mm 1.9 mm reusable cryoprobe or the single-used flexible cryoprobe (1.1 or 1.7 mm) was used in conjunction with the ERBECRYO®2 system (ERBE, Medizintechnik, Tübingen, Germany). An endobronchial balloon (B5-2C; Olympus Medical) was placed at the target segmental bronchi if advanced airways were used during the procedure. The cryoprobe was subsequently inserted into the guide sheath, activated and removed en bloc with the bronchoscope from the patient. The pre-placed balloon was inflated immediately upon removal of bronchoscope. Tissue from cryobiopsy was thawed and retrieved in normal saline from the cryoprobe. After biopsy, normal saline was instilled into the target segment via guide sheath and aspirated for microbiological or cytological examination in cases where infective causes were suspected. Rapid on-site examination services were not available in our institution. Chest radiographs were performed to look for pneumothorax 2 hours post-procedure. Figure 1 summarizes our work procedures.
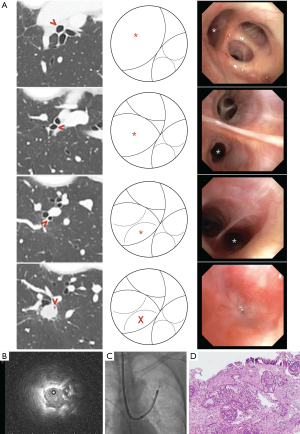
Definition
The procedure was considered to be conclusive if histological, microbiological, or cytological studies provided definitive results, allowing implementation of specific treatment. Lesions with inflammatory findings on biopsy would be considered as conclusive only if the lesion demonstrated radiological stability for at least 6 months during CT surveillance. Procedures were considered to be inconclusive if results failed to provide a definitive answer to the presenting clinical problem, requiring further intervention or biopsy.
Statistical analysis
SPSS (version 20; Chicago, IL, USA) was used for data analysis. Normality was assessed with the Shapiro-Wilk test. Results were presented as mean ± standard deviation (SD) for normally distributed variables, and as median and interquartile range (IQR) for non-normally distributed variables. Categorical data were expressed as absolute numbers and percentages, and compared using Pearson’s Chi-squared test or Fisher’s exact test. Independent sample t-tests and Mann-Whitney tests were used to compare normally and non-normally distributed variables between groups, respectively. The overall diagnostic yield for rEBUS is determined by dividing the total number of conclusive procedures by the total number of rEBUS procedures, with the result expressed as a percentage along with a 95% confidence interval (CI). Predictive factors for conclusive rEBUS results were assessed using multivariate logistic regression with variables having P<0.20 on univariate analysis. In a subgroup analysis, we assess the diagnostic accuracy (sensitivity, specificity, and predictive values) of rEBUS in malignant and tuberculous PPLs. Subjects without follow-up data will be excluded for a more lenient diagnostic accuracy analysis. To establish a stricter and more rigorous minimum sensitivity threshold, we conducted a diagnostic accuracy analysis encompassing all subjects, with those lacking follow-up data classified as “false negatives”. Conversely, to determine the maximum sensitivity threshold, we classified subjects without follow-up data as “true negatives” (16). Diagnostic accuracy, sensitivity, specificity, and predictive values were then calculated based on standard definitions. A P value of <0.05 was considered significant.
Results
During the study period, a total of 558 procedures were performed. Seven cases were excluded due to the presence of visible endobronchial lesions during initial scouting bronchoscopy. Hence, the final number of procedures available for analysis was 551.
Baseline demographic and target lesions characteristic
In our study, the majority (65.0%) of subjects were male, with an overall median age of 62.0 (IQR, 54.0–70.0) years. The median target lesion size was 2.70 (IQR, 2.10–3.70) cm, and the median target to pleural distance was 2.30 (IQR, 1.64–3.25) cm. The majority of lesions (57.7%) were located in the upper lobes of the lung. Approximately 8 out of 10 lesions (84.2%) were recorded as having a “within” rEBUS orientation (Table 1).
Table 1
Characteristics | Value |
---|---|
Patient | |
Age (years) | 62.0 (54.0–70.0) |
Gender | |
Male | 358 (65.0) |
Female | 193 (35.0) |
Target lesions | |
Size (cm) | 2.70 (2.10–3.70) |
Target to pleural distance (cm) | 2.30 (1.64–3.25) |
Bronchus sign | 464 (84.2) |
Location | |
Right upper lobe | 188 (34.1) |
Right middle lobe | 43 (7.8) |
Right lower lobe | 97 (17.6) |
Left upper lobe | 130 (23.6) |
Lingular | 35 (6.4) |
Left lower lobe | 58 (10.5) |
rEBUS orientation | |
Concentric | 255 (46.3) |
Non-concentric | 296 (53.7) |
Procedure | |
Procedural time (min) | 40.0 (30.0–50.0) |
Access | |
Natural airway | 335 (60.8) |
Artificial airway | 216 (39.2) |
Sedation | |
Conscious sedation | 323 (58.6) |
Total intravenous anesthesia | 228 (41.4) |
Fluoroscopy | 456 (82.8) |
Bronchoscope | |
Conventional | 492 (89.3) |
Thin | 59 (10.7) |
Guide sheath | 448 (81.3) |
Biopsy method | |
Conventional | 416 (75.5) |
Cryobiopsy | 135 (24.5) |
Number of forceps pass | 10.0 (9.0–10.0) |
Number of cryobiopsy pass | 2.0 (1.0–3.0) |
Cryobiopsy activation (s) | 5.5 (4.0–8.0) |
Data are presented as median (IQR) or n (%). rEBUS, radial endobronchial ultrasound; IQR, interquartile range.
Baseline procedural characteristic
The median procedural duration was 40.0 (IQR, 30.0–50.0) minutes, determined from the initiation of anesthetic sedation to the conclusion of the procedure. Almost two-thirds of the procedures were performed without an artificial airway under conscious sedation. Fluoroscopy was utilized in the majority (82.8%) of cases. Standard bronchoscope with guide sheath was used in majority of procedures. Cryobiopsy was employed in 24.5% of cases.
Overall diagnostic yield & complication rate
In our cohort, 434 out of 551 cases attained a conclusive diagnosis, resulting in an overall diagnostic yield of 78.8% (95% CI: 75.1–82.1%). Six pneumothoraxes (1.1%) were recorded. Mild bleeding occurred in 22.1% cases, while moderate bleeding occurred in 5.3%. Cryobiopsy was associated with a higher frequency of bleeding episodes than conventional sampling methods (41.5% vs. 22.8%, P<0.001). However, there were no severe bleeding episodes recorded in our cohort.
Almost two-thirds of conclusive cases were malignant—the commonest being adenocarcinoma lung at 42.4% followed by squamous cell carcinoma of lung at 6.9%. The remaining were benign in nature with 24.7% being tuberculous. The complete histopathological analyses can be found in Tables S1,S2.
Diagnostic accuracy of rEBUS for malignant and tuberculous disease
Among the 117/551 (21.2%) of cases with inconclusive histology, 68 had complete follow-up data while 49 had incomplete follow-up data for final diagnosis conclusion. Hence, a total number of 502 subjects were available for the more lenient diagnostic accuracy analysis (Tables S3-S6).
The prevalence of malignant disease in our cohort was 63.5% (319/502). The sensitivity of rEBUS for malignant disease was 85.27% (95% CI: 80.89–88.97%) with a negative predictive value of 79.57% (95% CI: 74.94–83.53%)—this translates to an overall diagnostic accuracy of 90.64% (95% CI: 87.74–93.04%) for malignant diseases. When encompassing all subjects, the minimum diagnostic accuracy, sensitivity, and negative predictive value of rEBUS for malignant disease was 82.58% (95% CI: 79.15–85.65%), 73.91% (95% CI: 69.11–78.33%), and 65.59% (95% CI: 61.61–69.36%), respectively.
For tuberculous disease, the prevalence in our cohort was 24.7%. The overall diagnostic accuracy of rEBUS for tuberculous disease was 93.03% (95% CI: 90.44–95.10%). The sensitivity and negative predictive value were 71.77% (95% CI: 62.99–79.49%) and 91.53% (95% CI: 89.08–93.46%), respectively. When encompassing all subjects, the minimum diagnostic accuracy, sensitivity, and negative predictive value of rEBUS for tuberculous disease was 84.75% (95% CI: 81.48–87.65%), 51.45% (95% CI: 43.74–59.10%), and 81.82% (95% CI: 79.42–83.99%), respectively.
Factors predicting conclusive diagnostic yield
In our cohort, female gender was associated with higher diagnostic yield (83.9% vs. 76.0%, P=0.029) along with type A bronchus sign and a concentric rEBUS orientation (Table S7). However, in multivariate analysis, only direct bronchus sign [adjusted odds ratio (adj. OR): 2.268; 95% CI: 1.335–3.850; P=0.002] and concentric rEBUS orientation [adj. OR: 3.426; 95% CI: 2.081–5.641; P<0.001] were independent factors predicting higher diagnostic yield (Table 2).
Table 2
Variables | OR (95% CI), P value | Adj. OR (95% CI), P value |
---|---|---|
Female | 1.652 (1.049–2.603), 0.030 | 1.587 (0.987–2.551), 0.057 |
Artificial airway | 1.141 (0.748–1.740), 0.541 | |
Total intravenous anesthesia | 1.066 (0.703–1.615), 0.765 | |
Less than 5 airway generation | 1.334 (0.730–2.434), 0.349 | |
Upper lobe target | 1.221 (0.810–1.841), 0.340 | |
Target less than 2 cm | 0.832 (0.502–1.377), 0.474 | |
Bronchus sign A | 3.136 (1.920–5.120), <0.001 | 2.268 (1.335–3.850), 0.002 |
Solid target | 0.907 (0.556–1.477), 0.694 | |
Fluoroscopy | 0.843 (0.482–1.475), 0.549 | |
Concentric rEBUS | 3.910 (2.432–6.286), <0.001 | 3.426 (2.081–5.641), <0.001 |
Cryobiopsy | 1.422 (0.859–2.353), 0.171 | 1.465 (0.845–2.540), 0.174 |
Thin bronchoscope | 2.139 (0.945–4.843), 0.068 | 1.237 (0.436–3.507), 0.689 |
Guide sheath | 0.632 (0.355–1.126), 0.119 | 0.618 (0.295–1.293), 0.202 |
OR, odds ratio; CI, confidence interval; adj. OR, adjusted OR; rEBUS, radial endobronchial ultrasound.
Changes of rEBUS performance and practice over time
Dividing our overall cohort (n=551) into five batches (n=110/batch), a significant improving trend of diagnostic yield was found over the years (P<0.001). Median procedure time also reduced over the years significantly [Batch 1: 40.0 (IQR, 30.0–45.0) vs. Batch 5: 35 (IQR, 30.0–45.0) minutes, P=0.015]. Cryobiopsy service was introduced into our service starting Batch 2 onwards while thin bronchoscope was introduced in Batch 5 (Table 3, Figure 2).
Table 3
Variables | Batch | ||||
---|---|---|---|---|---|
1 | 2 | 3 | 4 | 5 | |
Changes in target lesion characteristic | |||||
Target size (cm) | 3.4 (2.69–4.52) | 2.89 (2.30–3.70) | 2.62 (2.08–3.37) | 2.90 (2.10–4.21) | 2.13 (1.78–2.80) |
Target to pleural distance (cm) | 3.17 (2.30–3.61) | 2.33 (1.86–3.41) | 2.31 (1.70–3.09) | 2.40 (1.50–3.67) | 2.08 (1.27–2.75) |
Changes in overall diagnostic yield (n=551) | |||||
Diagnostic yield (%) | 61.8 | 81.8 | 81.8 | 81.8 | 86.5 |
Changes in rEBUS performance for malignancy (n=502) | |||||
Sensitivity for malignancy | 69.49 (56.13–80.81) |
89.06 (78.75–95.49) |
92.31 (82.95–97.46) |
87.93 (76.70–95.01) |
86.30 (76.25–93.23) |
Diagnostic accuracy for malignancy | 81.63 (72.53–88.74) |
93.14 (86.37–97.20) |
94.85 (88.38–98.31) |
92.86 (85.84–97.08) |
90.65 (83.48–95.43) |
Changes in rEBUS performance for tuberculosis (n=502) | |||||
Sensitivity for tuberculosis | 46.67 (28.34–65.67) |
62.96 (42.37–80.60) |
71.43 (47.82–88.72) |
91.67 (73.00–98.97) |
98.45 (77.16–99.88) |
Diagnostic accuracy for tuberculosis | 83.67 (74.84–90.37) |
90.20 (82.71–95.20) |
93.81 (87.02–97.70) |
97.96 (92.82–99.75) |
99.07 (94.9–99.98) |
Method of tuberculosis diagnosis | |||||
Histology | 19 (63.3) | 16 (59.3) | 14 (66.7) | 14 (58.3) | 17 (77.3) |
Microbiology | 3 (10.0) | 1 (3.7) | 2 (9.5) | 8 (33.3) | 5 (22.7) |
Empirical | 8 (26.7) | 10 (37.0) | 5 (23.8) | 2 (8.3) | 0 (0.0) |
Data are presented as median (IQR), % (95% CI), or n (%). rEBUS, radial endobronchial ultrasound; IQR, interquartile range; CI, confidence interval.
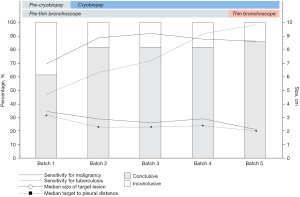
Changes in target lesion characteristic
Over the years, we have been able to access smaller lesions, with significantly decreasing median target size of 3.4 (IQR, 2.69–4.52) cm in first batch, to 2.13 (IQR, 1.78–2.80) cm in fifth batch, P<0.001. Accessed target PPL have also been more peripherally situated over the years with median target-to-pleural distance of 3.17 (IQR, 2.30–3.61) cm in Batch 1, to 2.08 (IQR, 1.27–2.75) cm in Batch 5, P<0.001.
Changes in overall diagnostic yield
Introduction of cryobiopsy into rEBUS services increased the diagnostic yield significantly (Batch 1 vs. Batch 2 onwards, 61.8% vs. 83.0%, P<0.001). However, the diagnostic yield then plateaued at 81.8% across the later three batches (Batch 2, 3, 4) with cryobiopsy services having become routine. Introduction of thin bronchoscope in Batch 5 service was associated with a non-significant increase of diagnostic yield from 81.8% to 86.5% (P=0.257).
Changes in rEBUS performance for malignant and tuberculous PPL
Across the five batches, neither sensitivity (r=0.57, P=0.313) nor diagnostic accuracy (r=0.53, P=0.353) of rEBUS for malignant PPL shown a positive correlation.
However, rEBUS demonstrated a positive correlation of diagnostic accuracy (r=0.97, P<0.01 and sensitivity (r=0.99, P<0.01) over the years. The method of tuberculous PPL diagnosis in the cohort also evolved over the years, with more cases diagnosed based on histological and microbiological criteria in Batch 5, rather than through empirical treatment response in Batch 1 (P<0.05).
Discussion
The diagnostic yield of rEBUS for PPL biopsy in our study was consistent with previously reported values, with an overall yield of 78.8% (7,8). In line with previous studies, the presence of a direct (type A) bronchus sign and a concentric rEBUS orientation were strong independent predictors of a successful rEBUS procedure (7,17). Fluoroscopy is a modality frequently employed to assist in rEBUS procedures. Our study revealed that there was no significant association between the use of fluoroscopy and the overall diagnostic yield. This finding aligns with a recent meta-analysis which also indicated no association between the usage of fluoroscopy and the overall performance of rEBUS procedures (18). Additionally, a recent study by Zheng et al. demonstrated the non-inferiority diagnostic yield of rEBUS (coupled with VBN and guide sheath) without fluoroscopy in target PPL with a direct bronchus sign (19).
Notably, our study reinforces the safety of rEBUS-guided biopsy, with a reported pneumothorax rate of only 1.1%, consistent with the literature. In our region, CT-guided transthoracic biopsy is the most common method for PPL biopsy. However, despite its higher diagnostic yield, it also carries a higher risk of complications, particularly pneumothorax, which can be as high as 25.5%, with 5.6% requiring percutaneous drainage (20). Recent data also suggests a potentially higher risk of ipsilateral pleural contamination, leading to an increased risk of subsequent ipsilateral pleural recurrence in malignant diseases (21). Therefore, proper patient selection for mode of biopsy is crucial to ensure patient safety, particularly for those who would benefit from the minimal risk of rEBUS-guided biopsy.
Our study also demonstrates that rEBUS without advanced automation technology is still feasible and relevant with equivalent diagnostic yield even in this era where guided bronchoscopy for PPL is frequently performed with the aid of VBN, catheter or robotic-based ENB systems, etc. Although VBN is commonly used in pre-procedural planning to create a virtual pathway to the target, the automation algorithm of VBN pathway creation can fail due to poor quality of pre-procedural CT scans, such as due to mucous impaction, as well as the innate inefficiency of automated algorithms in small airway identification in the extreme peripheral regions of the lung—these issues can frequently result in an incomplete or inaccurate navigational pathway creation (22,23). In contrast, manual airway mapping through bronchial branch tracing remains an effective navigation method for rEBUS navigation (14,24,25). When comparing the creation of a complete navigational road map using solely a VBN approach vs. a manual tracing method, Miyake et al. found that the manual tracing method outperformed the use of two commercially available VBN platforms in creating an accurate and complete navigational route to the target. This was observed as the manual method effectively reached the target in a higher proportion of cases (94%), while only around half of the cases accurately reached the target when using the VBN platforms (49% and 62%) (26). This technique has also been shown to improve bronchoscopic brushing sensitivity for malignant nodules in a large cohort of patients (27). Our previous experience also demonstrated the feasibility of this technique employing a larger caliber bronchoscope, with a navigation success rate of 98.9% and a histological diagnostic yield of 88.8% (14). Therefore, although automation technology may simplify the procedure, in regions where such technology is not available, a proper understanding of airway anatomy and manual navigation remains feasible and relevant to drive the rEBUS procedure with almost equivalent diagnostic yield.
According to the World Health Organization (WHO), tuberculosis affected 10.6 million people globally, with 45% of cases originating from the South-East Asia region (12). Given the high prevalence of tuberculosis in our region, rEBUS of PPL poses a unique challenge, as almost 2 in 10 of our cohort turned out to be tuberculous nodule, consistent with other reported series in the region (4,12). We have demonstrated that rEBUS can expedite tuberculosis diagnosis by providing histological and microbiological proof of disease, as rEBUS was performed only when initial sputum smears were negative for Mycobacterium. Over the years, the sensitivity of rEBUS for tuberculous PPL had increased in our study—this is likely attributable to the increasing use of rapid nucleic acid amplification tests in lavage specimens, such as Xpert® MTB/RIF and Mycobacterium culture which increase the diagnostic sensitivity. Recently, another study has also demonstrated higher detection rates for tuberculous nodule when bronchoalveolar lavage was performed in a more targeted manner using thin bronchoscope guided by VBN (28). This is important because it provides clinicians with the confidence to proceed with appropriate management, rather than empirical tuberculosis treatment which may lead to a delayed diagnosis of other equally serious causes, such as malignancy.
Over the years, introduction of two key changes in rEBUS practice have led to an improvement of overall performance. The introduction of cryobiopsy service significantly increased the overall diagnostic yield from 61.8% to 81.8%. This improvement could be multifactorial, including the learning curve effect, but is more likely due to an improvement in the biopsy method of eccentric and adjacently orientated lesions by cryobiopsy. In lesions with eccentric and adjacent orientations, cryobiopsy with its capability for circumferential biopsy, provides significantly better diagnostic yield than conventional forceps biopsy (29,30). More data on cryobiopsy in PPL has since emerged with an excellent diagnostic yield ranging 89.2–91.0% with acceptable complication rates (31,32). Secondly, the introduction of thin bronchoscope service has allowed us to access smaller and more peripherally lesions while maintaining overall diagnostic yield. Historically, thin bronchoscopes with small working channels had hindered rEBUS insertion, which led to unsatisfactory diagnostic yield (33). Improvement of thin bronchoscope design with larger working channel can now allow insertion of rEBUS for verification purpose which translates to better diagnostic yield (33). Interestingly, the combination of these two technologies (thin bronchoscope and cryobiopsy) has also recently been demonstrated in the literature to have a high diagnostic yield (74%) for small target lesions (34).
Our study is not without limitations. First, the retrospective and single institution design is associated with significant biases. However, we believe that this large cohort of real-world practice experience from the Southeast Asia region, which represents a unique cohort of patients, will be beneficial to clinicians when planning their approach to PPL diagnosis. Secondly, the high diagnostic yield reported in our cohort may be due to selection bias towards lesions with only visible bronchus sign on pre-procedural CT scans, as those deemed not feasible for rEBUS by the managing team were excluded from the procedure. Access into targets without bronchus sign remains a challenge, and although advanced transbronchial access tools are available in the market, they are not readily accessible worldwide (35,36). Moreover, the small proportion of ground-glass opacity (GGO) nodules in our cohort may have biased to the high overall diagnostic yield. Although GGO nodules can be localized by rEBUS through the identification of blizzard sign, it requires training and experience (37). Advancement in real-time imaging systems such as C-arm-based digital tomosynthesis and cone-beam CT may complement rEBUS in localizing GGO in the future (38,39). Thirdly, our study had a 6-month follow-up period for subjects with inflammatory biopsy result to establish a definitive diagnosis. This duration may be insufficient, as the literature typically considers a 12-month period of radiological stability as the standard (16). Lastly, we observed a longer total procedural duration in our study compared to what has been reported in the literature (24). Nevertheless, as our technique matured, we demonstrated a significant reduction in the overall procedural time. Furthermore, we did not explicitly present the duration required for bronchial branch tracing planning; however, it’s worth noting that manual mapping typically takes only around 2 to 5 min (24,26).
Conclusions
In conclusion, rEBUS without automation technology remains relevant and useful in this era. Its performance will be enhanced by advancements in guided bronchoscopy technology, including better navigation, steering tools, verification, and biopsy methods. In regions where tuberculosis is endemic, rEBUS provides a rapid and safe histological and microbiological proof of disease, reducing the risk of empirical treatment and improving patient care.
Acknowledgments
We would like to acknowledge the Director General of Health of the Ministry of Health Malaysia for granting permission to publish this paper.
Funding: None.
Footnote
Reporting Checklist: The authors have completed the STROBE reporting checklist. Available at https://jtd.amegroups.com/article/view/10.21037/jtd-23-979/rc
Data Sharing Statement: Available at https://jtd.amegroups.com/article/view/10.21037/jtd-23-979/dss
Peer Review File: Available at https://jtd.amegroups.com/article/view/10.21037/jtd-23-979/prf
Conflicts of Interest: All authors have completed the ICMJE uniform disclosure form (available at https://jtd.amegroups.com/article/view/10.21037/jtd-23-979/coif). The authors have no conflicts of interest to declare.
Ethical Statement: The authors are accountable for all aspects of the work in ensuring that questions related to the accuracy or integrity of any part of the work are appropriately investigated and resolved. The study was conducted in accordance with the Declaration of Helsinki (as revised in 2013). The study was approved by the medical research and ethics committee of Ministry of Health Malaysia [NMRR-ID-23-00520-LMM (IIR)] and individual consent for this retrospective analysis was waived.
Open Access Statement: This is an Open Access article distributed in accordance with the Creative Commons Attribution-NonCommercial-NoDerivs 4.0 International License (CC BY-NC-ND 4.0), which permits the non-commercial replication and distribution of the article with the strict proviso that no changes or edits are made and the original work is properly cited (including links to both the formal publication through the relevant DOI and the license). See: https://creativecommons.org/licenses/by-nc-nd/4.0/.
References
- Bai C, Choi CM, Chu CM, et al. Evaluation of Pulmonary Nodules: Clinical Practice Consensus Guidelines for Asia. Chest 2016;150:877-93. [Crossref] [PubMed]
- MacMahon H, Naidich DP, Goo JM, et al. Guidelines for Management of Incidental Pulmonary Nodules Detected on CT Images: From the Fleischner Society 2017. Radiology 2017;284:228-43. [Crossref] [PubMed]
- National Lung Screening Trial Research Team. Reduced lung-cancer mortality with low-dose computed tomographic screening. N Engl J Med 2011;365:395-409. [Crossref] [PubMed]
- Chan A, Devanand A, Low SY, et al. Radial endobronchial ultrasound in diagnosing peripheral lung lesions in a high tuberculosis setting. BMC Pulm Med 2015;15:90. [Crossref] [PubMed]
- Tan H, Bates JHT, Matthew Kinsey C. Discriminating TB lung nodules from early lung cancers using deep learning. BMC Med Inform Decis Mak 2022;22:161. [Crossref] [PubMed]
- Ho ATN, Gorthi R, Lee R, et al. Solitary Lung Nodule: CT-Guided Transthoracic Biopsy vs Transbronchial Biopsy With Endobronchial Ultrasound and Flexible Bronchoscope, a Meta-Analysis of Randomized Controlled Trials. Lung 2023;201:85-93. [Crossref] [PubMed]
- Ali MS, Trick W, Mba BI, et al. Radial endobronchial ultrasound for the diagnosis of peripheral pulmonary lesions: A systematic review and meta-analysis. Respirology 2017;22:443-53. [Crossref] [PubMed]
- Lachkar S, Perrot L, Gervereau D, et al. Radial-EBUS and virtual bronchoscopy planner for peripheral lung cancer diagnosis: How it became the first-line endoscopic procedure. Thorac Cancer 2022;13:2854-60. [Crossref] [PubMed]
- Khandhar SJ, Bowling MR, Flandes J, et al. Electromagnetic navigation bronchoscopy to access lung lesions in 1,000 subjects: first results of the prospective, multicenter NAVIGATE study. BMC Pulm Med 2017;17:59. [Crossref] [PubMed]
- Chen AC, Pastis NJ Jr, Mahajan AK, et al. Robotic Bronchoscopy for Peripheral Pulmonary Lesions: A Multicenter Pilot and Feasibility Study (BENEFIT). Chest 2021;159:845-52. [Crossref] [PubMed]
- World Medical Association Declaration of Helsinki. ethical principles for medical research involving human subjects. JAMA 2013;310:2191-4. [Crossref] [PubMed]
- World Health Organization. Global tuberculosis report 2022. 2022. [Cited 23 April 2023]. Available online: https://www.who.int/publications/i/item/9789240061729
- Minezawa T, Okamura T, Yatsuya H, et al. Bronchus sign on thin-section computed tomography is a powerful predictive factor for successful transbronchial biopsy using endobronchial ultrasound with a guide sheath for small peripheral lung lesions: a retrospective observational study. BMC Med Imaging 2015;15:21. [Crossref] [PubMed]
- Kho SS, Nyanti LE, Chai CS, et al. Feasibility of manual bronchial branch reading technique in navigating conventional rEBUS bronchoscopy in the evaluation of peripheral pulmonary lesion. Clin Respir J 2021;15:595-603. [Crossref] [PubMed]
- Matsumoto Y, Izumo T, Sasada S, et al. Diagnostic utility of endobronchial ultrasound with a guide sheath under the computed tomography workstation (ziostation) for small peripheral pulmonary lesions. Clin Respir J 2017;11:185-92. [Crossref] [PubMed]
- Ost DE, Ernst A, Lei X, et al. Diagnostic Yield and Complications of Bronchoscopy for Peripheral Lung Lesions. Results of the AQuIRE Registry. Am J Respir Crit Care Med 2016;193:68-77. [Crossref] [PubMed]
- Ito T, Matsumoto Y, Okachi S, et al. A Diagnostic Predictive Model of Bronchoscopy with Radial Endobronchial Ultrasound for Peripheral Pulmonary Lesions. Respiration 2022;101:1148-56. [Crossref] [PubMed]
- Sainz Zuñiga PV, Vakil E, Molina S, et al. Sensitivity of Radial Endobronchial Ultrasound-Guided Bronchoscopy for Lung Cancer in Patients With Peripheral Pulmonary Lesions: An Updated Meta-analysis. Chest 2020;157:994-1011. [Crossref] [PubMed]
- Zheng X, Zhong C, Xie F, et al. Virtual bronchoscopic navigation and endobronchial ultrasound with a guide sheath without fluoroscopy for diagnosing peripheral pulmonary lesions with a bronchus leading to or adjacent to the lesion: A randomized non-inferiority trial. Respirology 2023;28:389-98. [Crossref] [PubMed]
- Heerink WJ, de Bock GH, de Jonge GJ, et al. Complication rates of CT-guided transthoracic lung biopsy: meta-analysis. Eur Radiol 2017;27:138-48. [Crossref] [PubMed]
- Hong H, Hahn S, Matsuguma H, et al. Pleural recurrence after transthoracic needle lung biopsy in stage I lung cancer: a systematic review and individual patient-level meta-analysis. Thorax 2021;76:582-90. [Crossref] [PubMed]
- Fielding D, Oki M. Technologies for targeting the peripheral pulmonary nodule including robotics. Respirology 2020;25:914-23. [Crossref] [PubMed]
- Akulian JA, Molena D, Wahidi MM, et al. A Direct Comparative Study of Bronchoscopic Navigation Planning Platforms for Peripheral Lung Navigation: The ATLAS Study. J Bronchology Interv Pulmonol 2022;29:171-8. [Crossref] [PubMed]
- Zhong CH, Su ZQ, Luo WZ, et al. Hierarchical clock-scale hand-drawn mapping as a simple method for bronchoscopic navigation in peripheral pulmonary nodule. Respir Res 2022;23:245. [Crossref] [PubMed]
- Inoue T, Kawamura T, Kunimasa K, et al. Manual route modification using an oblique method following automatic virtual bronchoscopic navigation. Medicine (Baltimore) 2022;101:e29076. [Crossref] [PubMed]
- Miyake K, Morimura O, Inoue T, et al. The Direct Oblique Method: A New Gold Standard for Bronchoscopic Navigation That is Superior to Automatic Methods. J Bronchology Interv Pulmonol 2018;25:305-14. [Crossref] [PubMed]
- Zhang L, Tong R, Wang J, et al. Improvements to bronchoscopic brushing with a manual mapping method: A three-year experience of 1143 cases. Thorac Cancer 2016;7:72-9. [Crossref] [PubMed]
- Eom JS, Park S, Jang H, et al. Bronchial Washing Using a Thin Versus a Thick Bronchoscope to Diagnose Pulmonary Tuberculosis: A Randomized Trial. Clin Infect Dis 2023;76:238-44. [Crossref] [PubMed]
- Kho SS, Chan SK, Yong MC, et al. Performance of transbronchial cryobiopsy in eccentrically and adjacently orientated radial endobronchial ultrasound lesions. ERJ Open Res 2019;5:00135-2019. [Crossref] [PubMed]
- Matsumoto Y, Nakai T, Tanaka M, et al. Diagnostic Outcomes and Safety of Cryobiopsy Added to Conventional Sampling Methods: An Observational Study. Chest 2021;160:1890-901. [Crossref] [PubMed]
- Herth FJ, Mayer M, Thiboutot J, et al. Safety and Performance of Transbronchial Cryobiopsy for Parenchymal Lung Lesions. Chest 2021;160:1512-9. [Crossref] [PubMed]
- Furuse H, Matsumoto Y, Nakai T, et al. Diagnostic efficacy of cryobiopsy for peripheral pulmonary lesions: A propensity score analysis. Lung Cancer 2023;178:220-8. [Crossref] [PubMed]
- Kim SH, Kim J, Pak K, et al. Ultrathin Bronchoscopy for the Diagnosis of Peripheral Pulmonary Lesions: A Meta-Analysis. Respiration 2023;102:34-45. [Crossref] [PubMed]
- Oki M, Saka H, Kogure Y, et al. Ultrathin bronchoscopic cryobiopsy of peripheral pulmonary lesions. Respirology 2023;28:143-51. [Crossref] [PubMed]
- Bowling MR, Brown C, Anciano CJ. Feasibility and Safety of the Transbronchial Access Tool for Peripheral Pulmonary Nodule and Mass. Ann Thorac Surg 2017;104:443-9. [Crossref] [PubMed]
- Herth FJ, Eberhardt R, Sterman D, et al. Bronchoscopic transparenchymal nodule access (BTPNA): first in human trial of a novel procedure for sampling solitary pulmonary nodules. Thorax 2015;70:326-32. [Crossref] [PubMed]
- Izumo T, Sasada S, Chavez C, et al. Radial endobronchial ultrasound images for ground-glass opacity pulmonary lesions. Eur Respir J 2015;45:1661-8. [Crossref] [PubMed]
- Podder S, Chaudry S, Singh H, et al. Efficacy and Safety of Cone-Beam CT Augmented Electromagnetic Navigation Guided Bronchoscopic Biopsies of Indeterminate Pulmonary Nodules. Tomography 2022;8:2049-58. [Crossref] [PubMed]
- Aboudara M, Roller L, Rickman O, et al. Improved diagnostic yield for lung nodules with digital tomosynthesis-corrected navigational bronchoscopy: Initial experience with a novel adjunct. Respirology 2020;25:206-13. [Crossref] [PubMed]