Genetic characteristics of lung adenocarcinoma with ground-glass opacity component
Highlight box
Key findings
• When compared to ground-glass opacity featured lung adenocarcinoma (GGO-LUAD), the mutation frequency of the tumor suppressor genes, TP53 and CDKN2A were found to be higher in solid-LUAD of similar histologically invasive size. Furthermore, gene mutations were more likely to be concentrated in the cell cycle and TP53 signaling pathway in solid-LUAD than in GGO-LUAD.
What is known and what is new?
• GGO-LUAD and solid-LUAD are completely distinct types of tumors, and they significantly differ in their prognosis.
• We used next-generation sequencing technology to analyze and compare gene mutations in a large gene panel across multiple signaling pathways between GGO-LUAD and solid-LUAD.
What is the implication, and what should change now?
• The wild-type TP53 and CDKN2A genes could potentially serve as molecular markers for the indolent behavior of GGO-LUAD. However, genetic testing with larger samples is necessary to further explore the involved key genes and signaling pathways.
Introduction
Increasing evidence suggests that ground-glass opacity featured lung adenocarcinoma (GGO-LUAD) and pure solid-LUAD have significantly different tumor biological behaviors; the former is usually indolent and has a good prognosis. Some scholars even suggest that the two should be distinguished in future T-staging systems by introducing the layering parameter of whether the tumor contains GGO components (1). Next-generation sequencing (NGS) technology allows high-throughput and high-sensitivity multigene sequencing. NGS plays an increasingly important role in tumor genetic studies and is expected to be used to guide postoperative targeted therapy and monitor the recurrence of early lung cancer. Just as the TRACERx study was performed to decipher lung cancer evolution and determine the relationship between intratumor heterogeneity and clinical outcome (2), it is feasible to analyze the difference in oncogenes in different biological behaviors of the same histological type by using NGS technology in a large panel and multiple signaling pathways. Therefore, we conducted the following study to analyze and compare the gene mutations between GGO-LUAD and pure solid-LUAD using NGS technology. We present this article in accordance with the MDAR reporting checklist (available at https://jtd.amegroups.com/article/view/10.21037/jtd-23-745/rc).
Methods
Inclusion and exclusion criteria
The data of clinically early-stage LUAD patients operated in the Thoracic Surgery Department of Beijing Tsinghua Changgung Hospital with a histologically invasive subtype ≤3 cm who underwent NGS testing from May 2019 to December 2022 were collected. These patients underwent segmentectomy or lobectomy (depending on the location and size of the tumor) plus mediastinal lymph node dissection to obtain whole tumor specimens. Genetic tests were all performed on the tissue samples that were obtained from surgically removed tumor specimens. Non-lepidic growth components were defined as histologically invasive subtypes, including acinar, papillary, and micropapillary. For multiple ground-glass nodules, only the nodules with the largest invasive component were analyzed if both excision and genetic testing were performed. Cases of carcinoma in situ or minimally invasive adenocarcinoma were excluded because of their apparent oncological indolent nature and favorable prognosis in previous studies. Cases of mucinous LUAD and patients with negative genetic test results were excluded. Patients who met the requirements were divided into two groups according to the computed tomography (CT) imaging: GGO-LUAD, 0≤ consolidation-to-tumor ratio (CTR) <1 and pure solid-LUAD, CTR =1.
Sequencing protocol
The LungTrak panel protocol developed by Geneseeq Company (Nanjing, China) was used for genetic testing, which can identify 139 lung cancer-related genes simultaneously. We extracted genomic DNA from formalin-fixed, paraffin-embedded (FFPE) tissue samples and white blood cells using the QIAamp DNA FFPE Tissue Kit (Qiagen, Dusseldorf, Germany). All DNA concentrations and purities were qualified by a NanoDrop 2000 (Thermo Fisher Scientific, Boston, USA). All DNA samples were also quantified by Qubit 3.0 using the double-stranded DNA (dsDNA) high sensitivity (HS) Assay Kit (Life Technologies, Grand Island, USA) according to the manufacturer’s protocol.
Sequencing libraries were constructed using the KAPA Hyper Prep kit (KAPA Biosystems, Wilmington, MA, USA) following the optimized manufacturer’s instructions. Briefly, DNA went through end repair, A-tailing, adapter ligation and size selection using Agencourt AMPure XP beads (Beckman Coulter, Brea, CA, USA). After constructing libraries, we conducted polymerase chain reaction (PCR) amplification and purification before targeted enrichment. The size distribution of the libraries was measured by an Agilent Technologies 2100 Bioanalyzer (Agilent Technologies, California, USA). The enriched libraries were sequenced on Illumina HiSeq 4000 (Illumina, San Diego, CA, USA) NGS platforms to cover mean depths of at least 1,000× for FFPE samples. For bioinformatics analyses, trimmomatic48 was used for FASTQ file quality control (QC) (3). Sequencing data were aligned to the reference hg19 (Human Genome version 19) with the Burrows-Wheeler Aligner (BWA-mem, v0.7.12) (4). Single nucleotide variants (SNVs) and indels were detected using SCALPEL (http://scalpel.sourceforge.net) and the Genome Analysis Toolkit (GATK). Tumor purity was estimated by ABSOLUTE (5). Purity-adjusted gene-level and segment-level copy numbers were calculated by copy number variation (CNV) Kit (6) ADTEx (http://adtex.sourceforge.net).
Study design
A case-control study was performed to compare the differences in general information, pathological results, and genetic testing results between the two groups. Genes belonging to the same signaling pathway were used as a variable factor for mutation enrichment signaling pathway analysis. The maximum diameter of histological invasive subtypes was compared between the two groups to determine whether it was matched. According to previous experience and research results, the parameters that may be related to tumor metastasis or biological behavior malignancy were selected for comparative analysis. The study was conducted in accordance with the Declaration of Helsinki (as revised in 2013). This study was approved by the Institutional Review Board (IRB) of Beijing Tsinghua Changgung Hospital Ethics Committee (IRB No. 23324-6-01). The need for patient consent was waived due to the retrospective nature of the study.
Statistical analysis
SPSS 19.0 software was used to statistically process the data. The measurement data with a nonnormal distribution are expressed as the median (quartile), and the comparison between the two groups was conducted by a nonparametric test (Mann-Whitney test). χ2 tests were used to compare count data between the two groups. R 3.5.3 was used to conduct Fisher’s test analysis and plot the differences between different genes and signaling pathways. All P values tested were bilateral, with a difference of P<0.05 being statistically significant.
Results
Data from 53 patients were collected, and five cases of carcinoma in situ or minimally invasive adenocarcinoma were excluded. One case of mucinous LUAD was excluded. One patient with negative genetic test results was excluded. Finally, a total of 46 patients were included in the analysis, including 24 females and 22 males, with an average age of 63.4 years (range, 42 to 80 years). There were 31 cases of GGO-LUAD and 15 cases of solid-LUAD.
Comparison of the size of the tumor on CT and histologic subtypes between solid LUAD and GGO-LUAD
The size was represented by the maximum diameter, and the size of pathologic invasive subtypes was the total pathologic tumor size multiplied by the proportion of non-Lepidic subtypes. The total tumor size on CT of GGO-LUAD [18.5 mm (15.0, 27.0 mm)] vs. solid-LUAD [20.0 mm (15.0, 25.0 mm)] (Mann-Whitney test, Z=−0.07, P>0.05) was not significantly different. The pathologic tumor size of solid LUAD was 17.0 mm (12.0, 23.5 mm), which was almost the same as the pathologic invasive size. However, in the GGO-LUAD group, the difference between the size of the solid part on CT and the size of the pathologically invasive lesion was relatively obvious: [8 mm (3, 15 mm)] vs. [14.3 mm (8.1, 20.9 mm)]. The size of the pathologic invasive subtypes was as follows: GGO-LUAD [14.3 mm (8.1, 20.9 mm)] vs. solid-LUAD [17.0 mm (12.0, 23.5 mm)] (Mann-Whitney test, Z=−1.583, P>0.05), there was no significant difference between the two groups. The size of histologic invasive subtypes between the two groups was matched.
Comparison of general information between solid-LUAD and GGO-LUAD
The solid-LUAD group had all solitary nodules, except for one case that simultaneously merged with a ground glass nodule. In GGO-LUAD, 13 cases (13/31, 41.9%) had more than two multiple nodules, and there was a significant difference between the two. There was no significant difference in age, sex, or smoking status between the two groups (Table 1).
Table 1
Variables | Classification | Solid-LUAD | GGO-LUAD | P value |
---|---|---|---|---|
Age, years | ≤60 | 5 | 9 | >0.99 |
>60 | 10 | 22 | ||
Gender | Male | 8 | 14 | 0.755 |
Female | 7 | 17 | ||
Smoking status | Yes | 6 | 10 | 0.744 |
No | 9 | 21 | ||
Multiple nodules | Yes | 1 | 13 | 0.018 |
No | 14 | 18 |
Solid-LUAD, pure solid lung adenocarcinoma; GGO-LUAD, ground-glass opacity featuring lung adenocarcinoma.
Comparison of pathological data between solid-LUAD and GGO-LUAD
In the solid-LUAD group, almost all pathologic subtypes were non-Lepidic growth subtypes, with only one case containing approximately 5% of the Lepidic growth subtypes. Among them, solid predominant (7/15) and acinar predominant (7/15) were the most common subtypes. Moreover, the tumor was often a mixture of multiple subtypes. In the GGO-LUAD group, almost all of the tumors contained lepidic subtypes. Among the other pathologic invasive subtypes mixed with the tumor, the most common was acinar (21/31), a few were papillary (7/31), and only one case was found to contain the solid subtype (less than 5%).
There were three cases of postoperative lymph node staging upgrades, which only occurred in solid-LUAD, and no lymph node metastasis was found in GGO-LUAD. There was a significant difference in vascular invasion, tumor spread through air spaces (STAS) and high-risk histological subtypes (micropapillary or solid subtypes) between the two groups. STAS was not observed in GGO-LUAD (Table 2).
Table 2
Variables | Classification | Solid-LUAD | GGO-LUAD | P value |
---|---|---|---|---|
pN | N0 | 12 | 31 | 0.030 |
N+ | 3 | 0 | ||
Micropapillary or solid subtypes | Yes | 11 | 1 | <0.001 |
No | 4 | 30 | ||
Vascular invasion | Yes | 4 | 1 | 0.033 |
No | 11 | 30 | ||
STAS | Yes | 6 | 0 | 0.001 |
No | 9 | 31 |
Solid-LUAD, pure solid lung adenocarcinoma; GGO-LUAD, ground-glass opacity featuring lung adenocarcinoma; pN, pathological lymph node staging; STAS, tumor spread through air spaces.
Gene testing results of all cases
A total of 137 mutations were identified in 46 patients, averaging around three mutations per individual; The most common type of mutation was missense mutations, which made up 60.6% (83/137) of the total, followed by in-frame deletion mutations, accounting for 11.7% (16/137).
The genes with the highest frequency of mutations were EGFR, TP53 and KRAS. EGFR mutations were most common, found in 71.7% (33/46). These mutations were of five types, with the most common being EGFR_19del, EGFR_L858R, EGFR_719/768/861. TP53 mutations were found in 39.1% (18/46) of patients, and were of 19 different types. The most common were TP53_Q136E and TP53_198*. KRAS mutations were less common, found in 15.2% (7/46) of patients. These were of five different types, with KRAS_G12D, KRAS_G12A, and KRAS_G12E being the most prevalent. The mutations were primarily concentrated in the RAS-RAF (95.7%) and TP53 (39.1%) (Figure 1).
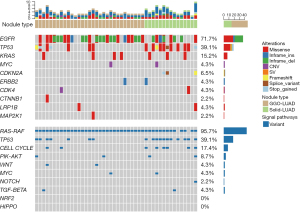
Comparison of gene testing results between solid-LUAD and GGO-LUAD
There was no notable difference in the frequency of EGFR and KRAS mutations between solid-LUAD and GGO-LUAD. However, the frequency of TP53 and CDKN2A mutations was significantly higher in solid-LUAD. Notably, CDKN2A gene mutations were not found in GGO-LUAD. Compared to GGO-LUAD, solid-LUAD displayed a higher concentration of mutations in the TP53 and cell cycle signaling pathways (Table 3).
Table 3
Category | Variables | Classification | Solid-LUAD | GGO-LUAD | P value |
---|---|---|---|---|---|
Genes | EGFR | Mutation | 8 | 25 | 0.082 |
Wild | 7 | 6 | |||
KRAS | Mutation | 3 | 4 | 0.667 | |
Wild | 12 | 27 | |||
TP53 | Mutation | 11 | 7 | 0.001 | |
Wild | 4 | 24 | |||
CDKN2A | Mutation | 3 | 0 | 0.030 | |
Wild | 12 | 31 | |||
Signaling pathways | RAS-RAF | Mutation | 13 | 31 | 0.101 |
Wild | 2 | 0 | |||
TP53 | Mutation | 11 | 7 | 0.001 | |
Wild | 4 | 24 | |||
Cell cycle | Mutation | 6 | 2 | 0.010 | |
Wild | 9 | 29 |
Solid-LUAD, pure solid lung adenocarcinoma; GGO-LUAD, ground-glass opacity featuring lung adenocarcinoma.
Discussion
In this group of cases, although the size of invasive histological subtypes (non-lepidic growth components) related to tumor invasion was similar between GGO-LUAD and solid-LUAD, there were many significant differences between the two groups in terms of histological high-risk factors, gene mutations, and mutation enrichment signaling pathways.
The vast majority of persistent ground-glass nodules are primary LUAD (7). GGO-LUAD progresses slowly, generally over the course of many years, and is indolent (8,9). Chang et al. observed that only approximately 12% of pure ground-glass nodules progressed during a 6-year follow-up (10). The probability of mixed ground-glass nodules developing is slightly higher, approximately 29–41%, but generally no lymph node or distant metastasis occurs (11,12). Some scholars even refer to this type of lung cancer as “nonfatal lung cancer”, which is completely inconsistent with solid LUAD. Solid-LUAD is often in the micropapillary or solid histological subtype. In this group, the risk factors for pathological recurrence of solid LUAD were significantly greater than those of GGO-LUAD, suggesting that the prognosis of solid LUAD is not as good as that of GGO-LUAD.
According to the World Health Organization new pathological classification of LUAD, the lepidic growth subtype is considered a noninvasive growth pattern, which basically corresponds to GGOs on CT scans (13). Therefore, the non-lepidic growth component can be defined as a histologically invasive subtype, which means that the tumor cells are at risk for metastasis. However, imaging GGO and histological lepidic growth do not always correspond. In cases with pure GGO on CT, 39–48% are still invasive adenocarcinoma, including invasive components such as acinar or papillary components. Fu et al. reported that among invasive LUADs presenting as pure GGOs, 45% are non-lepidic growth subtypes (14-16). Over the past few years, research has shown that the presence of GGO components on imaging results can serve as an independent predictor of good prognosis in GGO-LUAD, without even considering the invasive component (1,17-19). Some studies have shown that even if the GGO component is small, the prognosis of GGO-LUAD is still better than that of solid-LUAD (20). Although both GGO-LUAD and solid-LUAD are LUADs, they are completely different tumors. Similar to this group, the risk factors for pathological recurrence were more common in solid LUAD, with significant differences. Genetic differences are the fundamental cause of tumor heterogeneity.
Kobayashi et al. proposed that the escalation in tumor malignancy necessitates further activation of certain genes, predominantly EGFR, KRAS, and TP53 (21). This is consistent with the gene exhibiting the highest mutation frequency rate identified in this group. Sanchez Vega, F and other research teams from the The Cancer Genome Atlas (TCGA) program analyzed the mechanism and pattern of somatic mutation across ten classic signaling pathways in 502 cases of LUAD. They discovered that the mutation of the RAS-RAF signaling pathway was up to 74%, followed by TP53 at 61% and the cell cycle at 56% (22). The mutations in this group were chiefly concentrated in the RAS-RAF and TP53 signaling pathways.
EGFR and KRAS are key genes in the RAS-RAF signaling pathway in LUAD; in this study, these two genes hold the greatest weight. Kobayashi et al. conducted simple driver gene testing (EGFR/KRAS/ALK/HER2) on surgically removed pulmonary ground-glass nodules and found that EGFR mutations occurred in 64.5% of the cases. Approximately 80% of invasive LUADs (including microinvasive adenocarcinoma and invasive adenocarcinoma) exhibit EGFR mutations. In addition, EGFR mutations were present in 90% of progressive nodules analyzed using long-term CT follow-ups (23). The research by Lu et al. showed that the increase in histological malignancy is basically synchronized with the increase in EGFR mutation frequency. CT follow-up indicated that patients with ground-glass nodules with EGFR mutations had a higher likelihood growth (24). Lu et al. inferred that EGFR mutations are associated with GGO-LUAD invasiveness. However, Li et al. found that EGFR mutation was not a prognostic factor for overall survival (OS) and recurrence-free survival (RFS) in stage cIA LUAD containing GGO components (25). Similarly, Hattori et al. also found no significant difference in the 5-year RFS between the clinical stage I EGFR mutation group and the wild-type group (67.3% vs. 64.9%) for solid-LUAD (26). Our research showed that there was no significant difference in the frequency of EGFR mutation between GGO-LUAD and solid-LUAD.
Similar to the case with EGFR, no significant difference was observed in the mutation frequency of the KRAS between GGO-LUAD and solid-LUAD within this group. The mutation rate of the KRAS gene in non-small cell lung cancer is 8% to 10% in Chinese patients. KRAS has been consistently viewed as a “non-producible drug” target, and there are no effective targeted drugs available (27). Both EGFR and KRAS may be involved in the development of both GGO-LUAD and solid-LUAD.
Compared to GGO-LUAD, oncogene mutations in solid-LUAD were more likely to be concentrated in the cell cycle and TP53 signaling pathways. A noticeable difference was observed in the mutation frequency of the tumor suppressor genes, TP53 and CDKN2A, between the two groups.
The tumor suppressor gene TP53 is a critical gene in the TP53 signaling pathway, and the gene with the highest correlation with human tumors that has been discovered thus far (27). The majority of mutations in the TP53 gene are missense mutations, accounting for about 75% (28). The mutant TP53 gene plays the role of a proto-oncogene and can promote tumor development and growth (29). The frequency of TP53 mutations in LUAD is approximately 34% (30). The overall survival of untreated p53-positive non-small cell lung cancer patients is significantly shorter than that of p53-negative patients (31). The TP53 signaling pathway often becomes inactivated due to mutations in TP53 gene itself or alterations in its regulatory factors, including CDKN2A, also known as p14ARF and MDM2.8 (32).
The CDKN2A gene is a representative gene in the cell cycle signaling pathway, also known as a tumor suppressor gene, encoding a cycle suppressor protein that plays a crucial role in cell cycle regulation. Inactivation mutations of CDKN2A frequently found in lung cancer and have been reported in various cancers, closely related to the occurrence of cancer (33). Compared with those of CDKN2A wild-type patients, CDKN2A mutant patients have poorer PFS and OS and are more susceptible to cancer recurrence (34).
In summary, with similar sizes of histological invasive components ≤3 cm, GGO-LUAD of clinical early stage had a lower mutation frequency than solid-LUAD in the suppressor genes TP53 and CDKN2A, and the mutant gene was less enriched in the cell cycle and TP53 signaling pathway, which may be the related genetic mechanism underlying fewer recurrence risk factors, relatively inactive cell growth, and less migration/invasion for GGO-LUAD.
The limitation of this study is that the sample size is small. At the same time, due to the heterogeneity of tumors, genetic testing may not be able to comprehensively assess tumor gene mutations. In addition, some patients with similar conditions to those included in this study did not undergo tumor NGS because of the high cost and its uncertain prognostic predictive value, especially patients presenting with small pure ground glass nodules or whose histological subtype predominantly consisted of lepidic growth pattern, and their genetic mutation status was unclear.
Although there is evidence to suggest that early LUAD may transition from atypical adenomatous hyperplasia (AAH) to adenocarcinoma in situ (AIS), minimally invasive adenocarcinoma (MIA), and ultimately to invasive LUAD, which progresses from GGO to solid tumor on imaging (35). However, this progression is not always observed in real cases. Whether and when GGO-LUAD will eventually progress to solid LUAD are major clinical challenges that cannot be well predicted. In the future, genetic testing with larger samples is needed to explore the key genes and signaling pathways involved, thereby explaining the genetic characteristics and molecular mechanisms of tumor heterogeneity. For those patients with GGO-LUAD after resection, if the patient has the above genetic mutation, we can focus our attention and carry out wild-type and mutant prognostic analysis. In the future, we can design a panel protocol based on these genes and use liquid biopsy technology to dynamically monitor GGO-LUAD patients who do not want to undergo repeated radiography or who cannot tell whether the tumor is no longer indolent, even after radiography follow-up, to predict whether the tumor has turned to a more aggressive degree or already had invasiveness and migration capability.
Conclusions
The wild-type TP53 and CDKN2A genes could potentially be molecular markers for GGO-featured indolent LUAD. Given the limitations of the study, this conclusion is speculative and should be interpreted with caution.
Acknowledgments
We want to thank AME Editing Service for their help in English language editing.
Funding: None.
Footnote
Reporting Checklist: The authors have completed the MDAR reporting checklist. Available at https://jtd.amegroups.com/article/view/10.21037/jtd-23-745/rc
Data Sharing Statement: Available at https://jtd.amegroups.com/article/view/10.21037/jtd-23-745/dss
Peer Review File: Available at https://jtd.amegroups.com/article/view/10.21037/jtd-23-745/prf
Conflicts of Interest: All authors have completed the ICMJE uniform disclosure form (available at https://jtd.amegroups.com/article/view/10.21037/jtd-23-745/coif). N.B. is the project manager in the medical department of Nanjing Geneseeq Technology Inc. and participated in the project data analysis. The other authors have no conflicts of interest to declare.
Ethical Statement: The authors are accountable for all aspects of the work in ensuring that questions related to the accuracy or integrity of any part of the work are appropriately investigated and resolved. The study was conducted in accordance with the Declaration of Helsinki (as revised in 2013). This study was approved by the Institutional Review Board (IRB) of Beijing Tsinghua Changgung Hospital Ethics Committee (IRB No. 23324-6-01). The need for patient consent was waived due to the retrospective nature of the study.
Open Access Statement: This is an Open Access article distributed in accordance with the Creative Commons Attribution-NonCommercial-NoDerivs 4.0 International License (CC BY-NC-ND 4.0), which permits the non-commercial replication and distribution of the article with the strict proviso that no changes or edits are made and the original work is properly cited (including links to both the formal publication through the relevant DOI and the license). See: https://creativecommons.org/licenses/by-nc-nd/4.0/.
References
- Joubert P, Travis WD. Prognostic Impact of Ground-Glass Opacity/Lepidic Component in Pulmonary Adenocarcinoma: A Hazy Staging Dilemma. J Thorac Oncol 2022;17:19-21. [Crossref] [PubMed]
- Frankell AM, Dietzen M, Al Bakir M, et al. The evolution of lung cancer and impact of subclonal selection in TRACERx. Nature 2023;616:525-33. [Crossref] [PubMed]
- Bolger AM, Lohse M, Usadel B. Trimmomatic: a flexible trimmer for Illumina sequence data. Bioinformatics 2014;30:2114-20. [Crossref] [PubMed]
- Li H, Durbin R. Fast and accurate short read alignment with Burrows-Wheeler transform. Bioinformatics 2009;25:1754-60. [Crossref] [PubMed]
- Carter SL, Cibulskis K, Helman E, et al. Absolute quantification of somatic DNA alterations in human cancer. Nat Biotechnol 2012;30:413-21. [Crossref] [PubMed]
- Talevich E, Shain AH, Botton T, et al. CNVkit: Genome-Wide Copy Number Detection and Visualization from Targeted DNA Sequencing. PLoS Comput Biol 2016;12:e1004873. [Crossref] [PubMed]
- Oh JY, Kwon SY, Yoon HI, et al. Clinical significance of a solitary ground-glass opacity (GGO) lesion of the lung detected by chest CT. Lung Cancer 2007;55:67-73. [Crossref] [PubMed]
- Lee SW, Leem CS, Kim TJ, et al. The long-term course of ground-glass opacities detected on thin-section computed tomography. Respir Med 2013;107:904-10. [Crossref] [PubMed]
- Hiramatsu M, Inagaki T, Inagaki T, et al. Pulmonary ground-glass opacity (GGO) lesions-large size and a history of lung cancer are risk factors for growth. J Thorac Oncol 2008;3:1245-50. [Crossref] [PubMed]
- Chang B, Hwang JH, Choi YH, et al. Natural history of pure ground-glass opacity lung nodules detected by low-dose CT scan. Chest 2013;143:172-8. [Crossref] [PubMed]
- Matsuguma H, Mori K, Nakahara R, et al. Characteristics of subsolid pulmonary nodules showing growth during follow-up with CT scanning. Chest 2013;143:436-43. [Crossref] [PubMed]
- Kobayashi Y, Fukui T, Ito S, et al. How long should small lung lesions of ground-glass opacity be followed? J Thorac Oncol 2013;8:309-14. [Crossref] [PubMed]
- Travis WD, Asamura H, Bankier AA, et al. The IASLC Lung Cancer Staging Project: Proposals for Coding T Categories for Subsolid Nodules and Assessment of Tumor Size in Part-Solid Tumors in the Forthcoming Eighth Edition of the TNM Classification of Lung Cancer. J Thorac Oncol 2016;11:1204-23.
- Fu F, Ma X, Zhang Y, et al. Personalized treatment strategy for ground-glass opacity-featured lung cancer. Chinese Journal of Thoracic and Cardiovascular Surgery 2022;29:1-10. [Crossref]
- Zhang Y, Fu F, Chen H. Management of Ground-Glass Opacities in the Lung Cancer Spectrum. Ann Thorac Surg 2020;110:1796-804. [Crossref] [PubMed]
- Fu F, Zhang Y, Wen Z, et al. Distinct Prognostic Factors in Patients with Stage I Non-Small Cell Lung Cancer with Radiologic Part-Solid or Solid Lesions. J Thorac Oncol 2019;14:2133-42. [Crossref] [PubMed]
- Watanabe Y, Hattori A, Nojiri S, et al. Clinical impact of a small component of ground-glass opacity in solid-dominant clinical stage IA non-small cell lung cancer. J Thorac Cardiovasc Surg 2022;163:791-801.e4. [Crossref] [PubMed]
- Fan F, Zhang Y, Fu F, et al. Subsolid Lesions Exceeding 3 Centimeters: The Ground-Glass Opacity Component Still Matters. Ann Thorac Surg 2022;113:984-92. [Crossref] [PubMed]
- Sato D, Matsubara H, Matsuoka H, et al. Lepidic growth component as a favorable prognostic factor in non-small cell lung cancer of ≤3 cm. Thorac Cancer 2022;13:3274-83. [Crossref] [PubMed]
- Mimae T, Miyata Y, Tsutani Y, et al. Role of ground-glass opacity in pure invasive and lepidic component in pure solid lung adenocarcinoma for predicting aggressiveness. JTCVS Open 2022;11:300-16. [Crossref] [PubMed]
- Kobayashi Y, Ambrogio C, Mitsudomi T. Ground-glass nodules of the lung in never-smokers and smokers: clinical and genetic insights. Transl Lung Cancer Res 2018;7:487-97. [Crossref] [PubMed]
- Sanchez-Vega F, Mina M, Armenia J, et al. Oncogenic Signaling Pathways in The Cancer Genome Atlas. Cell 2018;173:321-337.e10. [Crossref] [PubMed]
- Kobayashi Y, Mitsudomi T, Sakao Y, et al. Genetic features of pulmonary adenocarcinoma presenting with ground-glass nodules: the differences between nodules with and without growth. Ann Oncol 2015;26:156-61. [Crossref] [PubMed]
- Lu Q, Ma Y, An Z, et al. Epidermal growth factor receptor mutation accelerates radiographic progression in lung adenocarcinoma presented as a solitary ground-glass opacity. J Thorac Dis 2018;10:6030-9. [Crossref] [PubMed]
- Li M, Xi J, Zhang H, et al. Pan-Driver-Negatives versus Epidermal Growth Factor Receptor Mutants for C-Stage IA Lung Adenocarcinoma with Ground-Glass Opacity. Ann Thorac Cardiovasc Surg 2022;28:320-8. [Crossref] [PubMed]
- Hattori A, Matsunaga T, Fukui M, et al. Prognosis of epidermal growth factor receptor-mutated stage I lung adenocarcinoma with radiologically solid features. Eur J Cardiothorac Surg 2022;61:769-77. [Crossref] [PubMed]
- Han B. Chinese expert consensus on immunotherapy for advanced non-small cell lung cancer with oncogenic driver mutations (2022 edition). Chin J Oncol 2022;44:1047-65. [Crossref] [PubMed]
- Donehower LA, Soussi T, Korkut A, et al. Integrated Analysis of TP53 Gene and Pathway Alterations in The Cancer Genome Atlas. Cell Rep 2019;28:1370-1384.e5. [Crossref] [PubMed]
- Stracquadanio G, Wang X, Wallace MD, et al. The importance of p53 pathway genetics in inherited and somatic cancer genomes. Nat Rev Cancer 2016;16:251-65. [Crossref] [PubMed]
- Mitsudomi T, Hamajima N, Ogawa M, et al. Prognostic significance of p53 alterations in patients with non-small cell lung cancer: a meta-analysis. Clin Cancer Res 2000;6:4055-63.
- Tsao MS, Aviel-Ronen S, Ding K, et al. Prognostic and predictive importance of p53 and RAS for adjuvant chemotherapy in non small-cell lung cancer. J Clin Oncol 2007;25:5240-7. [Crossref] [PubMed]
- Wade M, Li YC, Wahl GM. MDM2, MDMX and p53 in oncogenesis and cancer therapy. Nat Rev Cancer 2013;13:83-96. [Crossref] [PubMed]
- Foulkes WD, Flanders TY, Pollock PM, et al. The CDKN2A (p16) gene and human cancer. Mol Med 1997;3:5-20.
- Gutiontov SI, Turchan WT, Spurr LF, et al. CDKN2A loss-of-function predicts immunotherapy resistance in non-small cell lung cancer. Sci Rep 2021;11:20059. [Crossref] [PubMed]
- Yatabe Y, Borczuk AC, Powell CA. Do all lung adenocarcinomas follow a stepwise progression? Lung Cancer 2011;74:7-11. [Crossref] [PubMed]