Yanghepingchuan granule improves airway inflammation by inhibiting autophagy via miRNA328-3p/high mobility group box 1/Toll-like receptor 4 targeting of the pathway of signaling in rat models of asthma
Highlight box
Key findings
• This study reveals that Yanghepingchuan granules (YHPCG) inhibit the high mobility group box 1 (HMGB1)/Toll-like receptor 4 (TLR4)/nuclear factor κB (NF-κB) pathway by upregulating miR-328-3p, reducing the formation of autophagosomes, inhibiting autophagy, and effectively preventing the progression of lung inflammation.
What is known and what is new?
• While YHPCG have been extensively used as traditional Chinese medicine to treat inflammatory lung diseases, including asthma, their molecular mechanisms were unknown.
• The novelty here lies in the discovery that YHPCG treat airway inflammation in asthma by inhibiting autophagy via the miR-328-3p/HMGB1/TLR4/NF‐κB signaling pathway.
What is the implication, and what should change now?
• This finding not only unveils the molecular mechanism behind the anti-inflammatory effects of YHPCG, but also provides a potential new therapeutic approach aimed at curbing the inflammatory response in asthma by modulating specific microRNAs and signaling pathways. Our study offers fresh perspectives and guidance for further research into the development and optimization of therapeutic agents for asthma.
Introduction
Bronchial asthma is a common chronic noninfectious respiratory disease that is mainly caused by inflammation of the airways, which leads to a variety of clinical manifestations, such as chest tightness, coughing, wheezing, or dyspnea (1). As a common respiratory disease, a progressive asthma epidemic affects approximately 334 million people worldwide according to World Health Organization projections (2). Previous researches have demonstrated that the pathogenesis of asthma is closely related to changes in genetic mechanisms, the immune response, chronic airway inflammation, airway hyperresponsiveness, and airway remodeling (3,4). In recent years, Western medicine involving hormones has been the main method to treat asthma; however, the therapeutic effect of glucocorticoids on severe asthma is limited, and glucocorticoid resistance is common in asthma patients, thus resulting in the poor therapeutic effect of glucocorticoids (5,6). Therefore, it is essential to better understand the sophisticated mechanisms of asthma and to explore new beneficial alternatives.
Autophagy is a highly conserved intracellular catabolic pathway that is triggered by nutrient deprivation and stress; this pathway captures and degrades damaged proteins and organelles in lysosomes. The decomposition products are recycled into the cellular metabolic pathway to inhibit inflammation and contribute to the maintenance of cellular homeostasis. An emerging study has found that autophagy is linked with several inflammatory diseases because it modulates genetic and immune mechanisms (7). It is worth noting that autophagy disorders have a key role in the process of metabolic remodeling, thus further leading to airway inflammation (8,9). This nonhistone chromosomal protein is known as high mobility group box 1 (HMGB1), which is encoded by the human HMGB1 gene and can be regarded as being an immune regulator that promotes inflammation through autocrine and paracrine mechanisms (10,11). As an essential damage-associated molecular pattern (DAMP) protein, HMGB1 is involved in pathological processes, including pneumonia, arthritis, sepsis, and other diseases (7,12). Extracellular HMGB1 plays a vital role in tissue injury and cell death by interacting with important receptors such as receptors of advanced glycation end-products (RAGE), as well as toll-like receptors (TLRs), thereby contributing to mitogen-activated protein kinase and nuclear factor κB (NF-κB) activation (13). A study has reported that HMGB1 release is closely related to autophagy regulation in multiple diseases as an inducer of autophagy (14). Whether HMGB1 modulates autophagy in asthma-related airway inflammation and the underlying mechanisms remain to be elucidated.
TLRs are a group of family member of pattern recognition receptors (PRRs) belonging to TLR/interleukin (IL)-1 receptor superfamily (15), and that TLR4 signaling in epithelial cells may trigger T-helper 2 (Th2) immune responses by overexpression of TSLP (16). Previous study has demonstrated that a beneficial role for IL-1 receptor associated-kinase (IRAK)-M in attenuating Th2-dominated allergic airway inflammation through modulating macrophages overactivation and enhancement of antigen uptake by macrophage phagocytosis (17). In addition, TAK242, as a specific TLR4 antagonist that was initially developed for sepsis therapy, is a potent anti-inflammatory drug (18,19). However, the efficacy of this drug in the treatment of asthma remains unknown. Of note, microRNAs (miRNAs), as short noncoding RNAs, play a pivotal role in the regulation of posttranscriptional gene expression, thus resulting in many chronic airway diseases, such as asthma (20). A recent study has shown that miRNAs are essential for asthma and that miRNA dysregulation has an effect on bronchial epithelial cells (21). Moreover, a functional study documented that miR-146a contributes to airway remodeling after cytokine stimulation in asthmatic patients, which may be associated with the survival and proliferation of human bronchial epithelial cells (22). Additionally, it has been reported that miR-19a can be enhanced and associated with cell proliferation of bronchial epithelial lymphocytes in severe asthmatic patients (23). Recently, investigators have indicated that miR-328-3p, as a DNA damage response (DDR) mechanism factor, may be a potential therapeutic target for lung cancer, and the ectopic expression of miR-328-3p can induce DNA damage in A549 cells after irradiation (24). New evidence suggests that miR328 may be an effective regulator of the complex process of wound repair and can regulate airway epithelial injury repair (25). Although the role of miR-328-3p has been reported to play a vital role in repairing bronchial epithelial cell injury, its potential mechanism in asthma is still not fully understood.
Traditional Chinese medicine (TCM) treatment of bronchial asthma has a long history and has been widely used and proven to be effective (26-28). Accurate diagnoses combined with syndrome differentiation and treatment of TCM can achieve a satisfactory clinical effect. YHPCG has the effect of tonifying kidneys, promoting blood circulation, and eliminating phlegm; moreover, it consists of nine medicinal herb formulas. Our previous study found that YHPCG reduces the onset and severity of asthma by having anti-inflammatory properties (29). However, the underlying molecular mechanisms of YHPCG involvement in asthma remain unclear. To confirm this mechanism, in this study, we aimed to perform animal experiments with ovalbumin (OVA)-challenged asthmatic rats and investigated whether YHPCG could improve airway inflammation in asthmatic rats by regulating miRNA328-3p/HMGB1/TLR4-mediated autophagy to further explain the inflammatory and immune mechanisms of asthma. We present this article in accordance with the ARRIVE reporting checklist (available at https://jtd.amegroups.com/article/view/10.21037/jtd-23-1262/rc).
Methods
Animal feeding
In this study, forty male Sprague-Dawley rats (4–6 weeks of age) were weighed by the Henan Animal Experiment Center, which provided virus-free SD rats, and raised at 21–24 ℃ and 45–50% humidity in the Animal Experiment Center of Anhui University of Chinese Medicine with natural sunlight, free access to drinking water, and food. A protocol was prepared before the study without registration. Animal experiments were performed under a project license (No. AHUCM-rats-2019007) granted by the Ethics Committee of Anhui University of Chinese Medicine, in compliance with institutional guidelines for the care and use of animals.
Animal modeling establishment and grouping
One week after adaptive feeding, the rats with similar body weights were divided into two groups, and forty rats were randomly selected; the groups included the asthma group (thirty-two rats) and the normal control group (eight rats). A group of rats with asthma received a treatment consisting of a 1 mL sensitization mixture containing 10% OVA (Sigma Corporation, Lot No. AP0028, Shanghai, China), normal saline diluted with sterile water, and 100 mg sodium hydroxide of aluminum (Sinopharm Chemical Reagent Co., Ltd., No. 20190704, Shanghai, China) on the 1st and 8th day of modeling, with an intraperitoneal injection of 0.5 mL and a subcutaneous injection of 0.5 mL in the groin, respectively (0.25 mL on the left and right sides). After the first OVA administration, four groups of rats in the asthma group were created by using a random number table, including an asthma group, a YHPCG group, a TAK242 group, and a YHPCG + TAK242 group (with 8 rats in each group). Except for the normal group, the remaining groups of rats were placed in a glass atomizing box on the 15th day for atomization stimulation (1% OVA solution), with the rats being atomized for 30 minutes once a day for 14 days. Groups of rats with normal and asthmatic conditions received regular distilled water by gavage. The treatment groups received an injection of 7.74 g/kg (YHPCG group) (Sichuan New Green Pharmaceutical Technology Co., Ltd., Sichuan, China), 1 mg/kg (TAK242 group) (MedChenExpress Co., Ltd., Shanghai, China), and 7.74 g/kg +1 mg/kg (YHPCG + TAK242 group) on successive 14-day cycles once a day. After the rats were anesthetized with 3% pentobarbital in a 3 mg/kg intraperitoneal injection, the abdominal aortic blood of the rats was collected, and the left lung was fixed with 4% paraformaldehyde. The right lung was frozen at −80 ℃.
Periodic acid-Schiff (PAS) and hematoxylin-eosin (HE) staining
An embedded paraffin block of lung tissue was prepared by using 4% paraformaldehyde fixation. After being dehydrated with ethanol (Shanghai Guangnuo Chemical Technology Co., Ltd., Batch No. 20210914, Shanghai, China) and dewaxed with xylene (Tianjin Kaitong Chemical Co., Ltd., Batch No. 20210619, Tianjin, China), and following 10 minutes of soaking in periodic acid solution, the lung tissue sections were washed twice with distilled water and 70% ethanol and subsequently soaked for 1 hour and 15 minutes in Schiff solution. After dyeing with hematoxylin (Ebiogo Co., Ltd., Batch No. 09232110, Hefei, China), the transparent, neutral adhesive, and glass cap were eluted by using ethanol and xylene for gradient elution. Observations under a microscope showed pathological changes in the lungs of asthmatic rats. Furthermore, goblet cell proliferation was observed by using the double-blinding method. Increased mucus secretion was observed under a light microscope.
Enzyme-linked immunosorbent assay (ELISA) analyses
The levels of IL-4, IL-5, IL-13, CXCR3, and CCR4 cytokines in the serum of rats were detected via ELISA after the supernatant was taken from the aorta blood of rats after abdominal anesthesia.
Autophagosomes in lung tissue were observed under an electron microscope
Rat lung tissues were collected and fixed with 2.5% glutaraldehyde buffer for 4 h, washed with 0.1 mol/L phosphoric acid for 10 minutes, and washed 3 times for 10–15 minutes each. They were fixed with 1% osmium for 2 hours, dehydrated with ethanol, and embedded with epoxy resin. Subsequently, for 12 hours, they were baked in an oven at 60 ℃ after being placed in an oven at 30 ℃ overnight. The slices were approximately 50–100 nanometers thick.
Immunofluorescence staining
After the lung tissue sections were dried, three lines of xylene (5 minutes for each) and three lines of ethanol (3 minutes for each) were successively applied. Ethanol was washed, after which antigen high-pressure repair was performed. After washing with distilled water three times, hydrophobic rings were drawn. After incubation, the primary antibody was dropped and then incubated. The excess liquid was discarded, and the immunofluorescence secondary antibody was added for incubation. Anti-fluorescence quenching sealing tablets were used to seal the tablets.
Quantitative real-time polymerase chain reaction (qRT-PCR) to quantify gene-level expression
Lung tissue samples were cut into liquid nitrogen and ground into powder. The powder was collected, after which TRIzol (Life Technologies, No. 350508, Shanghai, China) was added to the crack. Trichloromethane was added to shake vigorously for 15 seconds and placed at room temperature for 5 minutes after the cracking was complete. Following centrifugation for 5 minutes, the ethanol solution was removed, and the dried RNA was precipitated for 5–10 minutes in air. To dissolve RNA, 20–30 µL of water was added without any RNA enzymes. The RNA solution was then preserved at −80 ℃ by repeatedly blowing air into it several times. After the concentration and purity of RNA were detected, cDNA was synthesized according to the reverse transcription kit instructions (TaKaRa, No. AL21115A, Beijing, China) as a fluorescent quantitative template and subsequently amplified and detected on the PCR instrument. The primer sequences used for the qRT-PCR, detailed in Table 1 and reaction conditions for PCR amplification were as follows: in amplification cycles, 95 ℃ was used to predenaturate for 1 minute, 95 ℃ was used to denature for 20 seconds, 60 ℃ was maintained for 1 minute, and 72 ℃ was used to anneal for 30 seconds. 2−ΔΔCt was used to compute and assess the mRNA expression of HMGB1, MyD88, TLR4, NF-κB, and miRNA328-3p by using β actin as an internal reference.
Table 1
Gene | Amplicon size (bp) | Forward primer (5'→3') | Reverse primer (5'→3') |
---|---|---|---|
β actin | 150 | CCCATCTATGAGGGTTACGC3 | TTTAATGTCACGCACGATTTC |
TLR4 | 95 | TAGCCATTGCTGCCAACATC | ACACCAACGGCTCTGGATAA |
HMGB1 | 168 | TGCCTCGCGGAGGAAAAT | CTCTGAGCACTTCTTGGAGA |
MyD88 | 96 | GCATGGTGGTGGTTGTTTCT | TCTGTTGGACACCTGGAGAC |
NF-κB | 78 | ACTGCTTTGACTCACTCCATA | AAGGTATGGGCCATCTGTTGA |
U6 | 94 | CTCGCTTCGGCAGCACA | AACGCTTCACGAATTTGCGT |
miR-328-3p | 66 | ACACTCCAGCTGGGCTGGCCCTCTCTGCCC | TGGTGTCGTGGAGTCG |
miR-328-3p-RT | CTCAACTGGTGTCGTGGAGTCGGCAATTCAGTTGAGACGGAA |
Western blotting analyses
The supernatant was collected after centrifugation, and the gel was configured. After 1 h of constant pressure electrophoresis on an electrophoresis apparatus (Shanghai Tieneng Technology Co., Ltd., No. EPS300, Shanghai, China), the membrane transfer solution was washed, blocking solution (5% skim milk powder) was added, and the membrane was sealed on a shaker at room temperature for 2 h. The primary antibodies against HMGB1 (Bioss Co., Ltd., No. AF11297418, Beijing, China) and TLR4 (Proteintech, No. 10010906, Wuhan, China) were incubated, and the secondary antibody was rinsed and incubated. The analytical membrane was then rinsed and analyzed with ImageJ software, which is a free and open-source image analysis software developed by the National Institutes of Health (NIH), USA.
Dual-luciferase reporter analyses
To obtain plasmids with wild-type (Wt HMGB1) and mutant-type (mutated HMGB1) sequences, we amplified wild-type and mutant sequences from the HMGB1 3' untranslated region (UTR) and cloned them into pGL3 luciferase reporter vectors (Promega, Beijing, China). HEK293T cells were plated on 96-well plates. In addition, HMGB1 was transfected with miR-328-3p simulators cotransfected with either the wt or mutant forms or simulated controls into HEK293T cells by using Lipofectamine 2000 (Invitrogen, Shanghai, China). Double luciferase reporter gene analysis was used to detect luciferase activity at 48 h after transfection with the Promega kit.
Statistical analysis
Data analysis was conducted by using SPSS version 25.0 (MBI, Institute, Armonk, NY, USA) and GraphPad Prism 6.0 (GraphPad Institute, San Diego, CA, USA). To compare multiple groups, an analysis of variance (ANOVA) was performed. The quantitative data were not normally distributed, and a nonparametric rank-sum test was used for multigroup comparison analysis. Finally, P<0.05 was defined as being statistically significant.
Results
Analysis of pathological experiments in rat lung tissue
First, we investigated the protective effects of YHPCG on asthmatic rat pathological impairments. Staining with HE demonstrated that the normal group’s bronchial structure was intact without evidence of inflammatory cells, and tube walls (the bronchial walls of the rats) from the normal group did not thicken. Substantial inflammatory infiltration and exudation were observed in the model group; moreover, epithelial cells were exfoliated, tube walls were thicker regarding smooth muscles, and inflammatory cells were more numerous, which indicated that the preparation of the asthma rat model was successful. Interestingly, there was a significant reduction in mucosal and lumen structure damage in the YHPCG treatment group (Figure 1A), which showed that epithelial degeneration necrosis and epithelial cell shedding were less severe. This is in agreement with our clinical observations that YHPCG is effective in treating asthma in rats. As shown in Figure 1B, PAS staining demonstrated hyperplasia of airway goblet epithelial cells and increased mucus secretion in the lung tissues of the model group. Furthermore, the YHPCG group was more effective at reducing airway mucus secretion and in improving collagen deposition under airway epithelial cells than the model group. The airway mucus secretion reserve and goblet epithelial cell metaplasia of asthmatic rats were significantly reduced by YHPCG plus TLR4 inhibitors compared with the TLR4 inhibitor group.
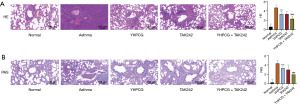
YHPCG improves inflammation in the serum of rats with bronchial asthma
A significant increase in proinflammatory cytokines such as IL-13, IL-4, IL-5, CXCR3, and CCR4 was observed in serum from the asthma model group. In the asthma group, YHPCG, TAK242, and YHPCG combined with TAK242 reversed the increased levels of IL-13, IL-4, IL-5, CXCR3, and CCR4 (Figure 2).
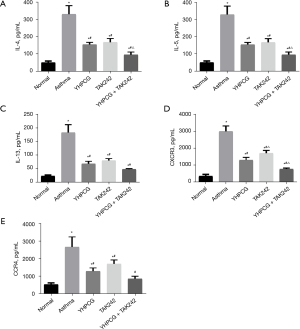
miRNA328-3p regulates asthma through HMGB1 targeting
RT-qPCR was used to confirm the presence of HMGB1 in asthma. The results in Figure 3A show a higher expression of HMGB1 in asthma than in normal controls. In the early stage, by collecting plasma samples from asthma patients, high-throughput sequencing was used to screen out miRNAs with differential expression, from which significantly high expression and low expression miRNAs were selected, among which 23 were upregulated, and 2 were downregulated. miR-328-3p is the type exhibiting upregulated expression and a significant difference in Figure 3B. Based on the conclusions of the correlation analysis, HMGB1 was inversely correlated with miR-328-3p. To confirm this effect, our investigation of miR-328-3p and HMGB1 confirmed its relationship, and an assessment of the mutation site design was conducted in the 3-UTRs of HMGB1 and miR-328-3p; As shown in Figure 3C on the left. In addition, a dual-luciferase reporter gene experiment was performed, according to this experiment, hsa-miR-328-3p significantly decreased luciferase expression in HMGB1-3UTR-WT compared to NC (P<0.01), thus indicating a binding effect between these two receptors. A successful mutation was demonstrated by the fact that hsa-miR-328-3p failed to inhibit the expression of luciferase in HMGB1-3UTR-Mut compared to NC. As shown in Figure 3C on the right. The expression levels of miR-328-3p in the asthma model group and the YHPCG-treated group were lower than those in the normal group; however, TAK242, a TLR4 inhibitor, attenuated this decrease. In addition, the combination of YHPCG + TAK242 also elevated the expression level of miR-328-3p. As shown in Figure 3D. In conclusion, HMGB1 expression was higher in asthmatics compared to normal controls, and an inverse correlation was found between HMGB1 and upregulated miR-328-3p.
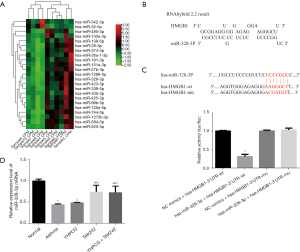
YHPCG inhibited autophagosomes in asthmatic rats
An electron microscope was used to observe autophagosomes, and the nucleus, mitochondria, and rough endoplasmic reticulum were examined in the normal group; however, no autophagosomes were observed. The structure of the cells in the model group was disordered and broken; moreover, the mitochondrial morphology was distorted and swollen, and autophagosomes were presented within the bilayer membrane. There was a decrease in autophagosomes and autophagic vesicles in the YHPCG groups and the TLR4 inhibitor groups. When compared to TLR4 inhibitors, the number of autophagosomes in the YHPCG plus TLR4 inhibitor groups was significantly decreased (Figure 4).

The suppressive effect of YHPCG on autophagy in an asthmatic rat model of inflammation
To determine whether YHPCG has an effect on autophagy in asthmatic model rats, we conducted an experiment. The immunostaining intensity of lung tissue from the asthma group was significantly increased compared with that of normal control tissue in the immunofluorescence analysis. As a result, these changes were significantly reduced when YHPCG, TAK242, or YHPCG and TAK242 were administered (Figure 5A). As shown in Figure 5B, western blotting was performed to evaluate the levels of the autophagy-related proteins LC3B-I and LC3B-II, Beclin, and ATG5 in lung tissue. The data showed that YHPCG suppressed autophagy in asthma model rats.
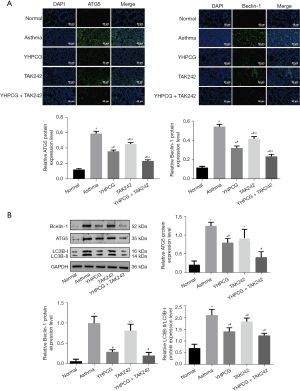
YHPCG suppresses pulmonary inflammation injury through the HMGB1/MyD88/TLR4/IκB/NF-κB signaling pathway
Our immunofluorescence analysis results showed that the protein expression of HMGB1, TLR4, p-NF-κB, and MyD88 in the lung tissue of normal rats is shown in Figure 6A. A higher expression level was observed in the asthma model group than in the normal group, as shown in Figure 6A; however, YHPCG treatment attenuated this increase. Furthermore, YHPCG + TAK242 treatment decreased the protein level, which implied that YHPCG can reduce inflammatory damage by inhibiting signaling pathways. As illustrated in Figure 6B, rat lung tissue samples were examined for mRNA expression of TLR4, MyD88, NF-κB, and HMGB1. A significant increase in all four mRNA levels was observed in the lungs of the asthma group compared to the normal group, but a significant reduction was observed in the lungs of the YHPCG-treated group compared with the asthmatic group. The results of the western blotting analysis distinctly showed that the HMGB1, TLR4, MyD88, and p-NF-κB protein expression levels of the asthma group were higher than those of the normal group, and the treatment group weakened the activation of the HMGB1 pathway signal and inhibited the progression of inflammation (Figure 6C). Taken together, YHPCG was involved in regulating signaling through TLR4 mediated by HMGB1 in the lung tissue of OVA-activated asthmatic rats.
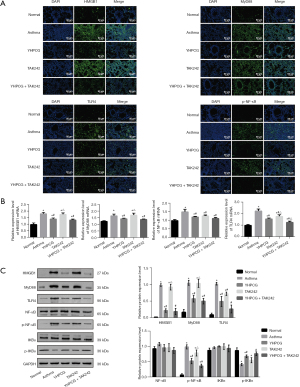
Discussion
Bronchial asthma can be triggered by genetics, allergies, and infections, among other factors. Chronic airway inflammation is characterized by various inflammatory cells, inflammatory chemokines, and large numbers of cytokines, which is also the basic pathological change in asthma (30). Airway inflammation is particularly critical in the development of asthma, which is characterized by damage to airway wall tissue, especially airway allergic inflammation. Currently, there is no clinically satisfactory treatment for asthma airway inflammation. Many studies have explored in depth the link between airway remodeling and inflammation. In general, airway remodeling is seen as a long-term result of chronic airway inflammation, which may be caused by factors such as smoking and allergies. When inflammation occurs, it may lead to the release of cytokines and growth factors, which can affect various cells and lead to structural changes in tissues. However, the relationship between airway remodeling and inflammation is not simply linear; they may co-exist, and airway remodeling is also associated with factors such as cell proliferation and apoptotic imbalance (31). Asthma is currently treated with a variety of effective drugs, including TCM. In a recent study, Yan et al. found that the wentong decoction may promote apoptosis in eosinophils, which is thought to be responsible for curing allergic asthma (32). YHPCG is a traditional hospital preparation that is made through the improvement of standardized production processes. Research results in clinical application have led to this prescription, which is based on long-term clinical verification and plays a vital role in the treatment of asthma. YHPCG is recognized for its properties to fortify the kidney, stimulate blood circulation, dissolve phlegm, and alleviate coughing and asthma symptoms (33). Its efficacy in the clinical management of bronchial asthma has been demonstrated over several years, with significant therapeutic outcomes (29). This study corroborates the potential of YHPCG in asthma treatment by impeding the PI3K/PKB pathway, curtailing the undue proliferation of airway smooth muscle cells (ASMCs), and rectifying kidney Yang deficiency. These findings unveil novel methodologies for treating asthma by targeting the PI3K/PKB signaling cascade and remedying kidney Yang inadequacy, thereby bolstering the clinical utilization of YHPCG (34). Further, the research indicated that YHPCG substantially mitigates the inflammatory cascade in the bronchial tissue of BA-afflicted rats, diminishes the levels of pro-inflammatory cytokines IL-1β and IL-18, and suppresses the articulation of pyroptosis mediators. In addition, YHPCG is capable of obstructing the TLR4/NF-κB signaling conduit. These insights propose that YHPCG might serve as a potent therapeutic agent for bronchial asthma by inhibiting the TLR4/NF-κB signaling trajectory and restraining pyroptosis (35). Asthma-related inflammation is known to be influenced by YHPCG, but little is known about its effect on airway inflammation. Hence, the purpose of the present study was to investigate the therapeutic effects of YHPCG on airway inflammation in asthmatic rats and to determine its molecular mechanism of action. Overall, the results of the current study suggest that YHPCG inhibits asthmatic inflammation in rats via the modulation of the HMGB1/TLR4 signaling pathway.
The HE staining results showed that asthma-prone rats had inflammatory cells around their lungs, as well as morphological changes and thickening of the bronchial wall. In agreement with previous findings (36), PAS staining demonstrated that asthmatic rats have a large number of PAS-positive goblet cells. Thus, an asthma model in rats was successfully established in the present study. As a next step, asthmatic rats stimulated by OVA were given exogenous TLR4 inhibitors. Our results showed that the use of YHPCG and TLR4 inhibitors may significantly improve the destruction of bronchial walls, reduce mucus secretion, and inhibit inflammation, hypertrophy of smooth muscles in the airways and submucosal gland, and hyperplasia in asthmatic rats stimulated by OVA. Moreover, cytokines and chemokines such as IL-5, IL-4, IL-13, CXCR3, and CCR4 were simultaneously downregulated. Based on these results, YHPCG may protect against OVA-induced airway inflammation.
Cellular recycling is achieved through autophagy, in which intracellular waste is transported from the lysosomes into autophagosomes, where it is degraded to maintain cellular balance. Inflammation in the lungs is caused by autophagy in both immune and nonimmune cells, wherein disruption or dysfunction is closely related to the development of a variety of inflammatory diseases, including asthma (37). In previous studies, autophagy has been linked to asthma pathogenesis, which is consistent with our current findings (38,39). The purpose of this study was to further investigate the effect of YHPCG on asthmatic rat lung autophagy. The administration of YHPCG orally suppresses asthma-induced autophagy, reduces autophagosome formation, and increases autophagy-related protein (LC3B-I, LC3B-II, Beclin-1, and ATG5) expression. YHPCG induced an inhibitory effect on autophagic flux when administered at a dosage of 7.74 g/kg rats, which may explain the attenuated asthmatic response.
A variety of chronic respiratory diseases, such as asthma, are caused by miRNAs (20). It has been demonstrated that miRNAs play key roles in the pathogenesis of asthma (40). MiR-155 stimulates eosinophils to migrate to the airways and regulates the Th2 response, thus resulting in allergic airway inflammation (41). The inhibition of IL-4 and IL-13 by miR-355-5p occurs via the downregulation of B7-H3 type 1 transmembrane protein (42). In our study, miR-328-3p was involved in asthma occurrence and development, with an interaction between miR-328-3p and HMGB1. It was found that miR-328-3p could activate downstream pathways by regulating HMGB1, as well as possess biological functions by targeting Beclin-1, promote autophagy levels, and aggravate asthma symptoms, in asthma model rats. YHPCG can inhibit the HMGB1/TLR4 signaling pathway, regulate autophagy, and improve inflammation of the airways by promoting miR-328-3p. The limitations of the experimental design limited the study to examine the relationship between the inhibition of HMGB1 downstream and autophagy; however, it did not confirm the direct association between upstream HMGB1 and autophagy, which could be verified via knockout experiments. Additionally, when considering the ceRNA mechanism, it is speculated that lincRNA or circRNA will exist upstream of miR-328-3p to regulate its expression, but the specific mechanism is unknown.
Conclusions
In conclusion, YHPCG may inhibit the activation of the HMGB1/TLR4 signaling pathway by promoting the expression of miRNA328-3p, inhibit the occurrence of autophagy in asthma, reduce the inflammatory response mediated by it, and regulate the immune imbalance, thus playing a role in the treatment of asthma. The results showed that YHPCG may increase the expression of miRNA328-3p, inhibit the protein levels of MyD88, HMGB1, TLR4 and NF-B, promote the expression of inflammatory factors IL-13, IL-4 and IL-5 and the secretion of goblet cells, and alleviate airway inflammation of asthma. Thus this study can enhance the understanding of the anti-inflammatory effect of YHPCG, which is conducive to the further rational application of YHPCG and provides new targets and new ideas for the clinical treatment of asthma.
Acknowledgments
We thank Prof. Yasuo Shimizu (Dokkyo Medical University School of Medicine, Tochigi, Japan) for the critical comments and valuable advice on this study.
Funding: The study was supported by the National Science Foundation of China (No. 81373600) and the Sixth Batch of Special Support Plan for Leading Innovative Talents in Anhui Province (No. T000614).
Footnote
Reporting Checklist: The authors have completed the ARRIVE reporting checklist. Available at https://jtd.amegroups.com/article/view/10.21037/jtd-23-1262/rc
Data Sharing Statement: Available at https://jtd.amegroups.com/article/view/10.21037/jtd-23-1262/dss
Peer Review File: Available at https://jtd.amegroups.com/article/view/10.21037/jtd-23-1262/prf
Conflicts of Interest: All authors have completed the ICMJE uniform disclosure form (available at https://jtd.amegroups.com/article/view/10.21037/jtd-23-1262/coif). All authors report funding support from the National Science Foundation of China (No. 81373600) and the Sixth Batch of Special Support Plan for Leading Innovative Talents in Anhui Province (No. T000614). The authors have no other conflicts of interest to declare.
Ethical Statement: The authors are accountable for all aspects of the work in ensuring that questions related to the accuracy or integrity of any part of the work are appropriately investigated and resolved. Animal experiments were performed under a project license (No. AHUCM-rats-2019007) granted by the Ethics Committee of Anhui University of Chinese Medicine, in compliance with institutional guidelines for the care and use of animals.
Open Access Statement: This is an Open Access article distributed in accordance with the Creative Commons Attribution-NonCommercial-NoDerivs 4.0 International License (CC BY-NC-ND 4.0), which permits the non-commercial replication and distribution of the article with the strict proviso that no changes or edits are made and the original work is properly cited (including links to both the formal publication through the relevant DOI and the license). See: https://creativecommons.org/licenses/by-nc-nd/4.0/.
References
- Mims JW. Asthma: definitions and pathophysiology. Int Forum Allergy Rhinol 2015;5:S2-6. [Crossref] [PubMed]
- Papi A, Brightling C, Pedersen SE, et al. Asthma. Lancet 2018;391:783-800. [Crossref] [PubMed]
- Rosenberg SL, Miller GE, Brehm JM, Celedón JC. Stress and asthma: novel insights on genetic, epigenetic, and immunologic mechanisms. J Allergy Clin Immunol 2014;134:1009-15. [Crossref] [PubMed]
- Hough KP, Curtiss ML, Blain TJ, et al. Airway Remodeling in Asthma. Front Med (Lausanne) 2020;7:191. [Crossref] [PubMed]
- Menzella F, Latorre M, Ruggiero P, et al. Reduction of oral corticosteroids in patients with severe eosinophilic asthma treated with Benralizumab: could it represent a marker of treatment efficacy? Expert Opin Biol Ther 2019;19:601-6. [Crossref] [PubMed]
- Fitzpatrick AM, Chipps BE, Holguin F, et al. T2-"Low" Asthma: Overview and Management Strategies. J Allergy Clin Immunol Pract 2020;8:452-63. [Crossref] [PubMed]
- Liu M, Shan M, Zhang Y, et al. Progranulin Protects Against Airway Remodeling Through the Modulation of Autophagy via HMGB1 Suppression in House Dust Mite-Induced Chronic Asthma. J Inflamm Res 2021;14:3891-904. [Crossref] [PubMed]
- He W, Xiong W, Xia X. Autophagy Regulation of Mammalian Immune Cells. Adv Exp Med Biol 2019;1209:7-22. [Crossref] [PubMed]
- Suzuki Y, Maazi H, Sankaranarayanan I, et al. Lack of autophagy induces steroid-resistant airway inflammation. J Allergy Clin Immunol 2016;137:1382-1389.e9. [Crossref] [PubMed]
- Hammad H, Lambrecht BN. Barrier Epithelial Cells and the Control of Type 2 Immunity. Immunity 2015;43:29-40. [Crossref] [PubMed]
- Gon Y, Hashimoto S. Role of airway epithelial barrier dysfunction in pathogenesis of asthma. Allergol Int 2018;67:12-7. [Crossref] [PubMed]
- Musumeci D, Roviello GN, Montesarchio D. An overview on HMGB1 inhibitors as potential therapeutic agents in HMGB1-related pathologies. Pharmacol Ther 2014;141:347-57. [Crossref] [PubMed]
- Imbalzano E, Quartuccio S, Di Salvo E, et al. Association between HMGB1 and asthma: a literature review. Clin Mol Allergy 2017;15:12. [Crossref] [PubMed]
- Zhang C, Hu J, Wang W, et al. HMGB1-induced aberrant autophagy contributes to insulin resistance in granulosa cells in PCOS. FASEB J 2020;34:9563-74. [Crossref] [PubMed]
- Medzhitov R, Preston-Hurlburt P, Janeway CA. A human homologue of the Drosophila Toll protein signals activation of adaptive immunity. Nature 1997;388:394-7. [Crossref] [PubMed]
- Li M, Wang ZN, Yang LF, et al. TLR4 antagonist suppresses airway remodeling in asthma by inhibiting the T-helper 2 response. Experimental and Therapeutic Medicine 2017;14:2911-6. [Crossref] [PubMed]
- Zhang X, Zhang M, Li L, et al. IRAK-M knockout promotes allergic airway inflammation, but not airway hyperresponsiveness, in house dust mite-induced experimental asthma model. J Thorac Dis 2021;13:1413-26. [Crossref] [PubMed]
- Meijvis SCA, van de Garde EMW, Rijkers GT, et al. Treatment with anti-inflammatory drugs in community-acquired pneumonia. J Intern Med 2012;272:25-35. [Crossref] [PubMed]
- Rice TW, Wheeler AP, Bernard GR, et al. A randomized, double-blind, placebo-controlled trial of TAK-242 for the treatment of severe sepsis. Crit Care Med 2010;38:1685-94. [Crossref] [PubMed]
- Stolzenburg LR, Harris A. The role of microRNAs in chronic respiratory disease: recent insights. Biol Chem 2018;399:219-34. [Crossref] [PubMed]
- Svitich OA, Sobolev VV, Gankovskaya LV, et al. The role of regulatory RNAs (miRNAs) in asthma. Allergol Immunopathol (Madr) 2018;46:201-5. [Crossref] [PubMed]
- Liu X, Nelson A, Wang X, et al. MicroRNA-146a modulates human bronchial epithelial cell survival in response to the cytokine-induced apoptosis. Biochem Biophys Res Commun 2009;380:177-82. [Crossref] [PubMed]
- Haj-Salem I, Fakhfakh R, Bérubé JC, et al. MicroRNA-19a enhances proliferation of bronchial epithelial cells by targeting TGFβR2 gene in severe asthma. Allergy 2015;70:212-9. [Crossref] [PubMed]
- Ma W, Ma CN, Zhou NN, et al. Up- regulation of miR-328-3p sensitizes non-small cell lung cancer to radiotherapy. Sci Rep 2016;6:31651. [Crossref] [PubMed]
- Narożna B, Langwinski W, Jackson C, et al. MicroRNA-328 is involved in wound repair process in human bronchial epithelial cells. Respir Physiol Neurobiol 2017;242:59-65. [Crossref] [PubMed]
- Wang R, Lin J. Analysis of the Mechanism of Zhichuanling Oral Liquid in Treating Bronchial Asthma Based on Network Pharmacology. Evid Based Complement Alternat Med 2020;2020:1875980. [Crossref] [PubMed]
- Wang L, Zheng X, Hui Y, et al. Adjuvant treatment with Xiaoqinglong formula for bronchial asthma: Protocol of systematic review and meta-analysis. Medicine (Baltimore) 2019;98:e17053. [Crossref] [PubMed]
- Su YH, Yu XY, Geng LM, et al. Oral administration versus iontophoresis of Sang Su decoction (a formula for dispersing lung to calm panting and resolve phlegm and for dredging collateral) in the treatment of acute bronchial asthma: A comparative study on their efficacy and impacts on the prognosis. Int J Clin Exp Med 2018;11:1305-11.
- Liu Y, Zhu H. Clinical study on Yang-He-Ping-Chuan granules treated chronic lung function duration particles bronchial asthma airway inflammation. Journal of Chengdu University of TCM 2014;37:52-5.
- Yang Y, Jia M, Ou Y, et al. Mechanisms and biomarkers of airway epithelial cell damage in asthma: A review. Clin Respir J 2021;15:1027-45. [Crossref] [PubMed]
- Blasi F, Bettoncelli G, Canonica GW, et al. The management of asthma in the phenotype and biomarker era: The proposal of a new diagnostic-therapeutic model. J Asthma 2016;53:665-7. [Crossref] [PubMed]
- Yan Y, Bao HP, Li CL, et al. Wentong decoction cures allergic bronchial asthma by regulating the apoptosis imbalance of EOS. Chin Med 2018;13:21. [Crossref] [PubMed]
- Zhu H, Wang K, Yang L, et al. Yanghe Pingchuan granule promotes BMSCs homing in asthmatic rats by upregulating miR-139-5p and downregulating Notch1/Hes1 pathway. Nan Fang Yi Ke Da Xue Xue Bao = Journal of Southern Medical University 2020;40:1703-11.
- Pan LY, Han YQ, Wang YZ, et al. Mechanism of Yanghe Pingchuan granules treatment for airway remodeling in asthma. Drug Design, Development and Therapy 2018;12:1941-51. [Crossref] [PubMed]
- Pan L, Chen Y, Jiang Y, et al. Yanghe Pingchuan Granules Alleviate Airway Inflammation in Bronchial Asthma and Inhibit Pyroptosis by Blocking the TLR4/NF-κB/NRLP3 Signaling Pathway. Mediators of Inflammation. 2022;2022:6561048. [Crossref] [PubMed]
- Tang X, Nian H, Li X, et al. Effects of the combined extracts of Herba Epimedii and Fructus Ligustrilucidi on airway remodeling in the asthmatic rats with the treatment of budesonide. BMC Complement Altern Med 2017;17:380. [Crossref] [PubMed]
- Painter JD, Galle-Treger L, Akbari O. Role of Autophagy in Lung Inflammation. Front Immunol 2020;11:1337. [Crossref] [PubMed]
- Xia F, Deng C, Jiang Y, et al. IL4 (interleukin 4) induces autophagy in B cells leading to exacerbated asthma. Autophagy 2018;14:450-64. [Crossref] [PubMed]
- Zhang Y, Do DC, Hu X, et al. CaMKII oxidation regulates cockroach allergen-induced mitophagy in asthma. J Allergy Clin Immunol 2021;147:1464-77.e11. [Crossref] [PubMed]
- Ariel D, Upadhyay D. The role and regulation of microRNAs in asthma. Curr Opin Allergy Clin Immunol 2012;12:49-52. [Crossref] [PubMed]
- Liang Z, Tang F. The potency of lncRNA MALAT1/miR-155/CTLA4 axis in altering Th1/Th2 balance of asthma. Biosci Rep 2020;40:BSR20190397. [Crossref] [PubMed]
- Zhang X, Zhao X, Sun H, et al. The role of miR-29c/B7-H3 axis in children with allergic asthma. J Transl Med 2018;16:218. [Crossref] [PubMed]