Mechanisms of limitation of oxygen delivery during veno-venous extracorporal membrane oxygenation
Ensuring adequate tissue oxygenation is a cornerstone of intensive care medicine. We here present a new integration of mechanisms of tissue oxygenation during veno-venous (V-V) extracorporal membrane oxygenation (ECMO) into a holistic physiologic model.
An integrated physiological model of tissue oxygenation describes oxygen delivery (DO2) as process of multiple steps: diffusion of the oxygen from the alveolus to the pulmonary capillary, convective transport via perfusion with oxygen mostly bound to hemoglobin (Hb), diffusion from capillary through tissue to the mitochondrium. As all steps occur in sequence, a limitation can occur on each of these steps (1-6).
We will first describe the integration of oxygen transport during convection and diffusion according to this model.
The global convective DO2 can be described as: DO2 = Q × CaO2 [Q: cardiac output (CO); CaO2: arterial oxygen content]. The main determinants of CaO2 are Hb concentration and arterial oxygen saturation (SaO2).
The oxygen consumption (VO2) according to the Fick principle can be described as: VO2 = Q × (CaO2 − CvO2) (CvO2: venous oxygen content).
The DO2 from the capillary to the mitochondrium with a certain diffusion capacity D can be described as: VO2 = D × (PcapO2 − PmitO2) (PcapO2: oxygen partial pressure in the capillaries; PmitO2: oxygen partial pressure at the mitochondrium).
If the partial pressure of oxygen at the mitochondrium can be neglected and the partial pressure of oxygen in the terminal capillaries is proportional to venous partial pressure of oxygen with a constant factor k, this can be described as: VO2 = D × k × PvO2 (PvO2: venous oxygen partial pressure).
If the dissolved oxygen is in equilibrium with the oxygen bound to Hb following the sigmoid oxygen dissociation curve, the connective and diffusive DO2 can be plotted on the same graph where VO2 is the ordinate and PvO2 is the abscissa. This model is consistent with the current understanding of the physiology of DO2 (5).
Patients with severe respiratory failure may experience a total loss of lung function. In these cases, V-V ECMO may provide the entire oxygen supply for the patients. If the Hb is considered constant, the CaO2 is determined by the maximal ECMO flow, because we consider the ECMO oxygenator to be capable of fully oxygenating all blood passing through the oxygenator.
In a subset of patients, a high CO that is significantly higher than the maximal achievable effective ECMO flow, will lead to a significant addition of deoxygenated venous blood, thus lowering the SaO2. There is controversy, if the addition of beta-blockers with the intention of lowering the CO and thus increasing the SaO2 is beneficial, because the total amount of oxygen delivered by ECMO is not changed (7).
To introduce our integrated model of tissue oxygenation during V-V ECMO in cases of total lung failure, we analyze two situations (Situation A and Situation B). We consider these situations to be completely equal regarding ECMO flow and all physiologic parameters and to differ only in regard to CO and SaO2
We make some simplifying assumptions:
- Situation A: high CO (QA), low CaO2 (CaO2A), DO2A = QA × CaO2A; Situation B: low CO (QB), higher CaO2 (CaO2B), DO2B = QB × CaO2B
- Under the conditions of a CO that is significantly higher than the ECMO flow, a properly functioning oxygenator and minimal recirculation we consider DO2A = DO2B
- VO2 remains constant and equal in both situations VO2A = VO2B = QA × (CaO2A − CvO2A) = QB × (CaO2B − CvO2B).
If we put these assumptions together, we get the following relationship: QA/QB = CaO2B/CaO2A = (CaO2B − CvO2B)/(CaO2A − CvO2A) = CvO2B/CvO2A.
That implies, that in Situation A with higher Q and lower CaO2, the absolute difference between CaO2 and CvO2 will be lower than in the low output situation, but the absolute value for CvO2 will still be lower in Situation A than in Situation B.
With these underlying assumptions we can generate a Wagner diagram depicting Situation A and Situation B (Figure 1). The intersections of the diffusion line with the curves of the convective transport are the values for maximal VO2 (VO2max).
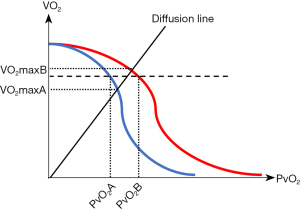
As it can be seen on the diagram, we consider the convective maximal DO2 to be identical in both situations. For any given VO2 (broken line in Figure 1) the PvO2 in Situation A will be lower than the PvO2 in Situation B, because the oxygen content in any given volume of blood will be higher in Situation B.
If we now introduce the diffusion capacity in our model, we see that the VO2max on the diffusion step is higher in Situation B, because the concentration gradient of O2 between the capillary and the mitochondrium is higher.
Since a limitation on the diffusion step has been described in past research (8,9), we consider it to be an important component of a holistic concept of DO2 into the tissues.
While values for arterial oxygen partial pressure (PaO2) around 60 mmHg have been considered safe in past clinical trials (HOT-ICU, ICU-ROX), the LOCO2 trial with a target of 55–70 mmHg in the conservative oxygen group was stopped early because of an increased number in mesenterial ischemia (10-12).
Our hypothesis is, that in PaO2 values below the lower threshold in the past trials, a diffusion limitation might lead to tissue hypoxia in patients with low CaO2 values despite an identical convective DO2. Diffusion limitation as the limiting factor of DO2 has previously been described (8,13,14). We therefore suggest, that the use of medications to decrease the CO in situations of total lung failure and ECMO support might decrease the risk of tissue hypoxia by accounting for the risk of a limitation on the diffusion step. The use of a short acting medication like esmolol is preferable in this situation in cases where adverse events are observed.
If there is some lung function left, this concept does not apply, since the potential influence of changes in CO on the ventilation/perfusion mismatch have to be taken into account (15). Furthermore, especially in the context of a femoro-jugular cannulation, recirculation might increase with a decrease in CO (16). In this situation the use of beta-blockers to reduce CO may be hazardous. Our concept therefore only applies to a situation where minimal recirculation can be achieved as with a bi-caval dual lumen cannula. In this case recirculation may be negligible (17). If the effect of the admixture of deoxygenated blood is also taken into account, the PvO2 of the venous blood will also be higher in Situation B as we calculated above.
While a previously discussed mathematical model suggests, that the convective DO2 is improved in the situation of increased CO and reduced SaO2, this does not take into account the following steps of tissue oxygenation (7). Furthermore, a decrease in CO might worsen perfusion on the microvascular level. To our knowledge, there is currently no established cut-off for CO and CaO2 to decide when lowering CO and thus increasing diffusion is beneficial.
There are multiple possible options to solve this question, including data mining of hemodynamic and blood gas data from patients treated with V-V ECMO, mathematical modelling of tissue perfusion and diffusion or models using animal tissue for direct testing.
Further data is needed to evaluate whether the effects predicted by this model have a clinically meaningful effect.
Acknowledgments
Funding: None.
Footnote
Peer Review File: Available at https://jtd.amegroups.com/article/view/10.21037/jtd-23-800/prf
Conflicts of Interest: All authors have completed the ICMJE uniform disclosure form (available at https://jtd.amegroups.com/article/view/10.21037/jtd-23-800/coif). D.R. receives an authors’ salary from Springer Verlag for having published a medical textbook. The other authors have no conflicts of interest to declare.
Ethical Statement: The authors are accountable for all aspects of the work in ensuring that questions related to the accuracy or integrity of any part of the work are appropriately investigated and resolved. No human or animal experiments have been performed for this work.
Open Access Statement: This is an Open Access article distributed in accordance with the Creative Commons Attribution-NonCommercial-NoDerivs 4.0 International License (CC BY-NC-ND 4.0), which permits the non-commercial replication and distribution of the article with the strict proviso that no changes or edits are made and the original work is properly cited (including links to both the formal publication through the relevant DOI and the license). See: https://creativecommons.org/licenses/by-nc-nd/4.0/.
References
- Wagner PD. Modeling O2 transport as an integrated system limiting (.)V(O2MAX). Comput Methods Programs Biomed 2011;101:109-14. [Crossref] [PubMed]
- Wagner PD. CrossTalk proposal: Diffusion limitation of O2 from microvessels into muscle does contribute to the limitation of V̇O2 max. J Physiol 2015;593:3757-8. [Crossref] [PubMed]
- Cano I, Roca J, Wagner PD. Effects of lung ventilation-perfusion and muscle metabolism-perfusion heterogeneities on maximal O2 transport and utilization. J Physiol 2015;593:1841-56. [Crossref] [PubMed]
- Wagner PD. Operation Everest II and the 1978 Habeler/Messner ascent of Everest without bottled O2: what might they have in common? J Appl Physiol (1985) 2017;123:1682-8. [Crossref] [PubMed]
- Poole DC, Musch TI. Capillary-Mitochondrial Oxygen Transport in Muscle: Paradigm Shifts. Function (Oxf) 2023;4:zqad013. [Crossref] [PubMed]
- Wagner PD. Career perspective: Peter D Wagner. Extrem Physiol Med 2013;2:31. [Crossref] [PubMed]
- Álvarez DR, Pérez-Costa E, Suso JJM. Dangers in using beta-blockers in patients with venovenous extracorporeal membrane oxygenation. Acute Crit Care 2022;37:683-4. [Crossref] [PubMed]
- Esposito F, Mathieu-Costello O, Shabetai R, et al. Limited maximal exercise capacity in patients with chronic heart failure: partitioning the contributors. J Am Coll Cardiol 2010;55:1945-54. [Crossref] [PubMed]
- Esposito F, Reese V, Shabetai R, et al. Isolated quadriceps training increases maximal exercise capacity in chronic heart failure: the role of skeletal muscle convective and diffusive oxygen transport. J Am Coll Cardiol 2011;58:1353-62. [Crossref] [PubMed]
- ICU-ROX Investigators and the Australian and New Zealand Intensive Care Society Clinical Trials Group. Conservative Oxygen Therapy during Mechanical Ventilation in the ICU. N Engl J Med 2020;382:989-98. [Crossref] [PubMed]
- Schjørring OL, Klitgaard TL, Perner A, et al. Lower or Higher Oxygenation Targets for Acute Hypoxemic Respiratory Failure. N Engl J Med 2021;384:1301-11. [Crossref] [PubMed]
- Barrot L, Asfar P, Mauny F, et al. Liberal or Conservative Oxygen Therapy for Acute Respiratory Distress Syndrome. N Engl J Med 2020;382:999-1008. [Crossref] [PubMed]
- Dhakal BP, Malhotra R, Murphy RM, et al. Mechanisms of exercise intolerance in heart failure with preserved ejection fraction: the role of abnormal peripheral oxygen extraction. Circ Heart Fail 2015;8:286-94. [Crossref] [PubMed]
- Menon DK, Coles JP, Gupta AK, et al. Diffusion limited oxygen delivery following head injury. Crit Care Med 2004;32:1384-90. [Crossref] [PubMed]
- Droogh JM, Oude Lansink A, Renes MH, et al. In veno-venous ECMO oxygen delivery should be the focus. J Crit Care 2019;54:76. [Crossref] [PubMed]
- Charbit J, Deras P, Courvalin E, et al. Structural recirculation and refractory hypoxemia under femoro-jugular veno-venous extracorporeal membrane oxygenation. Artif Organs 2021;45:893-902. [Crossref] [PubMed]
- Parker LP, Svensson Marcial A, Brismar TB, et al. Hemodynamic and recirculation performance of dual lumen cannulas for venovenous extracorporeal membrane oxygenation. Sci Rep 2023;13:7472. [Crossref] [PubMed]