Comparative bioinformatics analysis of transcriptomes between β-aminopropionitrile-induced aortic dissection murine model and human aortic dissection
Highlight box
Key findings
• We illustrated the similarities and differences by comparing the transcriptome data of aortic dissection (AD) animal model and patients. Related pathways, and potential drugs were revealed. Hub genes were identified and validated by immunohistochemistry.
What is known and what is new?
• AD is a life-threatening medical emergency caused by stress and abnormalities of the medial layer of the aortic wall. In recent years, β-aminopropionitrile (BAPN)-induced AD in rodents has been widely used in basic research.
• This study revealed the transcriptional changes in BAPN-induced AD murine model in detail. We identified the important hub genes, related pathways, and potential drugs by analyzing the overlapping differentially expressed genes.
What is the implication, and what should change now?
• This study showed a high degree of similarity between BAPN induced AD model and human AD. The hub genes, signaling pathways and potential drugs can be used for subsequent in vitro and in vivo studies to further reveal the pathogenesis of AD and drug modification.
Introduction
Aortic dissection (AD) is a fatal vascular disease characterized by the separation of the intima from the media caused by an intimal tear and resulting in aortic bleeding or blood flow into the aortic wall forming a false lumen which rapidly expands within the aorta (1,2). AD usually causes sudden persistent intense pain. The mortality rate associated with aortic rupture secondary to acute AD is as high as 90% (3). End organ malperfusion caused by AD is also life-threatening (4,5). The exact mechanism of AD formation is not fully understood. Therefore, a better understanding of the molecular mechanisms and pathophysiology of AD will aid the development of new diagnostic and therapeutic strategies and improve clinical outcomes.
One factor that greatly restricts research into the mechanism of action of AD is that clinical samples are difficult to obtain. Multiple AD experimental animal models have been generated to mimic the spontaneous development of AD, investigate its pathogenesis, and identify novel therapeutic targets and measurements, including drug- or chemical-induced experimental models, genetically modified experimental models, and surgically or invasively induced experimental models (6-8). In recent years, β-aminopropionitrile (BAPN)-induced AD in rodents has been widely used in basic research (9-11). This model is simple, is inexpensive, and can be implemented without genetic engineering, which provides a good platform for exploring the pathogenesis of AD and drug modification.
This study aimed to reveal transcriptional changes in BAPN-induced AD murine model in detail for the first time. It also aimed to compare the similarities and differences between the murine AD model and human AD models in the transcriptome. We present this article in accordance with the ARRIVE reporting checklist (available at https://jtd.amegroups.com/article/view/10.21037/jtd-23-981/rc).
Methods
Murine AD model and sample collection
Male C57BL/6J mice (3 weeks old; mean 10±2 g each) were purchased from Vital River Laboratory Animal Technology Co., Ltd. (Beijing, China). The mice were housed under controlled conditions of 55%±5% humidity and 22±2 ℃ under a 12 hours light/dark cycle with ad libitum access to food and water. All animals underwent adaptive feeding for 3 days before further treatment. All experiments involving animal treatment were performed in accordance with the National Institutes of Health Guide for the Care and Use of Laboratory Animals, and the protocol was approved by the Ethics Committee of Union Hospital affiliated with Huazhong University of Science and Technology (No. 2636).
All mice were randomly divided into two groups (n=5) by drawing lots: control group and AD group. The mice were fed a normal diet and freshly prepared BAPN solution (Sigma-Aldrich, St. Louis, MO, USA) dissolved in drinking water (1 g/kg/day) for 4 weeks to induce AD as described previously (12). The control group was not treated with BAPN. Mice surviving at the end of the experiment were sacrificed with an overdose of sodium pentobarbital, and tissue samples were collected for further analysis. Five AD tissues and five control tissues were subjected to RNA extraction. One control sample was discarded because its RNA concentration did not meet the standards. We then constructed the library and subsequently sequenced these nine samples (four controls, five ADs).
Ultrasound observation
The ultrasound observation was carried out on the 28th day for survival mice through a high-resolution Vevo 2100 microimaging system with an MX400 linear array transducer at 30 MHz (VisualSonics, Toronto, Canada). A thoracic aortic diameter increase of ≥50% was considered as aneurysm secondary to the dissection.
RNA extraction, library preparation, and sequencing
Total RNA was extracted using TRIzol reagent (cat. No. AG21101; Accurate Biotechnology Co., Guangzhou, China) as described by Chomczynski et al. (13).
Two micrograms of total RNA were used for stranded RNA sequencing library preparation using a KCTM Stranded mRNA Library Prep Kit for Illumina® (Catalog No. DR08402; Wuhan SeqHealth Co., Ltd., Wuhan, China) according to the manufacturer’s instructions. Polymerase chain reaction products corresponding to 200–500 base pairs were enriched, quantified, and sequenced on a NovaSeq 6000 sequencer (PE150 model; Illumina, Wuhan, China). Raw sequencing data were first filtered using Trimmomatic tool (version 0.36), low-quality reads were discarded and the reads contaminated with adaptor sequences were trimmed, and clean data were mapped to the reference genome of Mus musculus using STRA software (version 2.5.3a) with default parameters. Reads mapped to the exon regions of each gene were counted using featureCounts (Subread-1.5.1; Bioconductor, Massachusetts, USA), and reads per kilobase of exon per million reads mapped were calculated. Raw RNA-seq data of the murine AD model were analyzed.
Murine and human AD data sources
The murine RNA-seq data in this study can be found in National Center for Biotechnology Information (NCBI)’s Sequence Read Archive (accession no. PRJNA903222; http://www.ncbi.nlm.nih.gov/bioproject/903222). The dataset contained nine samples (four controls, five ADs).
The human AD dataset (GSE147026) was extracted from the NCBI Gene Expression Omnibus database (https://www.ncbi.nlm.nih.gov/geo/). Raw bioinformatics RNA-seq gene counts were obtained from GSE147026 using the GPL24676 platform (Illumina NovaSeq 6000; Homo sapiens). The dataset contains 10 samples (five controls, five ADs).
Differential expression analysis
We analyzed the differentially expressed genes (DEGs) using the DESeq package of R software (version 4.0.1; https://www.rproject.org/) (14). The significant criteria for identifying DEGs with a low false discovery rate were set as adjusted P values <0.001 and |log2(fold change)| ≥1.
Functional and pathway enrichment analysis
Gene ontology (GO) and Kyoto Encyclopedia of Genes and Genomes (KEGG) enrichment analyses were performed to analyze the functional enrichment of the DEGs associated with the biology underlying AD. GO terms with corrected P values <0.05 and KEGG pathways with P values <0.05 were defined as significant enrichments. The R packages ggplot2, enrichplot, and GOplot were used to create the bubble plot and circle plot of the GO and KEGG pathway annotation.
Construction of protein-protein interaction (PPI) network and identification of hub genes
A PPI network based on the DEGs was constructed using STRING (https://string-db.org/), with the cut-off standard as a combined score >0.95. Subsequently, PPI networks were exported from STRING and imported into Cytoscape. To identify the hub genes, we ranked all listed genes according to their degrees calculated in the PPI and co-expression networks. The hub genes in the PPI network were defined as those with the highest degree of connectivity. The Molecular Complex Detection (MCODE) app was used to identify significant modules in the PPI network.
Morphometric and immunohistochemical analysis
The tissues were fixed in 4% paraformaldehyde for 48 hours, transferred to 70% ethanol, processed in a graded series of ethanol solutions, embedded in paraffin, and cut into 3-µm-thick sections. The slices were dewaxed, dehydrated, and repaired using standard methods. The slices were blocked and treated with the target primary antibody and goat anti-rabbit/mouse immunoglobulin G. The slices were then dripped with Streptomyces ovalbumin protein labeled with horseradish peroxidase, and the slices were developed using diaminobenzidine. Hematoxylin was used as the counterstain. Gene expression was calculated as the integrated optical density of the yellow-brown-stained area using Image Pro Plus. The integrated optical density value was divided by the area of the target distribution region to obtain the average optical density.
Identification of potential drugs
Enrichr is a comprehensive web-based tool used for gene set enrichment analysis (15). It currently contains a large genome library available for analysis and download, containing 180–184 annotated gene sets from 102 gene set libraries. Drug Gene Interaction Database version 3.0.2 (https://www.dgidb.org) is a resource of drug-targeted and -sensitive genomes along with drug-gene interactions (16). To explore potential drugs, DSigDB in the Enrichr online database was used to analyze the potential drug targets of the overlapping DEGs.
Statistical analysis
Data were presented as mean ± standard deviation (SD). All data were analyzed using GraphPad Prism 8 software (GraphPad Software, La Jolla, CA, USA). Analysis of variance with repeated measures followed by a post hoc Bonferroni correction or Student’s two-sided t-test was used. For all comparisons, P values of <0.05 were considered statistically significant.
Results
Pathological changes and validation of murine AD model
AD and expansion were clearly observed in the murine model. Macroscopic pictures and ultrasound images showed the dissection aneurysm in BAPN-induced murine model (Figure 1A,1B). Hematoxylin and eosin staining showed tearing of the aortic wall and thrombi in the false lumen (Figure 1C). Elastica van Gieson (EVG) staining confirmed extensive elastic fiber fragmentation and depletion as well as loss of smooth muscle cells (Figure 1D). All of these pathological changes were similar to those observed in humans.
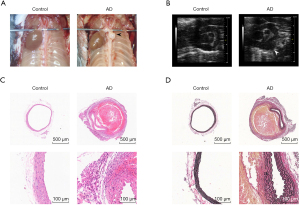
Gene expression levels in murine AD model
Principal component analysis (PCA) and principal coordinate analysis (PCoA) cluster analysis indicated significant differences between the AD and control groups (Figure 2A,2B). A total of 2,060 DEGs were identified, of which 1,467 were significantly upregulated and 593 were significantly downregulated (Figure 2C). We conducted GO enrichment analysis of the functions of the DEGs, including biological processes, cellular components, and molecular functions, to reveal the AD-related functional annotated terms. The top 10 are listed in Figure 2D.
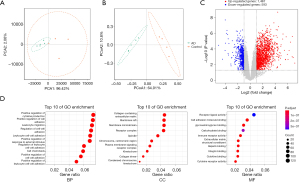
GO enrichment analysis revealed that DEGs were significantly enriched in biological processes, which involved positive regulation of cytokine production, positive regulation of cell adhesion, leukocyte migration, regulation of cell-cell adhesion, leukocyte cell-cell adhesion, and positive regulation of response to external stimuli. The DEGs also had a certain degree of enrichment in cellular components, including collagen-containing extracellular matrix, membrane raft, membrane microdomain, and receptor complex. The DEGs were enriched in molecular functions such as receptor ligand activity and cell adhesion molecule binding (Figure 2D).
Transcriptomes in murine AD model versus human AD
A total of 463 overlapping DEGs were identified (Figure 3A), of which 436 were significantly upregulated and 27 were significantly downregulated. Top 10 are listed in Table 1. The top 10 DEGs that were up- and down-specific for the murine and human samples are shown in Table 2. Wilcoxon’s rank-sum test of the relative expression data was performed for these 463 genes, which exhibited similar fold differential gene expression (Figure 3B). The heat map of the relative abundance of the 463 genes indicated that the murine AD model and human samples could be clustered accurately and had a similar clustering trend (Figure 3C).
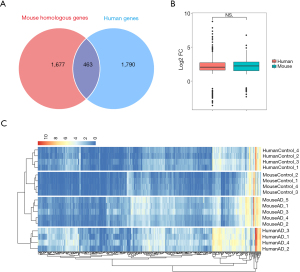
Table 1
Mouse genes | Homologous humanized genes | Log2FC |
---|---|---|
Spp1 | SPP1 | 6.757066819 |
Cemip | CEMIP | 5.262837185 |
Sirpb1a | SIRPA | 4.878752864 |
Mefv | MEFV | 4.589413818 |
Il1b | IL1B | 4.482047024 |
Tnfsf8 | TNFSF8 | 4.398257542 |
Cd300lf | CD300LF | 4.38701881 |
Bcl2a1b | BCL2A1 | 4.374588079 |
Cd300c2 | CD300C | 4.360359029 |
Havcr2 | HAVCR2 | 4.33306541 |
FC, fold change.
Table 2
Rank | Top 20 DEGs found only in human | Top20 DEGs found only in mouse | |||
---|---|---|---|---|---|
Gene name | Log2FC | Gene name | Log2FC | ||
1 | RASEF | −6.1624616 | Elovl3 | −5.078267114 | |
2 | ZNF536 | −6.085417764 | RP24-423G4.1 | −4.462431279 | |
3 | SOX10 | −5.951067139 | Gm3617 | −4.043266057 | |
4 | KCNT1 | −5.896028087 | Npas2 | −4.0182424 | |
5 | PCSK2 | −5.471847111 | 4631405J19Rik | −3.993797734 | |
6 | CYP4B1 | −5.347672248 | Arntl | −3.832143679 | |
7 | C10orf71 | −5.289514007 | Slc25a34 | −3.737851562 | |
8 | NRXN1 | −5.280965504 | Tmem82 | −3.626290672 | |
9 | SHISA9 | −5.033836557 | Sgk2 | −3.559600041 | |
10 | CDH8 | −4.992518818 | AI507597 | −3.543698101 | |
11 | MT1G | 5.9848049 | Nsg2 | 5.647148059 | |
12 | RORC | 6.067480204 | Gm13054 | 5.65142433 | |
13 | CXCL5 | 6.434200054 | Col10a1 | 5.769415812 | |
14 | SLAMF9 | 6.477445768 | Ighg2b | 5.953078797 | |
15 | IFNE | 6.566962218 | Nppa | 6.06784074 | |
16 | MCHR1 | 6.647742303 | Cfi | 6.240984848 | |
17 | CALB2 | 6.992393228 | Gm42793 | 6.461646172 | |
18 | CXCL3 | 7.080137544 | Saa3 | 6.735078572 | |
19 | FP565260.3 | 7.289973597 | Arg1 | 6.892727994 | |
20 | SLC28A3 | 7.516394822 | Npy | 7.159961069 |
DEGs, differentially expressed genes; FC, fold change.
Analysis of the 463 co-expressed genes in the KEGG pathway revealed that the cytokine-cytokine receptor interaction, tuberculosis, and phagosome pathways were significantly enriched and may interact with other pathways (Figure 4A,4B).
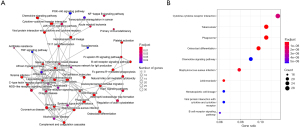
We performed GO enrichment analysis of the murine- and human-specific DEGs. Human-specific DEGs showed the highest enrichment in T cell activation, muscle system processes, and cell-cell adhesion regulation. Meanwhile, in the murine-specific DEGs, positive regulation of cell adhesion, leukocyte migration, and regulation of response to external stimuli had the highest enrichment degree (Figure 5A,5B).
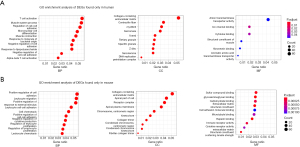
To further demonstrate the differences in physiological processes involved in murine- and human-specific DEGs, we performed a network analysis of all enriched GO pathways (Figure S1).
PPI network and module construction and hub gene analyses
To explore the interplay among DEGs in the different groups, PPI networks were built using the STRING tool with confidence >0.95 as the cut-off criterion (Figure 6A). Furthermore, according to the topological property analysis of the PPI network, we found that CDC20, CCNB2, and CCNB1 were the top three nodes with the greatest number of connections with other nodes (Figure 6B). Using the MCODE app, we found three clusters in the network. The first cluster, which had the highest score, contained nine nodes and 31 edges (Figure 6C). The second cluster, which contained four nodes and six edges, also exhibited a strong connection (Figure 6D). The third cluster contained three nodes and three edges (Figure 6E). These findings strongly suggest that these hub genes play vital roles in the pathogenesis of AD.
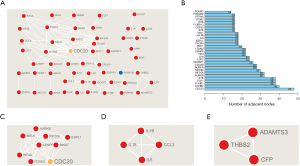
Hub gene validation
Owing to the complexity of obtaining clinical samples of human AD, we examined the protein levels of hub genes in the murine AD model tissues. Immunohistochemistry was used to assess the expression of the top three hub genes: CDC20, CCNB2, and CCNB1 (Figure 7). The protein expression levels of these genes were significantly increased in the AD group.
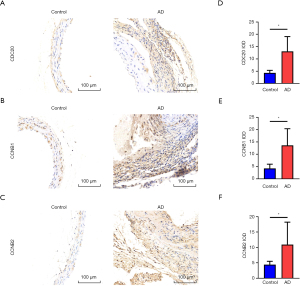
Identification of potential drugs
Assessment of protein-drug interactions is essential for the identification of important therapeutic targets for diseases (17,18). Targeting the overlapping DEGs in the murine and human AD models, we identified 10 possible drug molecules using Enrichr based on transcriptome signatures from the DSigDB database. The top 10 chemical compounds were extracted based on their P values (Table 3).
Table 3
Name | P value | Chemical formula |
---|---|---|
Mebendazole | 5.13E−30 | C16H13N3O3 |
Vorinostat | 3.28E−28 | C14H20N2O3 |
Etoposide | 8.03E−28 | C29H32O13 |
Calcitriol | 2.32E−26 | C27H44O3 |
Tamibarotene | 2.47E−25 | C22H25NO3 |
Trichostatin | 1.96E−24 | C17H22N2O3 |
Retinoic acid | 7.49E−24 | C20H28O2 |
Phorbol | 7.08E−23 | C20H28O6 |
Sodium dichromate | 1.96E−22 | H4Cr2Na2O9 |
Anisomycin | 2.81E−22 | C14H19NO4 |
Discussion
AD is a cardiovascular emergency with a high mortality rate. The most common causes of AD are arterial hypertension, connective tissue disease, vascular injury, and atherosclerosis (19-21). The incidence of AD is as high as 5 per 100,000 people, a rate that has increased dramatically over the past decade (22-25). Seasonal variation has an important effect on the incidence of AD, which increases significantly during winter (26). In addition, heavy physical labor and emotional excitement can increase blood pressure and induce AD (27). The pathogenesis of AD includes phenotypic switching and death of vascular smooth muscle cells, abnormal degradation of the extracellular matrix, endothelial dysfunction, and immune abnormalities (28). Although research on the etiology and mechanism of AD has made some progress, the exact mechanism of AD formation is not fully understood. Therefore, a better understanding of the molecular mechanisms of AD will aid our understanding of its pathophysiology, develop new diagnostic and therapeutic strategies, and improve clinical outcomes.
At present, a variety of experimental animal models of AD have been used to simulate the spontaneous development of AD, study its pathogenesis, and identify new therapeutic targets and methods for it; these mainly include surgical-induced experimental models and experimental models targeting pathogenic genes (compound induction or gene editing technology) (8). The existing microsurgery-induced AD animal models are established by surgical or intradural approaches to achieve the same appearance as of dissection. However, this model is similar to traumatic or iatrogenic AD in pathophysiological aspects but quite different from the occurrence and development of spontaneous AD. In addition, the establishment of this type of model depends on the operator’s surgical skills, which has the disadvantages of complex operation, difficulty in establishing modeling standards, and poor repeatability. Here we constructed a murine model of BAPN-induced AD. BAPN, a lysyl oxidase inhibitor, is the most widely used chemical compound for inducing AD in growth period mice and rats as it inhibits the cross-linking of collagen fibers (29). This model is simple, inexpensive, and can be implemented without genetic engineering of animals, which provides a good platform for exploring the pathogenesis and drug modification of AD.
This study constructed a murine model of BAPN-induced AD. On the one hand, anatomical dissection showed that, after a 4-week BAPN intervention, the mice showed obvious thrombolysis of the false lumen and dilation of the inner blood vessel walls. In contrast, hematoxylin and eosin staining showed blood clots in the tearing aortic wall after BAPN administration. EVG staining showed elastic fiber layer rupture and smooth muscle cell loss in the BAPN-treated group. These results again show that the pathological aspects of BAPN-induced AD are similar to those of spontaneous dissection. In the subsequent sequencing analysis, we observed an obvious clustering effect between the disease and normal groups of this model through PCA, which proved considerable model stability.
A total of 2,060 DEGs were identified and GO enrichment analysis showed that DEGs were significantly enriched in the immune and inflammatory pathways, including positive regulation of cytokine production, positive regulation of cell adhesion, leukocyte migration, regulation of cell-cell adhesion, leukocyte cell-cell adhesion, and other pathways.
We subsequently analyzed the intersecting genes of a BAPN-induced animal model and human AD. The homologous genes in humans and animals are highly conserved. In contrast, the differential genes obtained from disease and animal models helped us verify the important role of this molecule in AD. Among these intersecting genes, we found that many play a preliminary role in basic research on interlayers. For example, the most differentiated gene, spp1, expresses a bone-specific salivary protein in endothelial and smooth muscle cells and is upregulated in fibrosis, autoimmune diseases, and atherosclerosis. A study has shown that serum and local osteopontin expression levels are significantly increased in AD patients. It has also shown that osteopontin affects the progression of the interlayer by enhancing matrix metalloprotein (MMP)-2 gene expression and activity in human vascular smooth muscle cells and affecting extracellular matrix secretion (30). The enrichment analysis revealed the highest degree of enrichment in cytokine-cytokine receptor interactions. Cytokines are secreted proteins produced after immune stimulation. A variety of cytokines, including interleukin (IL), interferon, the tumor necrosis factor superfamily, colony-stimulating factors, chemokines, and growth factors, play an important role in AD directly or indirectly by affecting smooth muscle cell function (17,31). For example, Zhang et al. reported that IL-1β may be involved in AD through the upregulation of MMP-9 and stromal cell apoptosis (32). Data from clinical trials and animal studies indicated that elevated IL-6 mRNA levels in the circulation and aorta of patients with AD (33-35) downregulated IL-6 expression and affected the phenotypic transition of the smooth muscle by inhibiting the vascular smooth muscle cell contraction proteins, α-smooth muscle actin, and SM22α (36).
CXCL1, which was highly expressed in an angiotensin II-induced murine AD model, promotes neutrophil infiltration and activation, upregulates local IL-6 expression, and leads to aortic dilation and rupture (37).
In subsequent construction of the PPI network, the top three genes were CDC20, CCNB2, and CCNB1, all of which are directly or indirectly involved in cell cycle regulation. Apoptosis and proliferation are closely related. Cell cycle regulators can affect cell division and programmed cell death (38). We confirmed the difference in correlation analysis between the disease and normal group through immunohistochemical verification of animal models, but more basic studies are needed to verify the specific mechanism of action.
To predict the potential for the effective treatment of AD, we used the Enrichr to identify therapeutic agents that might reverse the abnormally high expression of AD-related differential genes. Some of these drugs have shown promising results in basic research. For example, calcitriol (1,25-dihydroxyvitamin D3) is the active form of vitamin D3, a fat-soluble vitamin that promotes calcium absorption (39). In apoE-/- mice, oral calcitriol reduced vascular wall macrophage infiltration and neovascularization; significantly decreased MMP-2, MMP-9, and chemokine expression; and reduced the incidence of interlayer abdominal aortic aneurysm independent of systolic blood pressure or plasma cholesterol concentration (40). Martorell et al. reported that tamibarotene can reduce the damage response pathway and inflammatory pathway activation after inflammatory stimulation in smooth muscle cells by inhibiting the transcription factor KLF5 (40). Trocomatostatin enhances vascular repair in human endothelial progenitor cells and stem cells by increasing TAL1-dependent gene expression (41). However, the role of these drugs or molecular compounds in AD requires further exploration.
Our study had the following limitations. First, the sample size of the animal model was small. In the RNA extraction process, we found that the RNA concentration of a normal sample was low and was thus discarded. To reduce batch effects, we did not add a new control sample. Second, in the hub gene verification process, due to the difficulty acquiring human AD samples, especially normal aortic samples, we chose to use tissue samples from a murine model, which limited the study. Moreover, we do not know the specific mechanism of action of the screened hub genes. We will further explore the specific mechanism of action of these molecules using gene editing technology.
Conclusions
This study revealed transcriptional changes in a murine BAPN-induced AD model versus human AD transcriptome. Furthermore, we identified the important hub genes, related pathways, and potential drugs by analyzing the overlapping DEGs between human AD and the murine AD model. Our results provide a basis for the further identification of potential molecular markers for diagnosing and treating AD.
Acknowledgments
Funding: This work was supported by the National Natural Science Foundation of China (No. 81873529).
Footnote
Reporting Checklist: The authors have completed the ARRIVE reporting checklist. Available at https://jtd.amegroups.com/article/view/10.21037/jtd-23-981/rc
Data Sharing Statement: Available at https://jtd.amegroups.com/article/view/10.21037/jtd-23-981/dss
Peer Review File: Available at https://jtd.amegroups.com/article/view/10.21037/jtd-23-981/prf
Conflicts of Interest: All authors have completed the ICMJE uniform disclosure form (available at https://jtd.amegroups.com/article/view/10.21037/jtd-23-981/coif). The authors have no conflicts of interest to declare.
Ethical Statement: The authors are accountable for all aspects of the work in ensuring that questions related to the accuracy or integrity of any part of the work are appropriately investigated and resolved. All experiments involving animal treatment were performed in accordance with the National Institutes of Health Guide for the Care and Use of Laboratory Animals, and the protocol was approved by the Ethics Committee of Union Hospital affiliated with Huazhong University of Science and Technology (No. 2636).
Open Access Statement: This is an Open Access article distributed in accordance with the Creative Commons Attribution-NonCommercial-NoDerivs 4.0 International License (CC BY-NC-ND 4.0), which permits the non-commercial replication and distribution of the article with the strict proviso that no changes or edits are made and the original work is properly cited (including links to both the formal publication through the relevant DOI and the license). See: https://creativecommons.org/licenses/by-nc-nd/4.0/.
References
- Golledge J, Eagle KA. Acute aortic dissection. Lancet 2008;372:55-66. [Crossref] [PubMed]
- Thrumurthy SG, Karthikesalingam A, Patterson BO, et al. The diagnosis and management of aortic dissection. BMJ 2011;344:d8290. [Crossref] [PubMed]
- Juraszek A, Czerny M, Rylski B. Update in aortic dissection. Trends Cardiovasc Med 2022;32:456-61. [Crossref] [PubMed]
- Czerny M, Rylski B. Acute type A aortic dissection reconsidered: it’s all about the location of the primary entry tear and the presence or absence of malperfusion. Eur Heart J 2021;43:53-5. [Crossref] [PubMed]
- Nienaber CA, Clough RE, Sakalihasan N, et al. Aortic dissection. Nat Rev Dis Primers 2016;2:16053. [Crossref] [PubMed]
- El-Hamamsy I, Yacoub MH. Cellular and molecular mechanisms of thoracic aortic aneurysms. Nat Rev Cardiol 2009;6:771-86. [Crossref] [PubMed]
- Boufi M, Claudel M, Dona B, et al. Endovascular creation and validation of acute in vivo animal model for type A aortic dissection. J Surg Res 2018;225:21-8. [Crossref] [PubMed]
- Zheng HQ, Rong JB, Ye FM, et al. Induction of thoracic aortic dissection: a mini-review of β-aminopropionitrile-related mouse models. J Zhejiang Univ Sci B 2020;21:603-10. [Crossref] [PubMed]
- Yang YY, Li LY, Jiao XL, et al. Intermittent Hypoxia Alleviates β-Aminopropionitrile Monofumarate Induced Thoracic Aortic Dissection in C57BL/6 Mice. Eur J Vasc Endovasc Surg 2020;59:1000-10. [Crossref] [PubMed]
- Yang K, Ren J, Li X, et al. Prevention of aortic dissection and aneurysm via an ALDH2-mediated switch in vascular smooth muscle cell phenotype. Eur Heart J 2020;41:2442-53. [Crossref] [PubMed]
- Pan L, Bai P, Weng X, et al. Legumain Is an Endogenous Modulator of Integrin αvβ3 Triggering Vascular Degeneration, Dissection, and Rupture. Circulation 2022;145:659-74. [Crossref] [PubMed]
- Kurihara T, Shimizu-Hirota R, Shimoda M, et al. Neutrophil-derived matrix metalloproteinase 9 triggers acute aortic dissection. Circulation 2012;126:3070-80. [Crossref] [PubMed]
- Chomczynski P, Sacchi N. Single-step method of RNA isolation by acid guanidinium thiocyanate-phenol-chloroform extraction. Anal Biochem 1987;162:156-9. [Crossref] [PubMed]
- Love MI, Huber W, Anders S. Moderated estimation of fold change and dispersion for RNA-seq data with DESeq2. Genome Biol 2014;15:550. [Crossref] [PubMed]
- Kuleshov MV, Jones MR, Rouillard AD, et al. Enrichr: a comprehensive gene set enrichment analysis web server 2016 update. Nucleic Acids Res 2016;44:W90-7. [Crossref] [PubMed]
- Yoo M, Shin J, Kim J, et al. DSigDB: drug signatures database for gene set analysis. Bioinformatics 2015;31:3069-71. [Crossref] [PubMed]
- Mahmud SMH, Chen W, Meng H, et al. Prediction of drug-target interaction based on protein features using undersampling and feature selection techniques with boosting. Anal Biochem 2020;589:113507. [Crossref] [PubMed]
- Al-Mustanjid M, Mahmud SMH, Royel MRI, et al. Detection of molecular signatures and pathways shared in inflammatory bowel disease and colorectal cancer: A bioinformatics and systems biology approach. Genomics 2020;112:3416-26. [Crossref] [PubMed]
- Prêtre R, Von Segesser LK. Aortic dissection. Lancet 1997;349:1461-4. [Crossref] [PubMed]
- Zeng T, Shi L, Ji Q, et al. Cytokines in aortic dissection. Clin Chim Acta 2018;486:177-82. [Crossref] [PubMed]
- Luo F, Zhou XL, Li JJ, et al. Inflammatory response is associated with aortic dissection. Ageing Res Rev 2009;8:31-5. [Crossref] [PubMed]
- Olsson C, Thelin S, Ståhle E, et al. Thoracic aortic aneurysm and dissection: increasing prevalence and improved outcomes reported in a nationwide population-based study of more than 14,000 cases from 1987 to 2002. Circulation 2006;114:2611-8. [Crossref] [PubMed]
- Parve S, Ziganshin BA, Elefteriades JA. Overview of the current knowledge on etiology, natural history and treatment of aortic dissection. J Cardiovasc Surg (Torino) 2017;58:238-51. [Crossref] [PubMed]
- Zhu Y, Lingala B, Baiocchi M, et al. Type A Aortic Dissection-Experience Over 5 Decades: JACC Historical Breakthroughs in Perspective. J Am Coll Cardiol 2020;76:1703-13. [Crossref] [PubMed]
- Silaschi M, Byrne J, Wendler O. Aortic dissection: medical, interventional and surgical management. Heart 2017;103:78-87. [Crossref] [PubMed]
- Takagi H, Ando T, Umemoto T, et al. Meta-Analysis of Seasonal Incidence of Aortic Dissection. Am J Cardiol 2017;120:700-7. [Crossref] [PubMed]
- Gawinecka J, Schönrath F, von Eckardstein A. Acute aortic dissection: pathogenesis, risk factors and diagnosis. Swiss Med Wkly 2017;147:w14489. [Crossref] [PubMed]
- Nienaber CA, Clough RE. Management of acute aortic dissection. Lancet 2015;385:800-11. [Crossref] [PubMed]
- Simpson CF, Taylor WJ. Effect of hydralazine on aortic rupture induced by B-aminopropionitrile in turkeys. Circulation 1982;65:704-8. [Crossref] [PubMed]
- Fan F, Zhou Q, Xu Z, et al. Osteopontin in the Pathogenesis of Aortic Dissection by the Enhancement of MMP Expressions. Int Heart J 2019;60:429-35. [Crossref] [PubMed]
- Wortmann M, Peters AS, Erhart P, et al. Inflammasomes in the Pathophysiology of Aortic Disease. Cells 2021;10:2433. [Crossref] [PubMed]
- Zhang L, Liao MF, Tian L, et al. Overexpression of interleukin-1β and interferon-γ in type I thoracic aortic dissections and ascending thoracic aortic aneurysms: possible correlation with matrix metalloproteinase-9 expression and apoptosis of aortic media cells. Eur J Cardiothorac Surg 2011;40:17-22. [Crossref] [PubMed]
- Sano M, Anzai J. The molecular mechanisms contributing to the pathophysiology of systemic inflammatory response after acute aortic dissection. Nihon Rinsho Meneki Gakkai Kaishi 2016;39:91-5. [Crossref] [PubMed]
- Brasier AR. The nuclear factor-kappaB-interleukin-6 signalling pathway mediating vascular inflammation. Cardiovasc Res 2010;86:211-8. [Crossref] [PubMed]
- del Porto F, Proietta M, Tritapepe L, et al. Inflammation and immune response in acute aortic dissection. Ann Med 2010;42:622-9. [Crossref] [PubMed]
- Xu Y, Ye J, Wang M, et al. Increased interleukin-11 levels in thoracic aorta and plasma from patients with acute thoracic aortic dissection. Clin Chim Acta 2018;481:193-9. [Crossref] [PubMed]
- Anzai A, Shimoda M, Endo J, et al. Adventitial CXCL1/G-CSF expression in response to acute aortic dissection triggers local neutrophil recruitment and activation leading to aortic rupture. Circ Res 2015;116:612-23. [Crossref] [PubMed]
- Vermeulen K, Berneman ZN, Van Bockstaele DR. Cell cycle and apoptosis. Cell Prolif 2003;36:165-75. [Crossref] [PubMed]
- Kassi E, Adamopoulos C, Basdra EK, et al. Role of vitamin D in atherosclerosis. Circulation 2013;128:2517-31. [Crossref] [PubMed]
- Martorell S, Hueso L, Gonzalez-Navarro H, et al. Vitamin D Receptor Activation Reduces Angiotensin-II-Induced Dissecting Abdominal Aortic Aneurysm in Apolipoprotein E-Knockout Mice. Arterioscler Thromb Vasc Biol 2016;36:1587-97. [Crossref] [PubMed]
- Palii CG, Vulesevic B, Fraineau S, et al. Trichostatin A enhances vascular repair by injected human endothelial progenitors through increasing the expression of TAL1-dependent genes. Cell Stem Cell 2014;14:644-57. [Crossref] [PubMed]