Early stroke after left ventricular assist device implantation: role of right heart failure
Highlight box
Key findings
• Right heart failure (RHF) after left ventricular assist device (LVAD) implantation was a risk factor for early post-LVAD stroke.
What is known and what is new?
• The incidence of stroke after LVAD implantation exhibits a bimodal reverse J-shaped curve, indicating both early and late post-LVAD stroke phases.
• Postoperative RHF might have significant impact on early stroke.
What is the implication, and what should change now?
• Since postoperative RHF usually accompanies low cerebral perfusion pressure (CPP) and can cause watershed infarct or lacunar infarct, it is suggested that maintaining mean CPP at least 60 mmHg or higher immediately after LVAD implantation could help reduce early stroke after LVAD implantation. This could be a new approach different from the current trend focusing on the risk of high mean blood pressure after LVAD implantation.
Introduction
Left ventricular assist devices (LVADs) are increasingly used to treat end-stage heart failure. LVADs extend survival and improved quality of life in heart failure patients (1). However, post-LVAD stroke is a frequent and devastating complication (2). The incidence of stroke after LVAD implantation exhibits a bimodal reverse J-shaped curve, with risk peaking immediately after implantation and increasing again 1 year later (3,4), indicating both early and late post-LVAD stroke phases.
Until now, maintaining adequate blood pressure (5,6) and anticoagulation targets (5,7) and preventing complications related to post-LVAD maintenance, including post-LVAD infection (8) and pump thrombosis (3), have been investigated as prognostic factors for post-LVAD stroke. However, few studies have analyzed early-phase and late-phase stroke separately (3,9,10). Postoperative right heart failure (RHF) is also a fatal complication after LVAD implantation (11). Patients with RHF typically have increased central venous pressure (CVP) and decreased mean arterial pressure (MAP). This physiology can reduce cerebral perfusion pressure (CPP) which causes cerebral hypoperfusion and congestion. Herein, we investigated the relation between early stroke and postoperative RHF. We present this article in accordance with the STROBE reporting checklist (available at https://jtd.amegroups.com/article/view/10.21037/jtd-23-1194/rc).
Methods
This was a single-center retrospective observational study. From January 2012 to December 2020, patients who underwent LVAD implantation in our medical center were eligible for study inclusion (Figure 1). We excluded patients with non-dischargeable LVAD insertion and one foreign patient lost to follow-up because she returned to her home country after implantation. The follow-up completion rate was 100%. The study was conducted in accordance with the Declaration of Helsinki (as revised in 2013). The Institutional Review Board of Samsung Medical Center approved this study (SMC 2021-09-016-001, date of approval: September 8, 2021). The Institutional Review Board waived informed consent from individual patients because this retrospective study had minimal risk for patients.
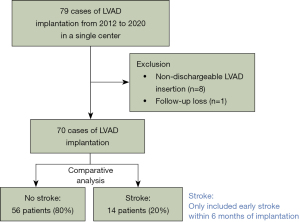
Study variables and definitions
Early stroke was defined as stroke within 6 months after implantation. Stroke was diagnosed using a standard neurological examination performed by a neurologist and documented with appropriate diagnostic imaging, such as computed tomography. Transient ischemic attacks were also included as stroke events. Patients who experienced strokes within 6 months of LVAD implantation were categorized into the stroke group; those who did not experience strokes in the first 6 months were categorized into the no-stroke group. The detailed definitions of post-LVAD complications including RHF, which are consistent with Interagency Registry for Mechanically Assisted Circulatory Support (INTERMACS) definitions, are provided in the Supplementary materials online (12). Frailty was assessed by a specialist in rehabilitation medicine and was defined as category 4 or higher according to the Spirduso classification (13).
Warfarin use was calculated as the percentage of overall time in therapeutic range (TTR) using the Rosendaal method (14). CPP was defined as the difference between MAP and CVP (15), which were measured simultaneously by invasive monitoring.
Patient management during and after LVAD implantation
Transesophageal monitoring was routinely used to exclude interatrial shunt in the operating room. After LVAD implantation, the target MAP was between 70 and 90 mmHg. In patients who underwent HeartWare Ventricular Assist Device System (HVAD) implantation, target MAP was <80 mmHg. If the patient did not have an arterial line, blood pressure was measured with an automated non-invasive cuff in patients; in patients who fail to check blood pressure from a non-invasive cuff, manual measurement with Doppler ultrasound assistance was used. For anticoagulation, an intravenous heparin infusion was initiated 12 hours after LVAD implantation, targeting an activated partial thromboplastin time of 45–55 seconds until the international normalized ratio (INR) reached 1.5 if no significant post-LVAD bleeding occurred. Warfarin, targeting an INR of 2.0–3.0, and an antiplatelet agent was initiated the day after implantation.
Statistical analysis
The detailed statistical analysis process is provided in the Supplementary materials online. Categorical variables are reported as frequencies with percentages; continuous variables are reported as means with standard deviations or medians with range. The cumulative incidence of RHF is estimated by a Fine-Gray model which accounts for the competing risk of death and heart transplantation. A Cox proportional hazard regression analysis was performed to identify predictors of early stroke at 6 months after implantation. Receiver operating characteristic (ROC) curve analysis for early stroke was performed, and the area under the curve (AUC) was calculated. To compare repetitive values of pump flow, mean blood pressure, INR, and lactate dehydrogenase (LDH) concentration according to stroke, we used a linear mixed model.
Results
Baseline characteristics
Among the 70 patients who were enrolled, 14 patients (20.0%) experienced a postoperative stroke during the 6 months after LVAD implantation (Table 1, Figure 2A). About 0.28 stroke events per patient-year occurred during follow-up. Mean age of the study population was 63.0±13.9 years and 55 patients (78.6%) were men. 24 patients (34.3%) received extracorporeal membrane oxygenation before LVAD implantation and 22 patients (31.4%) had history of cardiopulmonary resuscitation before LVAD implantation.
Table 1
Variables | No-stroke group (n=56) | Stroke group (n=14) | P value |
---|---|---|---|
Age (years) | 66.50 [56.50–73.25] | 64.00 [59.00–69.25] | 0.649 |
Male sex | 45 (80.4) | 10 (71.4) | 0.480 |
Body mass index (kg/m2) | 23.26±3.00 | 23.28±3.31 | 0.982 |
Preoperative comorbidity | |||
Frailty | 32 (57.1) | 13 (92.9) | 0.013 |
DM | 32 (57.1) | 9 (64.3) | 0.856 |
HTN | 26 (46.4) | 8 (57.1) | 0.676 |
Dialysis | 21 (37.5) | 6 (42.9) | 0.951 |
Previous stroke history | 10 (17.9) | 5 (35.7) | 0.275 |
Smoking history | 27 (48.2) | 8 (57.1) | 0.765 |
Solid organ cancer | 8 (14.3) | 1 (7.1) | 0.675 |
Liver dysfunction† | 18 (32.1) | 4 (28.6) | >0.99 |
Atrial fibrillation | 32 (57.1) | 8 (57.1) | >0.99 |
Cardiac surgery history | 7 (12.5) | 8 (57.1) | 0.001 |
CPR history | 18 (32.1) | 4 (28.6) | >0.99 |
ECMO status before LVAD implantation | 18 (32.1) | 6 (42.9) | 0.659 |
Ischemic etiology of heart failure | 26 (46.4) | 9 (64.3) | 0.370 |
Medical support status | 0.452 | ||
Bridge to transplantation | 29 (51.8) | 9 (64.3) | |
Bridge to candidacy | 3 (5.4) | 1 (7.1) | |
Destination therapy | 24 (42.9) | 4 (28.6) | |
Preoperative ECHO | |||
LVEF | 0.393 | ||
30–39% | 6 (10.7) | 3 (21.4) | |
20–29% | 30 (53.6) | 5 (35.7) | |
<20% | 20 (35.7) | 6 (42.9) | |
LVEDD (cm) | 6.87±1.10 | 6.88±0.96 | >0.99 |
TR | 0.739 | ||
None | 2 (3.6) | 1 (7.1) | |
Mild | 34 (60.7) | 9 (64.3) | |
Moderate | 14 (25.0) | 2 (14.3) | |
Severe | 8 (14.3) | 3 (21.4) | |
Mean PAP (mmHg) | 35.40±11.16 | 37.55±9.20 | 0.558 |
CVP | 11.0 [7.00–15.00] | 10.00 [8.50–18.00] | 0.670 |
Data are expressed as median [range], number (%), or mean ± standard deviation. †, liver dysfunction was defined as liver cirrhosis or congestive hepatomegaly. DM, diabetes mellitus; HTN, hypertension; CPR, cardiopulmonary resuscitation; ECMO, extracorporeal membrane oxygenation; LVAD, left ventricular assist device; ECHO, echocardiography; LVEF, left ventricular ejection fraction; LVEDD, left ventricular end-diastolic diameter; TR, tricuspid regurgitation; PAP, pulmonary arterial pressure; CVP, central venous pressure.
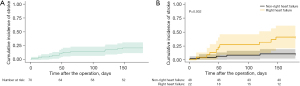
Patients with stroke were more likely to have preoperative frailty (92.9% vs. 57.1%, P=0.013) and cardiac surgery history (57.1% vs. 12.5%, P=0.001), compared to those without stroke. Specifically, a history of coronary artery bypass graft was more frequent in the stroke group compared with the frequency in the no-stroke group (42.9% vs. 5.4%, P=0.001) (Table S1).
Surgical and postoperative characteristics
During the study period, HeartMate II Left Ventricular Assist System (HMII), HVAD, and HeartMate 3 Left Ventricular Assist System (HM3) were implanted in 23 patients (32.9%), 46 patients (65.7%), and 1 patient (1.4%), respectively (Table 2). HVADs were more frequently implanted in the stroke group compared with the frequency in the no-stroke group (92.9% vs. 58.9%, P=0.042). Postoperative antiplatelet and heparin bridging were used in 56 patients (80.0%) and 57 patients (81.4%), respectively.
Table 2
Variables | No-stroke group (n=56) | Stroke group (n=14) | P value |
---|---|---|---|
Surgical characteristics | |||
Device type | 0.042 | ||
HMII | 22 (39.3) | 1 (7.1) | |
HM3 | 1 (1.8) | 0 (0.0) | |
HVAD | 33 (58.9) | 13 (92.9) | |
Intracardiac thrombus removal | 7 (12.5) | 1 (7.1) | >0.99 |
Concomitant valve surgery | 15 (26.8) | 1 (7.1) | 0.164 |
Postoperative characteristics | |||
Antiplatelet use | 47 (83.9) | 9 (64.3) | 0.204 |
Heparin bridging | 44 (78.6) | 13 (92.9) | 0.441 |
TTR using Rosendaal method <40% | 34 (60.7) | 7 (50.0) | 0.564 |
Pump flow within 7 days of implantation (L/min) | 4.11±0.98 | 3.98±0.64 | 0.621 |
Mean MAP within 3 days of implantation (mmHg) | 74.32±7.36 | 71.05±6.79 | 0.136 |
Mean CVP within 3 days of implantation (mmHg) | 10.26±2.38 | 11.04±2.35 | 0.279 |
Mean CPP within 3 days of implantation (mmHg) | 63.99±8.24 | 60.45±8.11 | 0.154 |
Mean CPP at POD 0 (mmHg) | 63.06±10.10 | 57.50±7.38 | 0.089 |
Mean CPP at POD 1 (mmHg) | 63.43±7.79 | 58.06±8.28 | 0.026 |
Mean CPP at POD 2 (mmHg) | 63.51±9.68 | 58.06±8.28 | 0.689 |
Mean CPP at POD 3 (mmHg) | 65.46±8.45 | 61.46±10.69 | 0.191 |
Data are expressed as number (%) or mean ± standard deviation. HMII, HeartMate II Left Ventricular Assist System; HM3, HeartMate 3 Left Ventricular Assist System; HVAD, HeartWare Ventricular Assist Device System; TTR, time in therapeutic range; MAP, mean arterial pressure; CVP, central venous pressure; CPP, cerebral perfusion pressure; POD, postoperative day.
The frequencies of postoperative atrial fibrillation, postoperative antiplatelet use, heparin bridging to warfarin, and TTR <40% were similar between the groups. Mean CPP at postoperative day (POD) 1 (58.06 vs. 63.43 mmHg, P=0.026; Table 2, Figure S1) was lower in the stroke group compared with these parameters in the no-stroke group. Pump flow during the 7 days within implantation, MAP within 3 days of implantation, and CVP within 3 days of implantation were similar between the groups (Table 2, Figures S1,S2).
Postoperative outcomes and stroke
Sixty-seven patients (95.7%) were successfully discharged and 16 patients (22.9%) died during follow-up (Table 3). No significant differences in postoperative ventilator support, inotropic support, atrial fibrillation, and infection were detected between the two groups. However, postoperative RHF was more common in the stroke group (64.3% vs. 23.2%, P=0.008) and the median time from implantation to RHF was 1 day.
Table 3
Variables | No-stroke group (n=56) | Stroke group (n=14) | P value |
---|---|---|---|
Heart transplantation | 24 (42.9) | 5 (35.7) | 0.856 |
Survival to discharge | 55 (98.2) | 12 (85.7) | 0.858 |
ICU stay (days) | 9.91±9.65 | 15.14±13.34 | 0.099 |
Hospital stay (days) | 54.50±58.91 | 85.21±87.40 | 0.231 |
Postoperative inotropic support (days) | 8.12±10.39 | 8.29±4.45 | 0.930 |
Postoperative ventilator support (days) | 4.02±6.51 | 4.86±4.62 | 0.652 |
Postoperative CRRT support (days) | 3.00±6.49 | 6.64±8.76 | 0.085 |
Postoperative atrial fibrillation | 11 (19.6) | 5 (35.7) | 0.355 |
Postoperative infection | 15 (26.8) | 2 (14.3) | 0.531 |
Postoperative pump thrombosis | 0 (0.0) | 0 (0.0) | NA |
Heparin induced thrombocytopenia | 0 (0.0) | 0 (0.0) | NA |
Postoperative RHF | 13 (23.2) | 9 (64.3) | 0.008 |
Implantation to RHF (days) | 1 [1–2] | 1 [0.5–3.5] | 0.948 |
CPC score at discharge | 0.303 | ||
1 | 54 (96.4) | 12 (85.7) | |
2 | 1 (1.8) | 1 (7.1) | |
4 | 1 (1.8) | 1 (7.1) | |
Stroke | – | ||
Ischemic stroke | – | 5 (35.7) | |
Hemorrhagic stroke | – | 8 (57.1) | |
Hemorrhagic transformation of ischemic stroke | – | 1 (7.1) | |
Traumatic | – | 1 (7.1) | |
PRES | – | 1 (7.1) | |
Modified Rankin scale at stroke event | – | 2 [0–6] | |
LVAD implantation to stroke (days) | – | 47 [1–147] |
Data are expressed as number (%), mean ± SD, or median [range]. ICU, intensive care unit; CRRT, continuous renal replacement therapy; NA, not available; RHF, right heart failure; CPC, cerebral performance category; PRES, posterior reversible encephalopathy syndrome;LVAD, left ventricular assist device.
Among the 14 patients (20.0%), who experienced a postoperative stroke during the 6 months after LVAD implantation, five patients had ischemic strokes, one patient had posterior reversible encephalopathy syndrome, and eight patients had hemorrhagic strokes. Among the eight cases of hemorrhagic stroke, one was suspected to be a hemorrhagic transformation of an ischemic stroke, and another was due to trauma-induced stroke. The median modified Rankin scale at the stroke event was 2, and the median time from implantation to stroke was 47 days (range, 1–147 days). There were no statistical differences in the rates of all-cause death between patients with stroke and those without stroke (Figure S3).
Risk factors for early stroke
Patients with postoperative RHF had a significantly higher 6-month stroke rate than patients without postoperative RHF (44.6% vs. 10.5%, P=0.002; Figure 2B). In the multivariable analyses including RHF as a variable (Table S2, Table 4, Figure 3), preoperative frailty [hazard ratio (HR): 7.703; 95% confidence interval (CI): 1.001–59.279; P=0.049], preoperative cardiac surgery history (HR: 3.065; 95% CI: 1.059–8.869; P=0.039), and postoperative RHF (HR: 5.063; 95% CI: 1.682–15.245; P=0.004) were significant independent predictors of early stroke. In the multivariable analyses including CPP at POD 1 as a variable (Table S3, Figure S4), preoperative cardiac surgery history (HR: 3.916; 95% CI: 1.323–11.594; P=0.014), postoperative antiplatelet use (HR: 0.286; 95% CI: 0.093–0.881; P=0.029), and CPP at POD 1 (HR: 0.923; 95% CI: 0.858–0.992; P=0.030) were independent risk factors for early stroke. In the ROC curve and cutoff analyses using the Youden index (Figure S5), the AUC for CPP was 0.688. The CPP cutoff value for predicting an early stroke event was 62 mmHg (sensitivity, 71.4%; specificity, 59.3%).
Table 4
Variables | Multivariable analysis | ||
---|---|---|---|
HR | 95% CI | P value | |
Preoperative | |||
Frailty | 7.703 | 1.001–59.279 | 0.049 |
Cardiac surgery history | 3.065 | 1.059–8.869 | 0.039 |
Postoperative | |||
RHF | 5.063 | 1.682–15.245 | 0.004 |
HR, hazard ratio; CI, confidence interval; RHF, right heart failure.
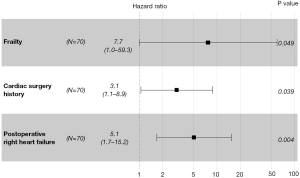
Patients with postoperative RHF
Patients in the RHF group were younger compared to patients in the no RHF group (56.68 vs. 65.94 years, P=0.009), but comorbidities and echocardiographic characteristics did not differ between the groups (Table S4). MAP was lower (69.20 vs. 73.97 mmHg, P<0.001), CVP was higher (11.82 vs. 9.85 mmHg, P<0.001), and mean CPP was lower (57.38 vs. 64.13 mmHg, P<0.001) within 3 days of implantation (Figure 4, Table S5) in the RHF group compared with these measurements in the no RHF group.
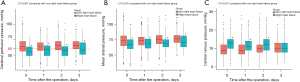
Patients who presented RHF after surgery received various forms of support during the early stage, including multiple inotropes (n=22, 100.0%; median duration of support, 7 days), inhaled nitric oxide (n=9, 40.9%; median duration of support, 2 days), continuous renal replacement therapy (n=15, 68.2%; median duration of support, 7.5 days), or right ventricular assist device support (n=4, 18.2%; median duration of support, 8.5 days; Table S6). Upon discharge, they continued to receive sildenafil (n=15, 68.2%), digoxin (n=8, 36.4%), and high-dose diuretics (n=4, 18.2%). After 6 months following LVAD surgery, 10 patients (45.5%), 3 patients (13.6%), and 4 patients (18.2%) were still on sildenafil, digoxin, and high-dose diuretics, respectively.
Discussion
Two main findings from this study are important: (I) postoperative RHF is an independent risk factor for early stroke after LVAD implantation, and (II) low CPP, which occurred in patients with post-LVAD RHF, is an independent risk factor for early stroke in LVAD-implanted patients. Postoperative stroke is a frequent and devastating complication after LVAD implantation, and many studies focused on correctable and non-correctable risk factors for postoperative stroke. One notable investigation into the risk factors for neurologic events suggested that anticoagulation, antiplatelet therapy, and blood pressure management affect the risk of cerebrovascular accidents after HVAD implantation (5,6). Other studies suggested that postoperative infection (8), low pump flow (3), anticoagulation (16), and etiology of heart failure (7) were risk factors for stroke. However, very few studies focused on risk factors for early stroke, except one study suggesting that low cardiac flow was a risk factor for early stroke (3) and a report showing a bimodal curve for the hazard function of stroke over time (4).
In this study, postoperative RHF was an independent risk factor for early stroke after LVAD implantation. Postoperative RHF is a common and harmful complication after LVAD implantation (11) and RHF usually occurs in the first 2 weeks (17). Heart failure is a well-known risk factor for stroke in the general population (18-20). In a population-based cohort study, the risk of stroke was strongly increased shortly after the diagnosis of heart failure but returned to normal within 6 months after the onset of heart failure (18), which was the period for early stroke in our study. RHF in LVAD-implanted patients may increase the risk of stroke similar to heart failure in the general population.
Two possible mechanisms might account for the association between RHF and stroke in LVAD-implanted patients: thromboembolism and hypoperfusion (20). Coagulopathy and stasis of blood flow in akinetic ventricular segments and dilated atrium in RHF might increase thrombus formation (20). This mechanism also explains the effects of preoperative cardiac surgery history on stroke development in this study; thrombogenicity is increased by artificial valve materials and akinetic ventricular segments after coronary artery bypass grafting surgery. However, we did not detect any differences in the anticoagulation profiles (i.e., postoperative antiplatelet use, heparin bridging, TTR, etc.) or postoperative LDH levels indicative of thrombosis (21,22) between the stroke and the non-stroke groups.
Regarding hypoperfusion, the CPP and cerebrovascular resistance regulate cerebral blood flow (15). Although cerebral autoregulation maintains cerebral blood flow at a relatively constant level over a wide range of CPP (50 to 100 mmHg) (15,23), autoregulation of cerebrovascular resistance might be dysfunctional in certain circumstances [i.e., chronic hypertension (16,24), cardiac arrest (23), chronic heart failure (24), continuous flow LVAD (25)] that are frequently observed in this study population, and minor changes of CPP could injure the brain (19,20). During cerebral hypoperfusion in patients with post-LVAD RHF, deep brain structures that are supplied by the deep penetrating arteries and lack collateral flow or structures located at the junction supplied by both the middle and anterior cerebral arteries may be vulnerable to ischemic damage (19,20). Furthermore, in a state where autoregulation is compromised, if CVP increases due to RHF, this can potentially lead to hemorrhagic strokes caused by venous or capillary rupture and could also serve as a cause for posterior reversible encephalopathy syndrome.
Interestingly, our results suggest that low perfusion pressure is a warning sign for the development of early stroke. In our study cohort, CPP was significantly lower in patients with post-LVAD RHF compared with CPP in patients without RHF on the first 3 PODs. The low cerebral perfusion on POD 1 was an independent risk factor for developing stroke, with a cutoff value of 62 mmHg. Previous studies focused on high MAP as a risk factor for developing stroke in LVAD-implanted patients (5,6). However, this study suggests that, during the early phase after LVAD implantation, when cerebral autoregulation might be dysfunctional or right-shifted (26), low CPP might increase the risk of stroke and should be avoided. We recommend that CPP should not be lower than 60mmHg in the immediate postoperative period after LVAD implantation, which is consistent in other recommendations in post-cardiac arrest care or in traumatic brain injury (27). Target CPP in the early postoperative period of LVAD implantation need to be investigated in future large-scale studies.
Several limitations of this study should be mentioned. Firstly, this is a retrospective single-center study with a small number of subjects, and selection bias could exist. Secondly, one limitation of this study is the inclusion of various LVAD devices, such as HMII, HM3, and HVAD. Although HVAD is no longer in use due to poor hemocompatibility, a significant number of patients still receive HVAD support. Therefore, insights from the older devices remain relevant. Furthermore, in this study, the influence of device type was accounted for in the Cox multivariable analysis, and since the focus is on the association between RHF and stroke, there are sufficient implications for the HM3 currently in use also. Future investigations should explore the association between stroke and RHF specifically in the context of the commonly used HM3 device. Thirdly, there are inherent limitations in establishing a causal relationship between RHF and stroke. It is challenging to determine the prolonged effect of RHF on post-LVAD hemodynamics (Table S6), making it difficult to distinguish the extent to which RHF contributes to individual stroke events. However, this study serves as an initial exploration of the link between RHF and stroke, and its significance lies in paving the way for further comprehensive investigations. In-depth research that thoroughly examines the impact of RHF and associated parameters on post-LVAD stroke is crucial for elucidating a causal relationship in the future. Lastly, ischemic and hemorrhagic stroke were not analyzed separately. Nevertheless, in fact, hemorrhagic stroke and ischemic stroke are known to share risk factors including infection, female sex, hypertension, and antiplatelet use. In addition, in the real world, it is hard to differentiate hemorrhagic stroke from hemorrhagic conversion of ischemic stroke. Future large-scale studies might be needed to differentiate the effects of RHF and CPP on the two types of strokes.
Conclusions
Post-LVAD RHF is a risk factor for early stroke after LVAD implantation, especially in those with dysfunctional cerebral autoregulation and low CPP. Prevention of RHF by appropriate patient selection and management of RHF with adequate CPP may reduce the incidence of post-LVAD stroke.
Acknowledgments
Funding: This work was supported by
Footnote
Reporting Checklist: The authors have completed the STROBE reporting checklist. Available at https://jtd.amegroups.com/article/view/10.21037/jtd-23-1194/rc
Data Sharing Statement: Available at https://jtd.amegroups.com/article/view/10.21037/jtd-23-1194/dss
Peer Review File: Available at https://jtd.amegroups.com/article/view/10.21037/jtd-23-1194/prf
Conflicts of Interest: All authors have completed the ICMJE uniform disclosure form (available at https://jtd.amegroups.com/article/view/10.21037/jtd-23-1194/coif). Y.H.C. reports that this work was supported by the Korea Medical Device Development Fund grant funded by the Korea government (the Ministry of Science and ICT, the Ministry of Trade, Industry and Energy, the Ministry of Health & Welfare, the Ministry of Food and Drug Safety) (Project Number: RS-2020-KD000159). The other authors have no conflicts of interest to declare.
Ethical Statement: The authors are accountable for all aspects of the work in ensuring that questions related to the accuracy or integrity of any part of the work are appropriately investigated and resolved. The study was conducted in accordance with the Declaration of Helsinki (as revised in 2013). The Institutional Review Board of Samsung Medical Center approved this study (SMC 2021-09-016-001, date of approval: September 8, 2021). The Institutional Review Board waived informed consent from individual patients because this retrospective study had minimal risk for patients.
Open Access Statement: This is an Open Access article distributed in accordance with the Creative Commons Attribution-NonCommercial-NoDerivs 4.0 International License (CC BY-NC-ND 4.0), which permits the non-commercial replication and distribution of the article with the strict proviso that no changes or edits are made and the original work is properly cited (including links to both the formal publication through the relevant DOI and the license). See: https://creativecommons.org/licenses/by-nc-nd/4.0/.
References
- Slaughter MS, Rogers JG, Milano CA, et al. Advanced heart failure treated with continuous-flow left ventricular assist device. N Engl J Med 2009;361:2241-51. [Crossref] [PubMed]
- Acharya D, Loyaga-Rendon R, Morgan CJ, et al. INTERMACS Analysis of Stroke During Support With Continuous-Flow Left Ventricular Assist Devices: Risk Factors and Outcomes. JACC Heart Fail 2017;5:703-11. [Crossref] [PubMed]
- Samura T, Yoshioka D, Toda K, et al. Risk of stroke early after implantation of a left ventricular assist device. J Thorac Cardiovasc Surg 2019;157:259-267.e1. [Crossref] [PubMed]
- Frontera JA, Starling R, Cho SM, et al. Risk factors, mortality, and timing of ischemic and hemorrhagic stroke with left ventricular assist devices. J Heart Lung Transplant 2017;36:673-83. [Crossref] [PubMed]
- Teuteberg JJ, Slaughter MS, Rogers JG, et al. The HVAD Left Ventricular Assist Device: Risk Factors for Neurological Events and Risk Mitigation Strategies. JACC Heart Fail 2015;3:818-28. [Crossref] [PubMed]
- Milano CA, Rogers JG, Tatooles AJ, et al. HVAD: The ENDURANCE Supplemental Trial. JACC Heart Fail 2018;6:792-802. [Crossref] [PubMed]
- Boyle AJ, Jorde UP, Sun B, et al. Pre-operative risk factors of bleeding and stroke during left ventricular assist device support: an analysis of more than 900 HeartMate II outpatients. J Am Coll Cardiol 2014;63:880-8. [Crossref] [PubMed]
- Cho SM, Moazami N, Katz S, et al. Stroke Risk Following Infection in Patients with Continuous-Flow Left Ventricular Assist Device. Neurocrit Care 2019;31:72-80. [Crossref] [PubMed]
- Yoshioka D, Sakaniwa R, Toda K, et al. Relationship Between Bacteremia and Hemorrhagic Stroke in Patients With Continuous-Flow Left Ventricular Assist Device. Circ J 2018;82:448-56. [Crossref] [PubMed]
- Yoshioka D, Okazaki S, Toda K, et al. Prevalence of Cerebral Microbleeds in Patients With Continuous-Flow Left Ventricular Assist Devices. J Am Heart Assoc 2017;6:e005955. [Crossref] [PubMed]
- LaRue SJ, Raymer DS, Pierce BR, et al. Clinical outcomes associated with INTERMACS-defined right heart failure after left ventricular assist device implantation. J Heart Lung Transplant 2017;36:475-7. [Crossref] [PubMed]
- Interagency Registry for Mechanically Assisted Circulatory Support. Appendix D - Adverse Event Definitions. Accessed December 13, 2023. Available online: http://classic.clinicaltrials.gov/ProvidedDocs/42/NCT02268942/Prot_000.pdf
- Freiberger E, Häberle L, Spirduso WW, et al. Long-term effects of three multicomponent exercise interventions on physical performance and fall-related psychological outcomes in community-dwelling older adults: a randomized controlled trial. J Am Geriatr Soc 2012;60:437-46. [Crossref] [PubMed]
- Rosendaal FR, Cannegieter SC, van der Meer FJ, et al. A method to determine the optimal intensity of oral anticoagulant therapy. Thromb Haemost 1993;69:236-9.
- Markus HS. Cerebral perfusion and stroke. J Neurol Neurosurg Psychiatry 2004;75:353-61. [Crossref] [PubMed]
- Harvey L, Holley C, Roy SS, et al. Stroke After Left Ventricular Assist Device Implantation: Outcomes in the Continuous-Flow Era. Ann Thorac Surg 2015;100:535-41. [Crossref] [PubMed]
- Kormos RL, Teuteberg JJ, Pagani FD, et al. Right ventricular failure in patients with the HeartMate II continuous-flow left ventricular assist device: incidence, risk factors, and effect on outcomes. J Thorac Cardiovasc Surg 2010;139:1316-24. [Crossref] [PubMed]
- Alberts VP, Bos MJ, Koudstaal P, et al. Heart failure and the risk of stroke: the Rotterdam Study. Eur J Epidemiol 2010;25:807-12. [Crossref] [PubMed]
- Havakuk O, King KS, Grazette L, et al. Heart Failure-Induced Brain Injury. J Am Coll Cardiol 2017;69:1609-16. [Crossref] [PubMed]
- Kim W, Kim EJ. Heart Failure as a Risk Factor for Stroke. J Stroke 2018;20:33-45. [Crossref] [PubMed]
- Cowger JA, Romano MA, Shah P, et al. Hemolysis: a harbinger of adverse outcome after left ventricular assist device implant. J Heart Lung Transplant 2014;33:35-43. [Crossref] [PubMed]
- Uriel N, Morrison KA, Garan AR, et al. Development of a novel echocardiography ramp test for speed optimization and diagnosis of device thrombosis in continuous-flow left ventricular assist devices: the Columbia ramp study. J Am Coll Cardiol 2012;60:1764-75. [Crossref] [PubMed]
- van den Brule JMD, van der Hoeven JG, Hoedemaekers CWE. Cerebral Perfusion and Cerebral Autoregulation after Cardiac Arrest. Biomed Res Int 2018;2018:4143636. [Crossref] [PubMed]
- Lepic T, Loncar G, Bozic B, et al. Cerebral blood flow in the chronic heart failure patients. Perspect Med 2012;1:304-8.
- Bhimaraj A, Uribe C, Suarez EE. Physiological impact of continuous flow on end-organ function: clinical implications in the current era of left ventricular assist devices. Methodist Debakey Cardiovasc J 2015;11:12-7. [Crossref] [PubMed]
- Sundgreen C, Larsen FS, Herzog TM, et al. Autoregulation of cerebral blood flow in patients resuscitated from cardiac arrest. Stroke 2001;32:128-32. [Crossref] [PubMed]
- Callaway CW, Donnino MW, Fink EL, et al. Part 8: Post-Cardiac Arrest Care: 2015 American Heart Association Guidelines Update for Cardiopulmonary Resuscitation and Emergency Cardiovascular Care. Circulation 2015;132:S465-82. [Crossref] [PubMed]