Knockdown of Slfn5 alleviates lipopolysaccharide-induced pneumonia by regulating Janus kinase/signal transduction and activator of transcription pathway
Highlight box
Key findings
• Slfn5 expression was increased in lipopolysaccharide (LPS)-induced pneumonia model. Knockdown of Slfn5 mitigated LPS‑induced mice lung injury and inflammation. Overexpression of Slfn5 enhanced apoptosis and inflammatory cytokines in LPS-treated A549 cells. Knockdown of Slfn5 weakened lung injury, apoptosis, and inflammatory response through the inhibition of JAK/STAT pathway.
What is known and what is new?
• Abnormal Slfn5 expression affects the progression of various cancers, including lung cancer.
• Here, in vivo and in vitro, we found that knockdown of Slfn5 inhibited cell apoptosis and the production of inflammatory cytokines in LPS-induced pneumonia model. Additionally, Slfn5 had a regulatory effect on the JAK/STAT pathway.
What is the implication, and what should change now?
• Our study provides new insights for the pathogenesis of pneumonia. Slfn5 may be a promising therapeutic target for pneumonia treatment.
Introduction
The incidence of pneumonia, a respiratory disease, has increased in recent years (1). Based on the investigation of disease burden, about 14% of children worldwide die of lower respiratory infections (2). Clinically, pneumonia mainly manifests as an elevated body temperature, dyspnoea, hypoxaemia, chills, chest pain, coughing, and other severe comorbidities (3-5). Various pathogens (such as bacteria, fungi, and viruses) can cause pneumonia (6,7). Currently, the primary diagnostic methods for pneumonia are through radiological technology and with multiple laboratory methods (8). The use of oxygen, assisted ventilation, antibiotics, and other supportive therapies is the primary treatment strategy for pneumonia (9,10). Owing to the complexity of the pathogenesis of pneumonia, therapeutic effects are not satisfactory. Therefore, exploring the pathogenesis and effective therapeutic targets is of paramount importance for improving the prognosis of pneumonia.
The family of schlafen (Slfn) genes is originally identified in mice, they are preferentially expressed in lymphoid tissue (11). During thymocyte maturation and T cell activation, the genes of Slfn family are differentially regulated. Moreover, in fibroblasts and thymoma cells, cell growth is inhibited by the expression of Slfn1. Recent studies have indicated that Slfn family members participate in primary cellular processes, such as proliferation, viral replication, differentiation, immune response, and growth regulation (12-14). Slfn5 is expressed in normal melanocytes, renal cells, and ovary cells (13). Abnormal Slfn5 expression affects the progression of renal cell carcinoma, gastric cancer, melanoma, and glioblastoma (13,15-17). However, the function of Slfn5 has not yet been completely understood in pneumonia.
JAK/STAT pathway is related to diverse biological processes, such as cell growth, immune response, apoptosis, and inflammatory response (18,19). Various growth factors, ligands, and cytokines can trigger the JAK pathway (20). The tyrosine of STAT can be phosphorylated by activated JAK, and the phosphorylated STAT transfers to the nucleus and binds to specific promotor, resulting in target mRNA expression (21). At present, an increasing amount of evidence has confirmed that JAK/STAT pathway is involved in lipopolysaccharide (LPS)-induced lung injury in mouse (22-24).
LPS is produced by gram-negative bacteria and is essential for inflammatory reactions related to pneumonia (25-27). TLR4 is a key receptor for LPS induction (28). The activated TLR4 can activate myeloid differentiation factor 88, extracellular signal-related kinases 1 and 2, JAK2, and interleukin-1 receptor-associated kinase 1, further promoting the nuclear translocation of nuclear factor kappa B and the phosphorylation of STAT3, consequently, upregulating the levels of inflammation-related factors, such as tumor necrosis factor (TNF) and interleukins (IL-6, IL-8, IL-1β) (29-33). Excessive accumulation of inflammatory cytokines can cause the dysfunction of immune system, thereby damage to multiple tissues, including lung tissue (34). Here, in vivo and in vitro, we first used LPS to establish a pneumonia model, and then investigated the role and underlying mechanism of Slfn5 in pneumonia. We present this article in accordance with the ARRIVE reporting checklist (available at https://jtd.amegroups.com/article/view/10.21037/jtd-23-889/rc).
Methods
Bioinformatics analysis
Slfn5 expression in rat bronchoalveolar lavage fluid (BALF) was analyzed through Gene Expression Omnibus database (GSE111241). Gene set enrichment analysis (GSEA) was used to analyze the pathways enriched by Slfn5.
Construction of LPS‑induced pneumonia mouse model
The experimental protocol of our study was performed in accordance with the Guide for the Care and Use of Laboratory Animals and was approved by the Jinan City People’s Hospital (No. 2022-0621). GemPharmatech Co., Ltd (Nanjing, China) provided C57BL/6 mice (10-week-old, 25–30 g). All mice had free access to food and water under a 12-h light-dark photocycle at constant temperature and humidity. Pentobarbital sodium (50 mg/kg; Sigma, St. Louis, MO, USA) was used to anaesthetise the mice. To construct pneumonia-infected lung injury, mice (LPS group, n=10) were intratracheally administered LPS (5 mg/kg) (Sigma). An equal volume of normal saline was administered to mice in the control group (n=10). One week before the construction of the pneumonia mouse model, in the LPS + sh-NC (n=10) and LPS + sh-Slfn5 group (n=10), mice were injected with adenoviral vectors containing short hairpin RNA-negative control (sh-NC, 1×109 pfu/100 µL) and shRNA of Slfn5 (sh-Slfn5, 1×109 pfu/100 µL) via the tail vein. After injection of LPS for three days, the mice were sacrificed and then the BALF and the lung tissues were harvested for later analysis.
Immunohistochemistry
The right lung of each mouse was fixed in 10% formalin. Next, the lung tissues were embedded in paraffin. After sectioning, the lung tissues were de-paraffinized with xylene and rehydrated with ethanol (75%, 80%, 90%, 95%, and 100%). To recover the antigen, lung tissue sections were incubated with Tris-EDTA buffer. Subsequently, sections were treated with 0.3% hydrogen peroxide and blocked with 10% goat serum. The sections were incubated with Slfn5 antibody and a horseradish peroxidase (HRP) -conjugated secondary antibody. After washing with phosphate-buffered saline (PBS), the sections were stained a DAB kit (Beyotime Biotechnology, Shanghai, China) and haematoxylin solution.
Lung wet-to-dry (W/D) ratio in mice
The wet weights of the left lungs were measured. After flushing with PBS, the lungs were incubated at 65 ℃ for 4 days in an oven to obtain dried specimens, and then the dry weight was measured. Finally, the W/D ratio of the mouse lungs was calculated.
Haematoxylin and eosin (H&E) staining
After dewaxing with xylene and treatment with ethanol, the lung tissue sections were stained with haematoxylin. The sections were then treated with 5% acetic acid and eosin. Next, the sections were dehydrated in ethanol (70%, 80%, 90%, and 100%), made transparent with xylene, and sealed with neutral gum. Pathological changes in the lung tissues were observed under a light microscope (Olympus, Tokyo, Japan).
TUNEL (terminal deoxynucleotidyl transferasemediated deoxyuridine triphosphate‑biotin nick end‑labelling) assay
After dewaxing with xylene and treatment with ethanol, sections of lung tissues were treated with 20 µg/mL proteinase K (Beyotime Biotechnology) for 20 min, and then washed with PBS. The TUNEL Apoptosis Detection Kit (Roche, Basel, Switzerland) was used to treat lung tissue sections. The sections were stained with diaminobenzidine and counterstained with haematoxylin. Finally, TUNEL-positive cells were observed under a light microscope. Brownish-yellow cells were considered apoptotic (TUNEL positive cells).
BALF collection and analysis
The mice lungs were washed three times with cooled PBS to collect the BALF. After centrifugation at 1,500 rpm for 10 min at 4 ℃, the cell pellets from BALF samples were resuspended in PBS. Total cells, neutrophils, and macrophages were counted using a haemocytometer and WrightGiemsa staining (Beyotime Biotechnology).
Cell culture and treatment
Procell Life Science & Technology Co., Ltd. (Wuhan, China) provided the A549 cell line. A549 cells were incubated in Dulbecco’s Modified Eagle Medium (DMEM) supplemented with 10% foetal bovine serum (FBS) and 1% penicillin/streptomycin (Invitrogen, Carlsbad, CA, USA) at 37 ℃ with 5% CO2.
To establish LPS-injured cell model, 10 µg/mL LPS was used to treat cells for 12 h. The pcDNA-Slfn5 (200 ng) and sh-Slfn5 (2 µg) and their respective negative controls [pcDNA-NC (200 ng) and sh-NC (2 µg)] were transfected into the cells using Lipofectamine 2000 (Life Technologies, Frederick, MD, USA). After transfection for 72 h, the cells in the LPS + sh-NC, LPS + sh-Slfn5, LPS + pcDNA-NC, and LPS + pcDNA-Slfn5 groups were treated with LPS. Cells in the LPS + pcDNA-Slfn5 + AG490 group were treated with the JAK/STAT inhibitor (AG490) and LPS.
Analysis of western blot
Total protein was extracted from the tissues and cells using radioimmunoprecipitation assay lysis buffer (Beyotime Biotechnology). Next, proteins (30 µg) were separated using 10% SDS-PAGE and transferred to PVDF (polyvinylidene difluoride) membranes (Sangon Biotech, Shanghai, China). Membranes were blocked with 5% skimmed milk for 1 h, and then membranes were probed with primary antibodies (Slfn5, TNF-α, IL-1β, IL-6, p-JAK2, JAK2, p-JAK3, JAK3, p-STAT3, and STAT3) at 4 ℃ overnight. Subsequently, the membranes were probed with secondary antibodies. Finally, an enhanced chemiluminescence kit (Beyotime Biotechnology) was used to visualise the protein bands.
Detection of cell viability
Cell viability was detected using a MTT [3-(4,5-dimethyl-2-thiazolyl)-2,5-diphenyl-2-h-tetrazolium bromide] assay. After indicated treatment, A549 cells in each well were treated with 20 µL MTT solution (Sigma) for 3 h. Next, cells were treated with 150 µL dimethyl sulfoxide (DMSO) for 15 min. Finally, the optical density was measured at 490 nm using a microplate reader (Bio-Rad, Hercules, CA, USA) and then cell viability was calculated.
Evaluation of cell apoptosis
Apoptosis was evaluated using the Annexin V-FITC Apoptosis Kit (Beyotime Biotechnology). Briefly, after the indicated treatments, A549 cells were resuspended in 1 × binding buffer. Subsequently, the cells were incubated with Annexin V-FITC and PI. Apoptotic cells were analyzed using flow cytometry (BD Biosciences, San Jose, CA, USA).
Enzyme-linked immunosorbent assay (ELISA)
After the indicated treatment, the levels of IL-6, IL-1β, and TNF-α were measured in A549 cell culture supernatant using ELISA kits (Beyotime Biotechnology) following the manufacturer’s instructions. Absorbance was measured at 450 nm using a microplate reader.
Statistical analysis
Data from three independent experiments were presented as the mean ± standard deviation (SD). Statistical analyses were performed using GraphPad Prism 7 and SPSS software. Student’s t-test and one-way analysis of variance (ANOVA) with Tukey’s test were used to compare significance. P value <0.05 indicated statistical significance.
Results
Slfn5 was increased in LPS-induced pneumonia in mice
GSE111241 database analyzes the abnormal expression genes in BALF of control (rats with normoxia) and LPS group (rats with LPS treatment with normoxia). An increase in Slfn5 expression was observed in LPS-treated rats (Figure 1A, P=0.0043). Next, we established a mouse model of pneumonia by treating with LPS. As shown in Figure 1B,1C, Slfn5 expression in the lung tissues was enhanced compared to that in the control group.
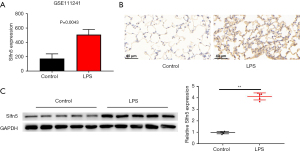
LPS‑induced mouse lung injury and inflammation were mitigated by knockdown of Slfn5
To further explore the regulatory role of Slfn5 in pneumonia, sh-Slfn5 and sh-NC were used to treat the mouse pneumonia model. As shown in Figure 2A, sh-Slfn5 decreased Slfn5 expression in the mouse lung tissues compared to that in the LPS + sh-NC group. Compared to the control group, LPS stimulation increased the lung W/D ratio, whereas knockdown of Slfn5 eliminated this increase (Figure 2B). H&E staining showed that LPS led to inflammatory cell infiltration, alveolar haemorrhage, and structural damage to the lung tissue, but knockdown of Slfn5 alleviated these LPS-induced changes (Figure 2C). TUNEL staining revealed a higher apoptosis ratio in LPS-treated mouse lung tissues; however, the apoptosis ratio was reduced by Slfn5 knockdown (Figure 2D). Next, we collected BALF and analyzed the number of total cells, macrophages, and neutrophils. LPS increased the number of total cells, neutrophils, and macrophages, whereas Slfn5 knockdown inhibited aggregation (Figure 2E-2G). Moreover, in LPS-treated lung tissues, knockdown of Slfn5 reduced the production of TNF-α, IL-1β, and IL-6 (Figure 2H).
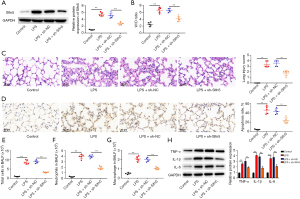
Knockdown of Slfn5 inhibited the JAK/STAT pathway in LPS-induced pneumonia in mice
To further explore the mechanism of Slfn5 in pneumonia, we performed GSEA and found that Slfn5 activated the JAK/STAT pathway (Figure 3A). We then assessed the changes in JAK/STAT pathway-related proteins. As shown in Figure 3B, LPS increased p-JAK2, p-JAK3, and p-STAT3 expression compared with the control group (P<0.01), whereas knockdown of Slfn5 decreased p-JAK2, p-JAK3, and p-STAT3 expression compared with the LPS + sh-NC group (P<0.01).
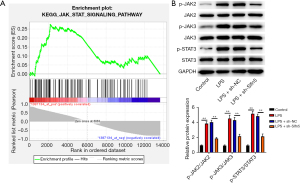
Overexpression of Slfn5 enhanced apoptosis and inflammatory factors in LPS-treated A549 cells
A549 cell is a human alveolar epithelial type II cell line. For various studies associated with respiratory diseases including pneumonia, A549 cells treated with LPS have been used as a cellular model (35-37). To investigate the role of Slfn5 in LPS-induced injury, sh-Slfn5 was transfected into A549 cells to construct low-expressed cells (Figure 4A). The MTT assay results showed that LPS reduced cell viability, whereas downregulation of Slfn5 expression increased cell viability in LPS-treated A549 cells (Figure 4B). In addition, the results of flow cytometry and ELISA showed an increased cell apoptosis and the levels of TNF-α, IL-1β, and IL-6 in LPS-treated cells, whereas downregulation of Slfn5 expression decreased cell apoptosis and the levels of TNF-α, IL-1β, and IL-6 compared to those in the LPS + sh-NC group (Figure 4C-4F). What’s more, pcDNA-Slfn5 was transfected into A549 cells to construct over-expressed cells (Figure 4G). As shown in Figure 4H-4L, compared to the LPS + pcDNA-NC group, overexpression of Slfn5 decreased cell viability and increased cell apoptosis and the levels of TNF-α, IL-1β, and IL-6.
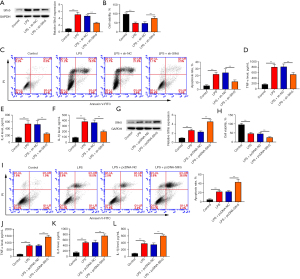
Knockdown of Slfn5 inhibited the JAK/STAT pathway in LPS-treated A549 cells
Changes in JAK/STAT pathway-related proteins were examined in LPS-treated A549 cells. The expression levels of p-JAK2, p-JAK3, and p-STAT3 were enhanced in LPS-stimulated cells (Figure 5A,5B). Compared with the LPS group, Slfn5 downregulation reduced p-JAK2, p-JAK3, and p-STAT3 expression (Figure 5A), whereas Slfn5 overexpression further enhanced p-JAK2, p-JAK3, and p-STAT3 expression (Figure 5B).
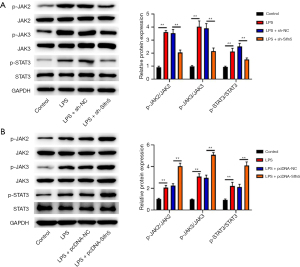
Slfn5 regulated apoptosis and inflammatory damage through the JAK/STAT pathway in LPS-treated A549 cells
To further verify whether Slfn5 regulates apoptosis and inflammatory damage through the JAK/STAT pathway, AG490 (an inhibitor of the JAK/STAT pathway) was used to treat LPS-stimulated A549 cells. As expected, compared to the LPS + pcDNA-Slfn5 group, AG490 inhibited p-JAK2, p-JAK3, and p-STAT3 expression in LPS-stimulated A549 cells (Figure 6A). Moreover, in LPS-stimulated A549 cells, AG490 mitigated the influences of Slfn5 overexpression on cell viability, apoptosis, and the production of TNF-α, IL-1β, and IL-6 (Figure 6B-6F).
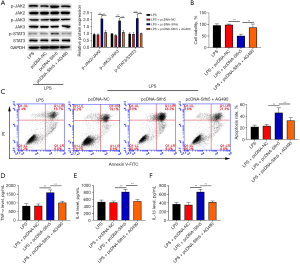
Discussion
As one of the lower respiratory tract illnesses, pneumonia is a common fatal infection in hospital (7,38). The pathogenesis of pneumonia is complex, and it is often accompanied by an immune response and inflammation, thus seriously damaging the function of the lung tissues (39). Severe pneumonia weakens the immune system, eventually leading to damage to the respiratory system, multiple organ failure, and even shock (40). Slfn5 was reported to be upregulated in non/low-invasive cancer cell lines (MCF7, HCT116, and A549 cells) compared with highly invasive cancer cell lines (HT1080 cells and 786-0 cells), and knockdown of Slfn5 increased the migration and invasion of MCF7, HCT116, and A549 cells (41). A previous study reported that Slfn5 expression was lower in lung adenocarcinoma (LUAC) tissues, and that overexpression of Slfn5 inhibited LUAC cell proliferation and promoted LUAC cell apoptosis (42). Here, in a LPS-induced pneumonia model, we found that Slfn5 expression was upregulated. Thus, we speculate that Slfn5 is involved in the development of pneumonia.
The most direct consequence of pneumonia is lung injury, characterized by the production of inflammatory cytokines and inflammatory damage to the alveolar capillary membrane (43,44). Animal experiments have shown that acute inflammatory reactions can be stimulated by LPS, with early histopathological changes observed in the lung tissues (45,46). Here, we injected LPS into mice to induce pulmonary inflammation and found that Slfn5 knockdown eliminated the influence of LPS on the lung W/D ratio, histopathological changes, apoptosis, and the number of total cells, macrophages, and neutrophils. Cytokine accumulation affects pneumonia progression. The release of TNF-α was reported to promote cell apoptosis and tissue necrosis (47). As a pro-inflammatory cytokine, IL-1β increases the permeability of pulmonary epithelium and neutrophils accumulation, causing lung oedema (48). In the plasma of patients with pneumonia, IL-6 levels are increased; high IL-6 levels in circulating leukocytes increase incidence of secondary infection in patients with pneumonia (49). In our study, in LPS-treated lung tissues, knockdown of Slfn5 reduced TNF-α, IL-1β, and IL-6 levels. Moreover, in vitro, downregulation of Slfn5 increased cell viability and decreased cell apoptosis and TNF-α, IL-1β, and IL-6 levels, whereas overexpression of Slfn5 led to the opposite results. Taken together, Slfn5 knockdown mediated the improvement of LPS-induced pneumonia.
One of the classic inflammation-related pathways, the JAK/STAT pathway participates in cytokine-mediated biological response (50). A previous study has reported that JAKs affects cytokine receptors and are involved in intracellular responses (51). STAT3 activation plays an important role in many oncogenic signaling pathways and promotes inflammatory cytokine production (52). Zhu-Ge et al. found that LPS exposure activated the JAK/STAT pathway, and knockdown of CRNDE alleviated inflammatory injury and reduced the activity of the JAK/STAT pathway (53). Moreover, knockdown of lncRNA SNHG16 attenuated LPS-induced WI-38 cell injury, which was related to the inhibition of the JNK pathways (54). In the present study, in vivo and in vitro, we observed that LPS increased p-JAK2, p-JAK3, and p-STAT3 expression, whereas Slfn5 knockdown decreased p-JAK2, p-JAK3, and p-STAT3 expression. Moreover, in LPS-stimulated A549 cells, AG490, an inhibitor of the JAK/STAT pathway, mitigated the influence of Slfn5 overexpression on cell viability, apoptosis, and the production of TNF-α, IL-1β, and IL-6. These results illustrate that Slfn5 regulates apoptosis and inflammatory damage via the JAK/STAT pathway in the LPS-induced pneumonia model. Although A549 cells are widely used for in vitro pneumonia research, a human normal non-cancerous lung cell line should be used to validate the results of this study.
Conclusions
Collectively, our results indicated that Slfn5 expression was upregulated in the LPS-induced pneumonia model. Knockdown of Slfn5 reduced lung injury, apoptosis, and inflammatory response, which might be related to the inhibition of the JAK/STAT pathway. Our study identified a promising therapeutic target for the treatment of pneumonia.
Acknowledgments
Funding: None.
Footnote
Reporting Checklist: The authors have completed the ARRIVE reporting checklist. Available at https://jtd.amegroups.com/article/view/10.21037/jtd-23-889/rc
Data Sharing Statement: Available at https://jtd.amegroups.com/article/view/10.21037/jtd-23-889/dss
Peer Review File: Available at https://jtd.amegroups.com/article/view/10.21037/jtd-23-889/prf
Conflicts of Interest: All authors have completed the ICMJE uniform disclosure form (available at https://jtd.amegroups.com/article/view/10.21037/jtd-23-889/coif). The authors have no conflicts of interest to declare.
Ethical Statement:
Open Access Statement: This is an Open Access article distributed in accordance with the Creative Commons Attribution-NonCommercial-NoDerivs 4.0 International License (CC BY-NC-ND 4.0), which permits the non-commercial replication and distribution of the article with the strict proviso that no changes or edits are made and the original work is properly cited (including links to both the formal publication through the relevant DOI and the license). See: https://creativecommons.org/licenses/by-nc-nd/4.0/.
References
- Bunthi C, Baggett HC, Gregory CJ, et al. Enhanced surveillance for severe pneumonia, Thailand 2010-2015. BMC Public Health 2019;19:472. [Crossref] [PubMed]
- Global Burden of Disease Pediatrics Collaboration. Global and National Burden of Diseases and Injuries Among Children and Adolescents Between 1990 and 2013: Findings From the Global Burden of Disease 2013 Study. JAMA Pediatr 2016;170:267-87. [Crossref] [PubMed]
- Mattila JT, Fine MJ, Limper AH, et al. Pneumonia. Treatment and diagnosis. Ann Am Thorac Soc 2014;11:S189-92. [Crossref] [PubMed]
- Watkins RR, Lemonovich TL. Diagnosis and management of community-acquired pneumonia in adults. Am Fam Physician 2011;83:1299-306.
- Dean P, Florin TA. Factors Associated With Pneumonia Severity in Children: A Systematic Review. J Pediatric Infect Dis Soc 2018;7:323-34. [Crossref] [PubMed]
- Cillóniz C, Torres A, Niederman M, et al. Community-acquired pneumonia related to intracellular pathogens. Intensive Care Med 2016;42:1374-86. [Crossref] [PubMed]
- Mandell LA. Community-acquired pneumonia: An overview. Postgrad Med 2015;127:607-15. [Crossref] [PubMed]
- Zar HJ, Andronikou S, Nicol MP. Advances in the diagnosis of pneumonia in children. BMJ 2017;358:j2739. [Crossref] [PubMed]
- Lassi ZS, Kumar R, Das JK, et al. Antibiotic therapy versus no antibiotic therapy for children aged two to 59 months with WHO-defined non-severe pneumonia and wheeze. Cochrane Database Syst Rev 2014;CD009576. [Crossref] [PubMed]
- Kolek V. Community pneumonia - fundamentals of diagnosing and treatment. Vnitr Lek 2017;63:514-7.
- Schwarz DA, Katayama CD, Hedrick SM. Schlafen, a new family of growth regulatory genes that affect thymocyte development. Immunity 1998;9:657-68. [Crossref] [PubMed]
- Puck A, Aigner R, Modak M, et al. Expression and regulation of Schlafen (SLFN) family members in primary human monocytes, monocyte-derived dendritic cells and T cells. Results Immunol 2015;5:23-32. [Crossref] [PubMed]
- Katsoulidis E, Mavrommatis E, Woodard J, et al. Role of interferon {alpha} (IFN{alpha})-inducible Schlafen-5 in regulation of anchorage-independent growth and invasion of malignant melanoma cells. J Biol Chem 2010;285:40333-41. [Crossref] [PubMed]
- de la Casa-Esperón E. From mammals to viruses: the Schlafen genes in developmental, proliferative and immune processes. Biomol Concepts 2011;2:159-69. [Crossref] [PubMed]
- Sassano A, Mavrommatis E, Arslan AD, et al. Human Schlafen 5 (SLFN5) Is a Regulator of Motility and Invasiveness of Renal Cell Carcinoma Cells. Mol Cell Biol 2015;35:2684-98. [Crossref] [PubMed]
- Companioni Nápoles O, Tsao AC, Sanz-Anquela JM, et al. SCHLAFEN 5 expression correlates with intestinal metaplasia that progresses to gastric cancer. J Gastroenterol 2017;52:39-49. [Crossref] [PubMed]
- Arslan AD, Sassano A, Saleiro D, et al. Human SLFN5 is a transcriptional co-repressor of STAT1-mediated interferon responses and promotes the malignant phenotype in glioblastoma. Oncogene 2017;36:6006-19. [Crossref] [PubMed]
- Stevens LE, Peluffo G, Qiu X, et al. JAK-STAT Signaling in Inflammatory Breast Cancer Enables Chemotherapy-Resistant Cell States. Cancer Res 2023;83:264-84. [Crossref] [PubMed]
- Hu Q, Bian Q, Rong D, et al. JAK/STAT pathway: Extracellular signals, diseases, immunity, and therapeutic regimens. Front Bioeng Biotechnol 2023;11:1110765. [Crossref] [PubMed]
- Bousoik E, Montazeri Aliabadi H. "Do We Know Jack" About JAK? A Closer Look at JAK/STAT Signaling Pathway. Front Oncol 2018;8:287. [Crossref] [PubMed]
- Qing Y, Stark GR. Alternative activation of STAT1 and STAT3 in response to interferon-gamma. J Biol Chem 2004;279:41679-85. [Crossref] [PubMed]
- Xu R, Shao Z, Cao Q. MicroRNA-144-3p enhances LPS induced septic acute lung injury in mice through downregulating Caveolin-2. Immunol Lett 2021;231:18-25. [Crossref] [PubMed]
- Chen L, Yu T, Zhai Y, et al. Luteolin Enhances Transepithelial Sodium Transport in the Lung Alveolar Model: Integrating Network Pharmacology and Mechanism Study. Int J Mol Sci 2023;24:10122. [Crossref] [PubMed]
- Qi S, Feng Z, Li Q, et al. Myricitrin Modulates NADPH Oxidase-Dependent ROS Production to Inhibit Endotoxin-Mediated Inflammation by Blocking the JAK/STAT1 and NOX2/p47(phox) Pathways. Oxid Med Cell Longev 2017;2017:9738745. [Crossref] [PubMed]
- Zhang X, Wu D, Tian Y, et al. Ganoderma lucidum polysaccharides ameliorate lipopolysaccharide-induced acute pneumonia via inhibiting NRP1-mediated inflammation. Pharm Biol 2022;60:2201-9. [Crossref] [PubMed]
- Oliveira J, Reygaert WC. Gram-Negative Bacteria. 2023.
- Li L, Xu W, Luo Y, et al. Aloe polymeric acemannan inhibits the cytokine storm in mouse pneumonia models by modulating macrophage metabolism. Carbohydr Polym 2022;297:120032. [Crossref] [PubMed]
- Liu X, Lin S, Zhong Y, et al. Remimazolam Protects Against LPS-Induced Endotoxicity Improving Survival of Endotoxemia Mice. Front Pharmacol 2021;12:739603. [Crossref] [PubMed]
- Oh H, Park SH, Kang MK, et al. Asaronic Acid Attenuates Macrophage Activation toward M1 Phenotype through Inhibition of NF-κB Pathway and JAK-STAT Signaling in Glucose-Loaded Murine Macrophages. J Agric Food Chem 2019;67:10069-78. [Crossref] [PubMed]
- Somensi N, Rabelo TK, Guimarães AG, et al. Carvacrol suppresses LPS-induced pro-inflammatory activation in RAW 264.7 macrophages through ERK1/2 and NF-kB pathway. Int Immunopharmacol 2019;75:105743. [Crossref] [PubMed]
- Park JG, Son YJ, Yoo BC, et al. Syk Plays a Critical Role in the Expression and Activation of IRAK1 in LPS-Treated Macrophages. Mediators Inflamm 2017;2017:1506248. [Crossref] [PubMed]
- Ju M, Liu B, He H, et al. MicroRNA-27a alleviates LPS-induced acute lung injury in mice via inhibiting inflammation and apoptosis through modulating TLR4/MyD88/NF-κB pathway. Cell Cycle 2018;17:2001-18. [Crossref] [PubMed]
- Wang Y, Wang X, Li Y, et al. Xuanfei Baidu Decoction reduces acute lung injury by regulating infiltration of neutrophils and macrophages via PD-1/IL17A pathway. Pharmacol Res 2022;176:106083. [Crossref] [PubMed]
- Schulte W, Bernhagen J, Bucala R. Cytokines in sepsis: potent immunoregulators and potential therapeutic targets--an updated view. Mediators Inflamm 2013;2013:165974. [Crossref] [PubMed]
- Bian XX, Zhao X, Ma CH, et al. Arbutin Alleviates LPS Induced Sepsis Pneumonia in Mice. Evid Based Complement Alternat Med 2022;2022:5863952. [Crossref] [PubMed]
- Liu Q, Yang H, Xu S, et al. Downregulation of p300 alleviates LPS-induced inflammatory injuries through regulation of RhoA/ROCK/NF-κB pathways in A549 cells. Biomed Pharmacother 2018;97:369-74. [Crossref] [PubMed]
- Wang QL, Yang L, Peng Y, et al. Ginsenoside Rg1 Regulates SIRT1 to Ameliorate Sepsis-Induced Lung Inflammation and Injury via Inhibiting Endoplasmic Reticulum Stress and Inflammation. Mediators Inflamm 2019;2019:6453296. [Crossref] [PubMed]
- Moldoveanu B, Otmishi P, Jani P, et al. Inflammatory mechanisms in the lung. J Inflamm Res 2009;2:1-11.
- Weight CM, Venturini C, Pojar S, et al. Microinvasion by Streptococcus pneumoniae induces epithelial innate immunity during colonisation at the human mucosal surface. Nat Commun 2019;10:3060. [Crossref] [PubMed]
- Luo W, Liu Y, Zhang Q, et al. Effect of traditional Chinese medicine injections on severe pneumonia: A protocol for systematic review and meta-analysis. Medicine (Baltimore) 2020;99:e22012. [Crossref] [PubMed]
- Wan G, Liu Y, Zhu J, et al. SLFN5 suppresses cancer cell migration and invasion by inhibiting MT1-MMP expression via AKT/GSK-3β/β-catenin pathway. Cell Signal 2019;59:1-12. [Crossref] [PubMed]
- Gu X, Zhou L, Chen L, et al. Human Schlafen 5 Inhibits Proliferation and Promotes Apoptosis in Lung Adenocarcinoma via the PTEN/PI3K/AKT/mTOR Pathway. Biomed Res Int 2021;2021:6628682. [Crossref] [PubMed]
- Gorski SA, Hufford MM, Braciale TJ. Recent insights into pulmonary repair following virus-induced inflammation of the respiratory tract. Curr Opin Virol 2012;2:233-41. [Crossref] [PubMed]
- Hernandez-Pando R, Orozco H, Arriaga K, et al. Analysis of the local kinetics and localization of interleukin-1 alpha, tumour necrosis factor-alpha and transforming growth factor-beta, during the course of experimental pulmonary tuberculosis. Immunology 1997;90:607-17. [Crossref] [PubMed]
- Lai K, Song C, Gao M, et al. Uridine Alleviates Sepsis-Induced Acute Lung Injury by Inhibiting Ferroptosis of Macrophage. Int J Mol Sci 2023;24:5093. [Crossref] [PubMed]
- Interdonato L, D’amico R, Cordaro M, et al. Aerosol-Administered Adelmidrol Attenuates Lung Inflammation in a Murine Model of Acute Lung Injury. Biomolecules 2022;12:1308. [Crossref] [PubMed]
- Redente EF, Keith RC, Janssen W, et al. Tumor necrosis factor-α accelerates the resolution of established pulmonary fibrosis in mice by targeting profibrotic lung macrophages. Am J Respir Cell Mol Biol 2014;50:825-37. [Crossref] [PubMed]
- Zhao G, Zhang T, Ma X, et al. Oridonin attenuates the release of pro-inflammatory cytokines in lipopolysaccharide-induced RAW264.7 cells and acute lung injury. Oncotarget 2017;8:68153-64. [Crossref] [PubMed]
- Chen C, Shi L, Li Y, et al. Disease-specific dynamic biomarkers selected by integrating inflammatory mediators with clinical informatics in ARDS patients with severe pneumonia. Cell Biol Toxicol 2016;32:169-84. [Crossref] [PubMed]
- Li SX, Yan W, Liu JP, et al. Long noncoding RNA SNHG4 remits lipopolysaccharide-engendered inflammatory lung damage by inhibiting METTL3 - Mediated m(6)A level of STAT2 mRNA. Mol Immunol 2021;139:10-22. [Crossref] [PubMed]
- Ghoreschi K, Laurence A, O’Shea JJ. Janus kinases in immune cell signaling. Immunol Rev 2009;228:273-87. [Crossref] [PubMed]
- Taub R. Hepatoprotection via the IL-6/Stat3 pathway. J Clin Invest 2003;112:978-80. [Crossref] [PubMed]
- Zhu-Ge D, Yang YP, Jiang ZJ. Knockdown CRNDE alleviates LPS-induced inflammation injury via FOXM1 in WI-38 cells. Biomed Pharmacother 2018;103:1678-87. [Crossref] [PubMed]
- Zhou Z, Zhu Y, Gao G, et al. Long noncoding RNA SNHG16 targets miR-146a-5p/CCL5 to regulate LPS-induced WI-38 cell apoptosis and inflammation in acute pneumonia. Life Sci 2019;228:189-97. [Crossref] [PubMed]