A better method to evaluate the reliability of echocardiography for assessment of pulmonary hypertension: comparison of tricuspid regurgitant spectrum quality grading and tricuspid valve regurgitation degree
Highlight box
Key findings
• For the first time, we graded the quality of tricuspid regurgitation (TR) spectrum based on its integrity and clarity, and the ultrasound measurements of pulmonary arterial systolic pressure (US-PASP) were trustworthy when tricuspid regurgitant spectrum quality grade (TR-SQG) was III or IV, even if TR was mild.
What is known and what is new?
• US-PASP was evaluated by sonographer based on TRS, and the information provided to the clinician was only the degree of regurgitation, not the quality of the spectrum.
• We demonstrated that the TR-SQG system was better than TR degree in evaluating the accuracy and reliability of US-PASP.
What is the implication, and what should change now?
• We can use the TR-SQG system to identify whose US-PASP is accurate, and to make reasonable treatment decisions; of course, assessing pulmonary arterial pressure with inaccurate US-PASP by other means is also necessary before making treatment decisions.
Introduction
Pulmonary hypertension (PH) is a progressive disease of the pulmonary vasculature characterized by increased vascular resistance and right ventricular (RV) pressure overload that can lead to RV failure and is associated with an increased mortality without treatment (1,2). The prevalence of PH is estimated at 1% in the global population and up to 10% of the 600 million people over the age of 65 years (3).
Early detection and accurate assessment are critical for improving the outcomes of PH patients. In the 2022, the European Society of Cardiology (ESC) and the European Respiratory Society (ERS) jointly released the 2022 Guidelines for the Diagnosis and Treatment of Pulmonary Hypertension, PH was defined by a pulmonary arterial mean pressure (PAMP) >20 mmHg at rest as confirmed by right heart catheterization (RHC) (4,5). This new definition undoubtedly unmasked a huge number of patients who previously fell in the range of elevated pulmonary arterial pressure (PAP) but were unable to meet the diagnostic requirements (6). Although direct measurement of PAP by RHC remains the reference method and the gold standard for diagnosis, its invasiveness limits its general applicability (7). According to current guidelines and consensus statements, transthoracic echocardiography (TTE) represents a widely available, feasible, and noninvasive method for screening, differential diagnosis, follow-up assessment, and risk stratification in PH (8,9).
The traditional echocardiographic approach for estimating pulmonary arterial systolic pressure (PASP) uses a derivation of RV systolic pressure from the tricuspid regurgitation velocity (TRV) added to a qualitative assessment of right atrial (RA) pressure (RAP). Using this method, previous studies have demonstrated an adequate correlation between this estimate of PASP and that obtained from invasive measurement but only moderate or insufficient precision of absolute PASP measurements from peak TRV (10-16). This is essential because substantial under- and over-estimation can occur in an individual patient, leading to misdiagnosis and improper therapy (17). This error is in part explained by inaccuracies in RAP estimation and poor Doppler imaging of the trans-tricuspid regurgitant jet (18). Especially for the latter, the volume of tricuspid regurgitation (TR), the operator’s measurement technique [e.g., taking multiple views to obtain the optimal window, ensuring the maximal continuous-wave (CW) Doppler velocity to flow angle is correctly aligned (19) and avoiding eccentric jets (6), over-gaining and artifacts (20), etc.], and the patient’s own conditions [e.g., age, sex, body mass index (BMI), breathing, heart rate, cardiopulmonary and metabolic comorbidities, and the ability to change position during examination (1,21-24), etc.] all influence the sharpness of the spectral image.
In daily practice, sonographers usually utilize the volume of regurgitant flow to determine the accuracy of TR measurements since, empirically, the larger the regurgitant volume, the clearer the image and the more reliable the measurement, and vice versa. Is a lower TR, however, an indication that the ultrasound measurement is inaccurate and imprecise? No. Even though the regurgitant flow is mild, we can still obtain reliable results if the spectrum is clear. Thus, the integrity and clarity of the TR spectrum (TRS), rather than the volume of regurgitant flow, should be used to determine the accuracy and precision of ultrasound measurements of PASP (US-PASP).
To date, no prospective literature exists to propose whether the quality of the TRS acts as a significant criterion for assessing the TR accuracy and precision. As such, this study was the first attempt to analyze the relationship between the quality of the TRS and the reliability of US-PASP. Here, we classified the quality of TR jet spectra into five grades, i.e., tricuspid regurgitant spectrum quality grade (TR-SQG) (Figure 1).
- TR-SQG 0: no TR or relevant spectrum;
- TR-SQG I: incomplete spectral wave envelope with less than 1/3 integrity and no peak;
- TR-SQG II: incomplete spectral wave envelope with an integrity of 1/3 to 2/3 and high signal dropout at peak velocity;
- TR-SQG III: incomplete spectral wave envelope with more than 2/3 integrity and a discernible peak;
- TR-SQG IV: high-intensity Doppler signal with a complete spectral wave envelope and a clearly visualized peak.
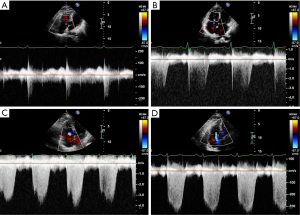
The aim of this study was to analyze the diagnostic reliability and clinical value of PH based on the quality of the TRS and to provide new insights into clinical practice for both sonographers and clinicians. We present this article in accordance with the STROBE reporting checklist (available at https://jtd.amegroups.com/article/view/10.21037/jtd-23-1084/rc).
Methods
This study was conducted on patients with chronic thromboembolic PH (CTEPH) who were assessed in the preoperative and postoperative period through comprehensive TTE and clinically indicated RHC to compare noninvasive hemodynamic estimates with invasively measured values. Echocardiography was first performed within a week before balloon pulmonary angioplasty (BPA), then PAP was measured each by RHC before and after BPA in CTEPH patients, and echocardiography was performed again within 24 hours after BPA. A noninvasive estimate of an estimated PASP of >35 mmHg or a TRV of >2.8 m/s is suspected of having PH. Direct measurement of PAMP >20 mmHg through invasive RHC remains the gold standard. The study was conducted in accordance with the Declaration of Helsinki (as revised in 2013). The study protocol was approved by the Ethics Committee at The First Affiliated Hospital of Guangzhou Medical University (Approval No. [2020]108). All patients provided written informed consent prior to participation in the current study.
Study population
There were 133 patients with CTEPH were enrolled, between November 2019 and March 2022, in The First Affiliation Hospital of Guangzhou Medical University, where a RHC and echocardiogram were performed. Exclusion criteria included: patients with absent or severe, free-flowing TR (the reflux tract was laminar flow), pulmonary artery stenosis or RV outflow track stenosis, no echocardiography examination.
TTE
According to current guidelines, all comprehensive TTE studies were performed using standard views and techniques, including routine measurements of tricuspid regurgitant flow, peak TRV, and regurgitant pressure difference. The severity of TR was determined based on color-flow Doppler imaging, using the parasternal RV inflow, parasternal short axis (PSAX), apical 4-chamber (A4C), and the subcostal views or a modified view between the PSAX and A4C (25,26). By comprehensively evaluating the regurgitation jet area, mild TR (TR ≤4.0 cm2) was defined as a small central jet, moderate TR (4.0 cm2 < TR ≤8.0 cm2) as an intermediate jet, and severe TR (TR >8.0 cm2) as a large jet. The spectrum image was obtained by CW Doppler across the tricuspid valve and was graded by the above self-defined criteria (Figure 1). The transvalvular pressure of TR (∆PTR) was estimated from the TR jet using the view showing maximal jet velocity and RAP was estimated by ultrasound assessment from the inferior vena cava diameter and inspiratory collapse. The US-PASP is equal to the sum between ∆PTR and ultrasound measurements of RAP (US-RAP), i.e., US-PASP = ∆PTR + US-RAP. All values presented were the average of at least three measurements conducted over consecutive cardiac cycles. Digitally recorded studies of all patients were reviewed and analyzed. Ultrasound images were performed by sonographers with extensive experience in echocardiography of 10 years.
BPA and RHC
BPA and RHC were performed by experienced investigators, blinded on echocardiographic features. All patients were placed supine in the center of the examination bed and given inhalation and suffocation training (inspiratory breath-hold). All procedures were carried out on conscious patients under local anesthesia. The intervention was performed via the right femoral venous access channel. BPA is a phased method in which a restricted number of lung segments are treated during each session. We treated 2 to 10 segmental or subsegmental arteries in each procedure session based on patient severity, procedure time (no more than 5 hours), and amount of contrast media provided (Visipaque®, 320 mg/mL, GE Healthcare, Chicago, IL, USA; the dosage does not exceed 100 mL/h). Heparin was used in place of warfarin for 3 days before to the surgery. During the process, the activated clotting time was set to be between 250 and 300 seconds. Warfarin was restarted following the procedure, and heparin infusion was prolonged until warfarin efficacy reached the optimum range (27). Applying the standardized protocols (28,29), RHC was performed using a Swan-Ganz catheter (Swan-Ganz CCOCEDV; Edwards Lifesciences, Irvine, CA, USA) before and immediately after the procedure. After suitable calibration with the zero-level set at the mid-thoracic line, pressure measurements were taken from the right atrium, right ventricle, and pulmonary artery at the end of expiration in baseline over at least three cardiac cycles to obtain an average of a minimum of three measurements, including PASP, PAMP, RA mean pressure (RAMP), pulmonary vascular resistance (PVR), systemic vascular resistance index, pulmonary/systemic vascular resistance ratio, and pulmonary and systemic vascular resistances. Cardiac output (CO) was determined with the Fick method, whereas the cardiac index (CI) was calculated by dividing the CO by the body surface area. Left-to-right shunting was ruled out by oximetry. All RHC measurements were repeated three times, and the mean value was calculated.
Statistical analysis
SPSS software version 26.0 was used for statistical analysis. Results were expressed as mean value ± standard deviation (SD). The correlation and consistency between US-PASP and RHC measurements of PASP (RHC-PASP) were tested by Pearson analysis, intraclass correlation coefficient (ICC) analysis, and Bland-Altman methods to derive bias, agreement, and confidence intervals. Paired t-test was conducted to compare the changes in hemodynamic parameters between US-PASP and RHC-PASP. P value <0.05 was considered statistically significant.
Results
Among these 133 patients, 7 patients with no TR and 18 patients with no echocardiography were excluded from the group. Therefore, data from a total of 108 patients were available for the final analysis. All patients were evaluated once each preoperatively and postoperatively for a total of 216 visits. Of these, 130 were mild TR, 40 were moderate TR, and 46 were severe TR. Patient characteristics recorded included age, gender, height, weight, invasive and echocardiographic data, as shown in Table 1. The analysis of the TRS quality’s composition ratio for TR severity showed that the larger the regurgitant volume, the higher the percentage of high-quality spectra (TR-SQG III and TR-SQG IV) obtained.
Table 1
Characteristics | Mild TR (n=130) | Moderate TR (n=40) | Severe TR (n=46) |
---|---|---|---|
Age (years) | 56.70±12.90 | 62.70±11.00 | 62.70±9.90 |
Male | 44 (33.85) | 12 (30.00) | 14 (30.43) |
Height (cm) | 167.40±7.90 | 161.70±9.00 | 163.10±8.50 |
Weight (kg) | 70.00±10.30 | 61.90±9.20 | 61.00±8.30 |
BMI (kg/m2) | 26.36±3.18 | 23.63±2.93 | 22.98±3.25 |
Hemodynamic data of RHC | |||
Mild PH/no PH | 84 (64.62) | 18 (45.00) | 5 (10.87) |
Moderate PH | 32 (24.62) | 14 (35.00) | 17 (36.96) |
Severe PH | 14 (10.77) | 8 (20.00) | 24 (52.17) |
CO (L/min) | 5.46±1.47 | 4.92±1.43 | 4.29±1.27 |
CI [L/(min·m2)] | 3.34±0.92 | 2.97±0.89 | 2.67±0.84 |
PVR (Wu) | 4.61±3.38 | 5.73±3.63 | 9.22±3.82 |
TPVR (Wu) | 6.26±3.88 | 7.89±4.12 | 11.36±3.88 |
RHC-RAMP (mmHg) | 6.08±2.78 | 6.13±4.31 | 7.67±4.68 |
RHC-PAMP (mmHg) | 30.84±11.96 | 34.45±11.60 | 45.46±9.87 |
RHC-PASP (mmHg) | 52.99±21.90 | 62.18±23.66 | 80.33±17.13 |
Echocardiography | |||
Area of TR (cm2) | 2.24±0.85 | 5.75±1.19 | 12.48±5.46 |
∆PTR (mmHg) | 41.64±18.64 | 59.63±21.11 | 80.46±16.33 |
US-PASP (mmHg) | 47.81±19.43 | 65.23±22.07 | 87.94±16.92 |
TR-SQG I | 26 (20.00) | 0 (0.00) | 0 (0.00) |
TR-SQG II | 42 (32.31) | 5 (12.50) | 0 (0.00) |
TR-SQG III | 43 (33.08) | 13 (32.50) | 6 (13.04) |
TR-SQG IV | 19 (14.62) | 22 (55.00) | 40 (86.96) |
Categorical variables presented as n (%); continuous variables presented as mean ± SD. TR, tricuspid regurgitation; BMI, body mass index; RHC, right heart catheterization; PH, pulmonary hypertension; CO, cardiac output; CI, cardiac index; PVR, pulmonary vascular resistance; TPVR, total pulmonary vascular resistance; RHC-RAMP, right heart catheterization measurements of right atrial mean pressure; RHC-PAMP, right heart catheterization measurements of pulmonary arterial mean pressure; RHC-PASP, right heart catheterization measurements of pulmonary arterial systolic pressure; ∆PTR, transvalvular pressure of tricuspid regurgitation; US-PASP, ultrasound measurements of pulmonary arterial systolic pressure; TR-SQG, tricuspid regurgitant spectrum quality grade; SD, standard deviation.
Consistency of US-PASP and RHC-PASP in severity TR
The ICC of US-PASP and RHC-PASP was 0.592 in mild TR, 0.750 in moderate TR, and 0.545 in severe TR, respectively, as shown in Table 2. The results of comparison between US-PASP and RHC-PASP based on TR severity are illustrated in Figure 2. Linear regression analysis showed positive correlation between US-PASP and RHC-PASP and moderate TR had the greatest correlation coefficient (r=0.754, P<0.001). However, it is not true that the larger the regurgitation volume, the closer US-PASP is to RHC-PASP. In moderate TR, US-PASP and RHC-PASP did not differ statistically (P=0.237), but they did in mild TR and severe TR (P<0.01). There was the underestimation or overestimation of PASP in mild TR or severe TR, respectively. Bland-Altman analysis revealed high bias between US-PASP and RHC-PASP with wide limits of agreements. The mean bias for US-PASP in moderate TR was 3.06 mmHg with 95% limits of agreement ranging from −28.53 to 34.65 mmHg. Thus, even after excluding the 5% of patients with the most discrepant values between US-PASP and RHC-PASP, TTE underestimated PASP as measured by RHC by as much as 28.53 mmHg and overestimated it by as much as 34.65 mmHg. The bias of the results obtained from Bland-Altman analysis was greater in both mild and severe TR.
Table 2
Different group of TR | US-PASP | RHC-PASP | ICC |
---|---|---|---|
TR severity | |||
Mild TR (n=130) | 47.81±19.43 | 52.99±21.90 | 0.592 |
Moderate TR (n=40) | 65.23±22.07 | 62.18±23.66 | 0.750 |
Severe TR (n=46) | 87.94±16.92 | 80.33±17.13 | 0.545 |
TR-SQG | |||
TR-SQG I (n=26) | 37.10±16.34 | 53.58±23.05 | 0.217 |
TR-SQG II (n=47) | 47.21±16.36 | 55.28±23.73 | 0.651 |
TR-SQG III (n=62) | 56.97±20.72 | 55.15±21.73 | 0.738 |
TR-SQG IV (n=81) | 75.98±24.79 | 69.89±23.19 | 0.793 |
TR-SQG III and TR-SQG IV | |||
Mild TR (n=62) | 53.42±20.89 | 50.98±19.50 | 0.774 |
Moderate TR (n=35) | 66.52±22.37 | 63.54±24.44 | 0.746 |
Severe TR (n=46) | 87.94±16.92 | 80.33±17.13 | 0.545 |
Continuous variables presented as mean ± SD. ICC, intraclass correlation coefficient; US-PASP, ultrasound measurements of pulmonary arterial systolic pressure; RHC-PASP, right heart catheterization measurements of pulmonary arterial systolic pressure; TR, tricuspid regurgitation; TR-SQG, tricuspid regurgitant spectrum quality grade; SD, standard deviation.
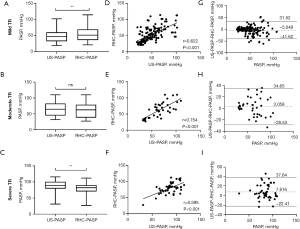
Consistency of US-PASP and RHC-PASP in TR-SQG
The ICC of US-PASP and RHC-PASP was 0.217, 0.651, 0.738, 0.793 in TR-SQG I–IV, respectively, as shown in Table 2. Using the self-defined grades of TRS to analyze US-PASP measurements (Figure 3), we found that there were strong correlations between US-PASP and RHC-PASP in grades II–IV spectrum, with r=0.747 (P<0.001), r=0.739 (P<0.001), and r=0.828 (P<0.001), respectively. However, US-PASP and RHC-PASP measurements were not statistically different in grades III (P=0.355), but were in TR-SQG II (P=0.001) and TR-SQG IV (P<0.001). Bland-Altman plots revealed the levels of agreement between the two were good for TR-SQG III but poor for others. The mean bias for US-PASP in TR-SQG III was 1.82 mmHg with 95% limits of agreement ranging from −28.30 to 31.94 mmHg, however the biases, for grades I, II, and IV, were −16.47, −8.07, and 6.09 mmHg, respectively, with wider 95% limits of agreement ranges.
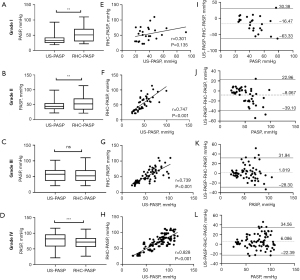
Which is a trustworthy US-PASP?
The higher the proportion of high-grade TR-SQ with the TR severity both before and after BPA is shown in Table 1. In theory, the higher of TR-SQG, the smaller the measurement error should be. Although the spectrum obtained from severe TR was TR-SQG III–IV, and TR-SQG IV accounted for 86.96%, there was no good consistency between US-PASP and RHC-PASP in severe TR (ICC was 0.545; r=0.595), which was lower than moderate TR (ICC was 0.750; r=0.754) and similar to mild TR (ICC was 0.592; r=0.622). In addition, TR-SQG III–IV had good consistency in US-PASP and RHC-PASP, and was significantly better than TR-SQG I–II. Even if TR was mild, its consistency was very good when the spectrums were TR-SQG III and IV between US-PASP and RHC-PASP (ICC was 0.774; r=0.779; Table 2), and it was not statistically different (P=0.160), the mean bias was 2.44 mmHg, and even similar to moderate TR with TR-SQG III and IV (ICC was 0.746; r=0.749; the mean bias was 2.98 mmHg).
Discussion
The closer the numbers measured by US-PASP and RHC-PASP are, the easier it is for US-PASP to gain trust and play a significant role in clinical decision-making.
Considering that RHC-PASP is an invasive examination and carries certain risks, the higher the PASP, the greater the risk, therefore, providing a highly reliable US-PASP data is of great help to physicians. The measurement results of US-PASP are influenced by various factors, but ultrasound doctors are more aware of which factors have the greatest impact on PASP results during the examination process, such as operator factors, reflux factors, spectral clarity, measurement factors, etc. How the impact of these influencing factors on the results is reflected in the ultrasound report and allows clinicians to clearly understand whether the measured results are accurate and credible is an important clinical problem to be solved.
In the present study, if there is no statistical difference in paired t-tests, the correlation coefficient is good, the Bland-Altman mean deviation is small, and ICC >0.70, it can be considered that the consistency between the two is good. We found that the reliability of high-quality spectra (grades III and IV) in assessing PH was better than that of TR severity, and TRS quality grading can help clinical recognition of the accuracy and precision of ultrasound assessment of PH and offer new insights for clinical practice. Therefore, providing the evaluation results of TR-SQG in ultrasound reporting can help to solve the aforementioned difficult problem.
Patients with mild TR in our study underestimated PASP and had large limits of agreement. Inadequate TR jet Doppler signals are the most common source of inappropriate US-PASP estimation. Some studies suggested that a low level of TR may lead to an incomplete Doppler spectrum and an underestimation of PASP (6,30). This is to be expected, as the Doppler method’s correctness is dependent on obtaining the right peak velocity, from which the peak pressure can be estimated. Likewise, our data showed that PASP were underestimated in grades I and II TR, with high biases and wide 95% levels of agreement ranges. Therefore, a large proportion of low spectra quality (TR-SQG I 20.00% and TR-SQG II 32.31%) in mild TR could explain the inaccurate assessment and the poor agreement. This problem can be alleviated in part by using contrast bubbles in the right side of the heart or injecting of intravenous agitated saline (31). In addition, a study by Jeon et al. (32) demonstrated that the correlations were further improved and the best limits of agreement were obtained between echocardiography and RHC measurements using a 10% air, 10% blood, and 80% saline mixture.
Although the proportion of higher-quality spectra increased with TR severity, in this study, higher-quality spectra and subsequent reliable PH measures can be obtained with small amounts of regurgitation, which may be related to the operator’s measurement technique and the patient’s own conditions (as mentioned before); conversely, large regurgitant volume such as severe TR can also produce high-quality spectrum, but the measurement results are not as good as moderate regurgitant volume such as moderate TR.
Obtaining a high-quality TRS is an important factor for a reliable PH assessment. This was supported by our findings that moderate TR with a considerable proportion of high-quality spectra (TR-SQG III and TR-SQG IV, 87.50%) did not differ statistically and had strong correlation and agreement. However, unlike moderate TR, severe TR, with 100% of high-quality spectra (TR-SQG III and TR-SQG IV, 100.00%), suffered from the same issue of high bias and large agreement limitations as mild TR. Patients with advanced PH or severe TR more often have a significant reduction in the systolic function of RV and hence low US-PASP, because RV is unable to generate the appropriate pressure, which translates into an increased pre-load and the development of peripheral features of RV failure. Although it is believed that extremely severe TR can hinder the normal RV-RA pressure gradient from being maintained and cause the TR Doppler envelope to be cut short (33,34), leading to PASP to be underestimated, our results did not support this conception. The PASP was overestimated even when the envelope quality was excellent, with the absolute degree of pressure overestimation being greatest at higher pressure (11). This is due to the fact that the TRV is squared and multiplied by 4 when estimating PASP from the TRV using the Bernoulli equation, so even tiny errors can result in huge changes. Even with the same TRV difference, say 0.1 m/s, the pressure overestimation develops as TRV increases. In the study data, the proportion of severe PH in severe TR reached 52.17%, which may be one of the reasons for the large ultrasound measurement error in severe TR.
Interestingly, our results showed that TR-SQG IV correlation is better than TR-SQG III but consistency is worse than TR-SQG III. However, it has been shown that a strong correlation between two methods should not be construed as meaning that those same two methods are accurate and clinically reliable interchangeability. The Bland-Altman analysis has become the gold standard for comparing measurement methods since it provides two crucial information: the bias, or the difference between the means and the limits of agreement, or the range of possible errors (13). Bias informs about accuracy, and agreement informs about precision. Previous studies demonstrated that the inherent instability and/or interventions (e.g., diuretics) that occur between studies could have influenced the findings (35,36). Our hypothesis was that medication taken before to RHC may reduce the risk of RHC in patients with severe PH (US-PASP >70 mmHg), and may be the main reason for the bias and agreement gap between TR-SQG III and IV. In our analysis, a high percentage of patients with US-PASP >70 mmHg mainly existed in severe TR and TR-SQG IV (Figure 4). When the proportion of medication prior to RHC measures at different US-PASP measurements (less than 50 mmHg, 50 to 70 mmHg, and greater than 70 mmHg) were analyzed, it was obvious that preoperative medication for severe PH was necessary and may lead a result of US-PASP overestimation. The elevation of PAP in patients with CTEPH is primarily caused by underlying pulmonary vascular disease. Not surprisingly, pulmonary vasoactive medications and other interventions targeted specifically at the underlying pulmonary vascular disease benefit these patients. The hypothesis was further confirmed by postoperative comparison of US-PASP and RHC-PASP: the bias in TR-SQG IV after BPA was not significantly different from that before BPA, and the limits of agreement were both small; paired t-test showed a statistical difference in both US-PASP and RHC-PASP before BPA in both severe TR and Grade IV, but not after BPA (Figure 5). This can be explained by the shorter measurement time intervals between TTE and RHC after BPA. Although patients with PH may experience with spontaneous fluctuations in up to 22% for PAMP and 36% for PVR within only a few hours (36). Ni et al. (15) demonstrated that shortening the time interval between TTE and RHC could improve the accuracy of TTE and the diagnostic accuracy was the highest when the time period was less than or equal to 24 hours. One never knows how much spontaneous variability persists, but our findings held true at shorter intervals (within 24 hours or the same-day measurement) because any intervention would be likely to influence pulmonary pressure. Overall, PH was more accurately and precisely estimated from high-quality spectra than from TR severity.
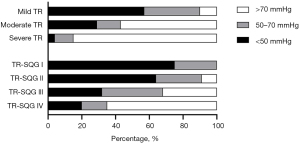
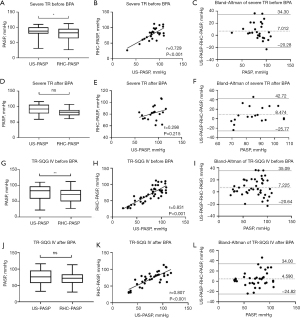
Assessing PAP based on the quality of TRS, on the one hand, allows for greater clinical confidence in the ultrasound assessment results. If the image quality is poor, as in grades I and II, the assessment may be unreliable; however, if good, as in TR-SQG III and IV, the measurement may be reliable, which may guide clinical management and referral for RHC. On the other hand, the spectrum’s quality can also be used as an indicator to test sonographers’ measurement technique. Although it is unknown whether the reliability of US-PASP based on the TRS quality will be applied in clinical practice, this study can fill the gap in the literature.
Limitations
This study has several limitations that merits emphasis. First, the present study was a small, retrospective, single-center study. Furthermore, the fact we selected subjects with CTEPH may limit external validity. Quality grading can, however, be generally used in all settings. Further studies using a larger cohort are required to investigate the application and clinical values of the spectrum grading criteria in different pathologic groups. Finally, we assumed that the RAP estimated by echocardiography from the inferior vena cava diameters and inspiratory collapse was accurate, based on which the consistency of US-PASP and RHC-PASP was analyzed. There may be reputedly imprecise (33,37), but Chen et al. (38) reported that RAMP did not change significantly before and after BPA. However, further study is needed on researching new methods of RAP ultrasound assessment in order to screen suspicious populations reliably.
Conclusions
The ability to estimate PASP reliably through echocardiography depends on the spectrum quality of the TR jet. The quality of TRS was superior to the severity of TR in PH assessing. The TRS quality grading enhanced the accuracy and precision of ultrasound PH assessment, enabling better use in clinical practice. Medication prior to RHC measures may influence correlation and agreement, particularly for severe TR with a very high PH.
Acknowledgments
We would like to thank Professor Mei Jiang from the First Affiliated Hospital of Guangzhou Medical University and the State Key Laboratory of Respiratory Diseases/National Clinical Research Center for Respiratory Disease for her guidance on the subject design and statistics, and to thank Professor Tao Wang from the First Affiliated Hospital of Guangzhou Medical University and the State Key Laboratory of Respiratory Diseases/National Clinical Research Center for Respiratory Disease for his guidance and revision for this manuscript.
Funding: The study was supported by
Footnote
Reporting Checklist: The authors have completed the STROBE reporting checklist. Available at https://jtd.amegroups.com/article/view/10.21037/jtd-23-1084/rc
Data Sharing Statement: Available at https://jtd.amegroups.com/article/view/10.21037/jtd-23-1084/dss
Peer Review File: Available at https://jtd.amegroups.com/article/view/10.21037/jtd-23-1084/prf
Conflicts of Interest: All authors have completed the ICMJE uniform disclosure form (available at https://jtd.amegroups.com/article/view/10.21037/jtd-23-1084/coif). The authors have no conflicts of interest to declare.
Ethical Statement: The authors are accountable for all aspects of the work in ensuring that questions related to the accuracy or integrity of any part of the work are appropriately investigated and resolved. The study was conducted in accordance with the Declaration of Helsinki (as revised in 2013). The study protocol was approved by the Ethics Committee at The First Affiliated Hospital of Guangzhou Medical University (Approval No. [2020]108). All patients provided written informed consent prior to participation in the current study.
Open Access Statement: This is an Open Access article distributed in accordance with the Creative Commons Attribution-NonCommercial-NoDerivs 4.0 International License (CC BY-NC-ND 4.0), which permits the non-commercial replication and distribution of the article with the strict proviso that no changes or edits are made and the original work is properly cited (including links to both the formal publication through the relevant DOI and the license). See: https://creativecommons.org/licenses/by-nc-nd/4.0/.
References
- Bossone E, Ferrara F, Grünig E. Echocardiography in pulmonary hypertension. Curr Opin Cardiol 2015;30:574-86. [Crossref] [PubMed]
- Cassady SJ, Ramani GV. Right Heart Failure in Pulmonary Hypertension. Cardiol Clin 2020;38:243-55. [Crossref] [PubMed]
- Hoeper MM, Humbert M, Souza R, et al. A global view of pulmonary hypertension. Lancet Respir Med 2016;4:306-22. [Crossref] [PubMed]
- Simonneau G, Montani D, Celermajer DS, et al. Haemodynamic definitions and updated clinical classification of pulmonary hypertension. Eur Respir J 2019;53:1801913. [Crossref] [PubMed]
- Humbert M, Kovacs G, Hoeper MM, et al. 2022 ESC/ERS Guidelines for the diagnosis and treatment of pulmonary hypertension. Eur Heart J 2022;43:3618-731. [Crossref] [PubMed]
- Augustine DX, Coates-Bradshaw LD, Willis J, et al. Echocardiographic assessment of pulmonary hypertension: a guideline protocol from the British Society of Echocardiography. Echo Res Pract 2018;5:G11-24. [Crossref] [PubMed]
- O'Leary JM, Assad TR, Xu M, et al. Lack of a Tricuspid Regurgitation Doppler Signal and Pulmonary Hypertension by Invasive Measurement. J Am Heart Assoc 2018;7:e009362. [Crossref] [PubMed]
- Galiè N, Hoeper MM, Humbert M, et al. Guidelines for the diagnosis and treatment of pulmonary hypertension: the Task Force for the Diagnosis and Treatment of Pulmonary Hypertension of the European Society of Cardiology (ESC) and the European Respiratory Society (ERS), endorsed by the International Society of Heart and Lung Transplantation (ISHLT). Eur Heart J 2009;30:2493-537. [Crossref] [PubMed]
- Singh N, Mullin CJ. Diagnosis of Pulmonary Hypertension. R I Med J (2013) 2011;104:30-5.
- Mourani PM, Sontag MK, Younoszai A, et al. Clinical utility of echocardiography for the diagnosis and management of pulmonary vascular disease in young children with chronic lung disease. Pediatrics 2008;121:317-25. [Crossref] [PubMed]
- Fisher MR, Forfia PR, Chamera E, et al. Accuracy of Doppler echocardiography in the hemodynamic assessment of pulmonary hypertension. Am J Respir Crit Care Med 2009;179:615-21. [Crossref] [PubMed]
- Rich JD, Shah SJ, Swamy RS, et al. Inaccuracy of Doppler echocardiographic estimates of pulmonary artery pressures in patients with pulmonary hypertension: implications for clinical practice. Chest 2011;139:988-93. [Crossref] [PubMed]
- D'Alto M, Romeo E, Argiento P, et al. Accuracy and precision of echocardiography versus right heart catheterization for the assessment of pulmonary hypertension. Int J Cardiol 2013;168:4058-62. [Crossref] [PubMed]
- Greiner S, Jud A, Aurich M, et al. Reliability of noninvasive assessment of systolic pulmonary artery pressure by Doppler echocardiography compared to right heart catheterization: analysis in a large patient population. J Am Heart Assoc 2014;3:e001103. [Crossref] [PubMed]
- Ni JR, Yan PJ, Liu SD, et al. Diagnostic accuracy of transthoracic echocardiography for pulmonary hypertension: a systematic review and meta-analysis. BMJ Open 2019;9:e033084. [Crossref] [PubMed]
- Venkateshvaran A, Seidova N, Tureli HO, et al. Accuracy of echocardiographic estimates of pulmonary artery pressures in pulmonary hypertension: insights from the KARUM hemodynamic database. Int J Cardiovasc Imaging 2021;37:2637-45. [Crossref] [PubMed]
- Roberts JD, Forfia PR. Diagnosis and assessment of pulmonary vascular disease by Doppler echocardiography. Pulm Circ 2011;1:160-81. [Crossref] [PubMed]
- Brugger N, Lichtblau M, Maeder M, et al. Two-dimensional transthoracic echocardiography at rest for the diagnosis, screening and management of pulmonary hypertension. Swiss Med Wkly 2021;151:w20486. [Crossref] [PubMed]
- Ferrara F, Zhou X, Gargani L, et al. Echocardiography in Pulmonary Arterial Hypertension. Curr Cardiol Rep 2019;21:22. [Crossref] [PubMed]
- D'Alto M, Bossone E, Opotowsky AR, et al. Strengths and weaknesses of echocardiography for the diagnosis of pulmonary hypertension. Int J Cardiol 2018;263:177-83. [Crossref] [PubMed]
- Li Y, Wang Y, Li H, et al. Evaluation of the hemodynamics and right ventricular function in pulmonary hypertension by echocardiography compared with right-sided heart catheterization. Exp Ther Med 2017;14:3616-22. [Crossref] [PubMed]
- Correale M, Tricarico L, Padovano G, et al. Echocardiographic score for prediction of pulmonary hypertension at catheterization: the Daunia Heart Failure Registry. J Cardiovasc Med (Hagerstown) 2019;20:809-15. [Crossref] [PubMed]
- Sanna GD, Parodi G. Accuracy of echocardiography in pulmonary hypertension: thinking outside of the box beyond the Achilles' heel of right atrial pressure estimation. Int J Cardiovasc Imaging 2021;37:2647-9. [Crossref] [PubMed]
- Jankowich M, Maron BA, Choudhary G. Mildly elevated pulmonary artery systolic pressure on echocardiography: bridging the gap in current guidelines. Lancet Respir Med 2021;9:1185-91. [Crossref] [PubMed]
- Lancellotti P, Tribouilloy C, Hagendorff A, et al. Recommendations for the echocardiographic assessment of native valvular regurgitation: an executive summary from the European Association of Cardiovascular Imaging. Eur Heart J Cardiovasc Imaging 2013;14:611-44. [Crossref] [PubMed]
- Schneider M, Pistritto AM, Gerges C, et al. Multi-view approach for the diagnosis of pulmonary hypertension using transthoracic echocardiography. Int J Cardiovasc Imaging 2018;34:695-700. [Crossref] [PubMed]
- Lin JL, Chen HM, Lin FC, et al. Application of DynaCT angiographic reconstruction in balloon pulmonary angioplasty. Eur Radiol 2020;30:6950-7. [Crossref] [PubMed]
- Rosenkranz S, Preston IR. Right heart catheterisation: best practice and pitfalls in pulmonary hypertension. Eur Respir Rev 2015;24:642-52. [Crossref] [PubMed]
- D'Alto M, Dimopoulos K, Coghlan JG, et al. Right Heart Catheterization for the Diagnosis of Pulmonary Hypertension: Controversies and Practical Issues. Heart Fail Clin 2018;14:467-77. [Crossref] [PubMed]
- Bossone E, D'Andrea A, D'Alto M, et al. Echocardiography in pulmonary arterial hypertension: from diagnosis to prognosis. J Am Soc Echocardiogr 2013;26:1-14. [Crossref] [PubMed]
- Platts DG, Vaishnav M, Burstow DJ, et al. Contrast microsphere enhancement of the tricuspid regurgitant spectral Doppler signal - Is it still necessary with contemporary scanners? Int J Cardiol Heart Vasc 2017;17:1-10. [Crossref] [PubMed]
- Jeon DS, Luo H, Iwami T, et al. The usefulness of a 10% air-10% blood-80% saline mixture for contrast echocardiography: Doppler measurement of pulmonary artery systolic pressure. J Am Coll Cardiol 2002;39:124-9. [Crossref] [PubMed]
- Özpelit E, Akdeniz B, Özpelit EM, et al. Impact of Severe Tricuspid Regurgitation on Accuracy of Echocardiographic Pulmonary Artery Systolic Pressure Estimation. Echocardiography 2015;32:1483-90. [Crossref] [PubMed]
- Lv GJ, Li AL, Tao XC, et al. The accuracy and influencing factors of Doppler echocardiography in estimating pulmonary artery systolic pressure: comparison with right heart catheterization: a retrospective cross-sectional study. BMC Med Imaging 2022;22:91. [Crossref] [PubMed]
- Rich S, D'Alonzo GE, Dantzker DR, et al. Magnitude and implications of spontaneous hemodynamic variability in primary pulmonary hypertension. Am J Cardiol 1985;55:159-63. [Crossref] [PubMed]
- Ziegler MU, Reinelt H. The heart catheter table is not the operating table: Intraindividual comparison of pulmonary artery pressures. Anaesthesist 2018;67:351-8. [Crossref] [PubMed]
- Beigel R, Cercek B, Luo H, et al. Noninvasive evaluation of right atrial pressure. J Am Soc Echocardiogr 2013;26:1033-42. [Crossref] [PubMed]
- Chen J, Ding S, Zhang C, et al. Clinical value of echocardiography in evaluating hemodynamics and right ventricular function in patients with chronic thromboembolic pulmonary hypertension after balloon pulmonary angioplasty. J Thorac Dis 2022;14:1401-10. [Crossref] [PubMed]