Postoperative C-reactive protein levels correlate with reduced spinal column mobility after median sternotomy: a prospective cohort study
Highlight box
Key findings
• Spinal range of motion showed a decrease in thoracic spine range of motion after median sternotomy. Perioperative factors involved in decreased range of motion were indicated by C-reactive protein measurements on postoperative day 3.
What is known and what is new?
• The only studies investigating the effects of a median sternotomy on the spine reported results in thoracic spine specimens.
• Therefore, in vivo results may be different and were investigated in this study. Perioperative related factors were also investigated.
What is the implication, and what should change now?
• Appropriate rehabilitation to improve decreased range of motion and interventions to reduce postoperative inflammation are needed.
Introduction
Background
In Japan, the number of cardiac surgeries is on the rise due to the high incidence of cardiovascular diseases (1). The indications for cardiac surgery are expanding due to the aging population, technological advances, aggressive surgical intervention, and advances in postoperative management. The number of cardiac operations surveyed by the Japanese Society of Cardiology increased from 72,572 for a population of 126,706 million in 2017 to 73,294 for a population of 125,502 million in 2021 (2). Median sternotomy is often used as a technique during cardiac surgery; the sternum is incised longitudinally and opened using a sternal spreader to secure the surgical field of view. Postoperatively, the sternum is fixed with thread, wire, or a sternal plate.
The sternum, together with the thoracic vertebrae and ribs, forms the thoracic cage, which is connected by the costovertebral and sternocostal joints consisting of the transverse costovertebral and costovertebral head joints. The thoracic cage contributes to the stability of the thoracic spine (3) and is a stronger and more stable structure than the cervical and lumbar spine. Median sternotomy is predicted to disrupt this thoracic structure, causing dysfunction of the spinal column.
Generally, changes in spinal alignment and range of motion cause decreased respiratory function (4,5); strength reduction in the trunk flexor, trunk extensor, and thoracic flexor muscles (6); and decreased grip strength (7). This reduces mobility (8) and balance (9), leading to falls and necessitating interventions to limit or reverse the changes in spinal alignment. However, after a median sternotomy, interventions on the spine are restricted for approximately 3 months until bony fusion of the sternum is achieved; trunk rotation, upper limb movements, and carrying heavy objects may cause displacement of the sternotomy site (10).
Rationale and knowledge gap
Although studies on postoperative changes in the spine due to other surgical methods have been reported in vivo (11), the only studies that have examined the effects of a mid-thoracic incision on the spine have used thoracic spine specimens (12-14). Therefore, the range of motion in vivo, including soft tissues and surrounding muscles, has not been examined. Furthermore, the effects of postoperative inflammation (15) and rehabilitation (16), which are known to cause perioperative complications and changes in physical function, have not been examined.
Objective
It is important to investigate the post-median sternotomy changes in the range of motion of the spine in vivo. This study aimed to compare the range of motion of the spine before and after cardiac surgery through a median sternotomy and investigate the effects of perioperative biological reactions and rehabilitation on the spine. We present this article in accordance with the STROBE reporting checklist (available at https://jtd.amegroups.com/article/view/10.21037/jtd-23-1439/rc).
Methods
Study design and population
We carried out a prospective cohort study. Participants were recruited from the Department of Cardiac Surgery, International University of Health and Welfare Hospital from April 2017 to March 2021. Inclusion criteria were as follows: age ≥18 years and having undergone cardiac surgery through a median sternal incision on a standby basis. Patients with a history of neurological or spinal disease, those who had difficulty holding a standing or forward-bent position due to cognitive decline or complications, and those with missing data were excluded. The sample size was determined assuming that multiple regression analysis would be performed. Assuming an effect size f2=0.15, α=0.05, 1 − β=0.8, and number of predictors =8, a sample of 109 was required.
Subsequently, assuming that 10–20% of the total sample would be excluded, the number of participants was set at 130.
This study was conducted in accordance with the Declaration of Helsinki (as revised in 2013). This study was approved by the ethical committee of the International University of Health and Welfare (approval No. 17-Io-138). Participants were informed of the content and purpose of the study in advance, and their verbal and written informed consents were obtained.
Measurements
Basic information on the participants, including age, sex, height, weight, body mass index (BMI), indication for surgery, New York Heart Association classification, complications, and preoperative activities of daily living BMI status, was obtained from medical records.
Spinal motion measurement
Spinal range of motion was measured using the Spinal Mouse (Idiag, Volkerswill, Switzerland) device in a noninvasive, reliable, and reproducible way. This device’s measurements have been shown to correlate with X-rays (17,18). The standard error of measurement varies from 0.61 to 13.18 degrees, depending on the area of interest (17-19). Measurements were taken preoperatively, up to 1 week before surgery, and postoperatively, before discharge. The measurement positions were at rest in a standing position and the trunk forward-bending position. In each position, both feet were parallel to each other, with a foot width of 10 cm between the medial phalanges, and both upper limbs were in a naturally drooping position. In the upright position, the head was kept gazing forward at eye level, and the trunk was in forward-bending and backward-bending positions, sequentially; the head was kept in the middle position between forward and backward bending. The trunk forward-bending was performed at maximum effort. By tracing the paraspinal area from the seventh cervical vertebra to the third sacral vertebra, the sacral range of motion, lumbar range of motion, thoracic range of motion, and overall tilt range of motion were measured from the upright position to the trunk forward-bending position.
Perioperative information
Perioperative information on the procedure, sternal fixation method, operative time, intraoperative blood loss, and C-reactive protein (CRP) on the third postoperative day were extracted from the medical records. The postoperative elevation of CRP (postoperative day 3) is a representative laboratory value that indicates the degree of systemic inflammatory response. Inflammation has been reported to cause functional symptoms due to inflammatory pain, edema and swelling, and is associated with postoperative complications.
The following sternal fixation methods were used after sternotomy: Ethibond Excel thread (Johnson & Johnson, New Brunswick, NJ, USA) (20), Yokozuna Wire (Matsuda Medical Industries, Tokyo, Japan) (21), Grand Fix sternal pin (Gunze, Tokyo, Japan) (22), SternaLock sternal plate (BIOMET, Warsaw, IN, USA) (23), and Super Fixove MX mesh plate (Teijin Medical Technologies, Osaka, Japan) (24).
Perioperative rehabilitation
After evaluation of physical function and consultation with the attending physician, exercise therapy to maintain physical function in accordance with cardiac function and respiratory training to prevent postoperative complications were commenced preoperatively. Postoperatively, patients began early mobilization following consultation with the attending physician 1 day after surgery. The starting of mobilization, the rehabilitation schedule, and exercise loading were performed under the supervision of a physical therapist in accordance with the Guidelines for Rehabilitation in Cardiovascular Disease (25). Rehabilitation progressed with interventions aimed at achieving independence on the ward within 4 to 5 days of commencing mobilization.
The date and stage of postoperative mobilization were investigated. The date was defined as the date when the head of the bed was first elevated. A numerical value was assigned to each stage of progression as follows: 1: bed rest, 2: elevation of the head of the bed, 3: end-sitting, 4: standing, 5: stepping, 6: indoor walking, 7: 50 m walking, and 8: 100 m walking. The numerical value achieved on postoperative day 2 was recorded.
Statistical analysis
The normality of each variable was checked with the Kolmogorov-Smirnov test. The paired t-test was used for normally distributed variables before and after surgery for each factor, and the Wilcoxon signed-rank test for non-normally distributed variables. Multiple regression analysis (forced entry method) was then performed using the amount of change in spinal range of motion as the dependent variable; surgery time, CRP on the third postoperative day, the start of postoperative weaning, and the weaning stage on the second postoperative day as perioperative factors, and age, sex, BMI, and days until postoperative measurement as adjustment variables. Multicollinearity was checked using the variance inflation factor (VIF) and normality of residuals using the Shapiro-Wilk test. Statistical tests were performed using the statistical analysis software SPSS 25 (IBM Corp., Armonk, NY, USA). As an a posteriori test, G Power was used to test for effect size and power. In addition, as a subanalysis, a correlation analysis was performed between the amount of change in spinal range of motion and sex and the factors that showed a significant relationship in the multiple regression analysis. Spearman’s rank correlation coefficient was used for correlation analysis. The probability of significance was set at 5%.
Results
There were 126 participants in the study. After excluding 33 participants who met the exclusion criteria (18 with spinal disease, four with neurological disease, seven with difficulty maintaining standing and forward bending positions, one with postoperative neurological complications, and three with missing measurements), 93 participants (60 males and 33 females; age: 66.1±11.6 years; height: 161.4±9.4 cm; weight: 56.9±10.5 kg; BMI: 21.8±3.1 kg/ m²) were included in the analysis (Figure 1). The basic attributes of the analyzed participants are shown in Table 1. Differences were observed between men and women in the number of comorbidities, surgical procedures, and sternal fixation methods.
Table 1
Variables | Total (n=93) | Male (n=60) | Female (n=33) | P value |
---|---|---|---|---|
Age (years) | 66.1±11.6 | 64.5±12.8 | 69.1±8.4 | n.s |
BMI (kg/m2) | 21.8±3.1 | 22.2±3.0 | 21.0±3.2 | n.s |
Preoperative | ||||
Disease state | ||||
Aortic valve stenosis | 18 [19] | 12 [20] | 6 [18] | n.s |
Aortic regurgitation | 24 [26] | 16 [27] | 8 [24] | n.s |
Mitral valve stenosis | 2 [2] | 0 [0] | 2 [6] | n.s |
Mitral regurgitation | 44 [47] | 22 [37] | 22 [67] | <0.05† |
Tricuspid regurgitation | 29 [31] | 10 [17] | 19 [58] | <0.05† |
Coronary artery disease | 25 [27] | 18 [30] | 7 [21] | n.s |
Atrial fibrillation | 28 [30] | 15 [25] | 13 [39] | n.s |
Thoracic aortic aneurysm | 2 [2] | 2 [3] | 0 [0] | n.s |
Hypertrophic cardiomyopathy | 2 [2] | 1 [2] | 1 [3] | n.s |
Cardiac myxoma | 1 [1] | 0 [0] | 1 [3] | n.s |
Ventricular aneurysm | 1 [1] | 1 [2] | 0 [0] | n.s |
Ventricular septal defect | 1 [1] | 0 [0] | 1 [3] | n.s |
Atrial septal defect | 1 [1] | 0 [0] | 1 [3] | n.s |
Constrictive pericarditis | 1 [1] | 1 [2] | 0 [0] | n.s |
Patent foramen ovale | 1 [1] | 0 [0] | 1 [3] | n.s |
Prosthetic valve infection | 1 [1] | 1 [2] | 0 [0] | n.s |
High blood pressure | 75 [81] | 50 [83] | 25 [76] | n.s |
Hyperlipidemia | 25 [27] | 16 [27] | 9 [27] | n.s |
Diabetes | 25 [27] | 15 [25] | 10 [30] | n.s |
Kidney failure | 14 [15] | 7 [12] | 7 [21] | n.s |
New York Heart Association | ||||
I | 17 [18] | 14 [23] | 3 [9] | n.s |
II | 61 [66] | 37 [62] | 24 [73] | n.s |
III | 12 [13] | 6 [10] | 6 [18] | n.s |
IV | 3 [3] | 3 [5] | 0 [0] | n.s |
Activities of daily living state | ||||
Independence | 93 [100] | 60 [100] | 33 [100] | n.s |
Postoperative | ||||
Type of cardiac surgery | ||||
Aortic valve replacement | 42 [45] | 28 [47] | 14 [42] | n.s |
Mitral valve replacement | 19 [20] | 6 [10] | 13 [39] | <0.05† |
Mitral valvuloplasty | 26 [28] | 16 [27] | 10 [30] | n.s |
Tricuspid annuloplasty | 32 [34] | 12 [20] | 20 [61] | <0.05† |
Coronary artery bypass surgery | 25 [27] | 16 [27] | 9 [27] | n.s |
Maze | 11 [12] | 5 [8] | 6 [18] | n.s |
Pulmonary vein isolation | 20 [22] | 11 [18] | 9 [27] | n.s |
Morrow | 3 [3] | 2 [3] | 1 [3] | n.s |
Tumor resection | 2 [2] | 1 [2] | 1 [3] | n.s |
Patent foramen ovale closure | 2 [2] | 2 [3] | 0 [0] | n.s |
Total arch replacement | 1 [1] | 0 [0] | 1 [3] | n.s |
Ventricular septal defect closure | 1 [1] | 0 [0] | 1 [3] | n.s |
Sternum fixation method | ||||
String | 13 [14] | 6 [10] | 7 [21] | n.s |
Wire | 81 [87] | 55 [92] | 26 [79] | n.s |
Sternal pin | 15 [16] | 5 [8] | 10 [30] | <0.05† |
Sternal plate | 70 [75] | 48 [80] | 22 [67] | n.s |
Data are presented as n [%] or mean ± SD. Some overlap in disease state, type of cardiac surgery, and sternal fixation method. †, chi-squared test. n.s, not significant; BMI, body mass index; SD, standard deviation.
Pre- and postoperative spinal range of motion is shown in Table 2. Thoracic spine range of motion was 13.7°±14.3° preoperatively and 8.8°±15.6° postoperatively, showing a significant decrease after surgery (P<0.05; r=0.40; 1 − β=0.96). There were no significant differences in sacral range of motion, lumbar spine range of motion, or overall tilt range of motion.
Table 2
Upright position-trunk forward bending position | Preoperative | Postoperative | P value |
---|---|---|---|
Sacral range of motion (°) | 55.3±20.2 | 56.7±19.3 | 0.39 |
Thoracic spine range of motion (°) | 13.7±14.3 | 8.8±15.6 | <0.01 |
Lumbar spine range of motion (°) | 39.3±14.5 | 39.2±13.6 | 0.82 |
Overall tilt range of motion (°) | 90.8±23.8 | 91.6±22.4 | 0.63 |
Data are presented as mean ± SD. SD, standard deviation.
The results of multiple regression analysis with thoracic range of motion as the dependent variable are shown in Table 3. Multiple regression analysis showed that an increase in CRP on the third postoperative day was responsible for the decrease in thoracic range of motion (β=−0.30; P<0.01). VIF was all less than 10, showing no multi-collinearity, and the Durbin-Watson ratio was 1.962. The Shapiro-Wilk test was performed on the residuals, and the result was 0.74, confirming normality.
Table 3
Variables | Partial regression coefficient | Standard partial regression coefficient | P value | R2 |
---|---|---|---|---|
Constant | 29.03 | – | – | – |
Age | −0.04 | 0.12 | 0.72 | – |
Sex | −2.15 | 2.88 | 0.46 | – |
BMI | −0.69 | 0.42 | 0.11 | – |
Days to postoperative measurement | −0.25 | 0.21 | 0.24 | – |
Surgery duration | −0.01 | 0.01 | 0.62 | – |
Start date of getting out of bed | 1.39 | 1.98 | 0.49 | – |
Weaning phase on the second postoperative day | −0.47 | 1.14 | 0.68 | – |
CRP on postoperative day 3 | −0.94 | 0.41 | 0.02 | – |
Durbin-Watson: 1.96 | – | – | – | 0.15 |
BMI, body mass index; CRP, C-reactive protein.
In the sub-analysis, correlation analysis was performed between the change in thoracic spine range of motion and CRP on postoperative day 3 (5.9±3.5 mg/dL for men and 6.0±4.1 mg/dL for women; Figure 2). The results showed that women were more likely to have a decreased range of thoracic spine motion with higher CRP on postoperative day 3 compared to men.
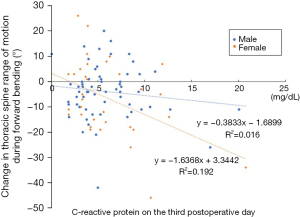
Discussion
Key findings
This study was conducted on patients who underwent cardiac surgery through a median sternotomy with the aim of analyzing pre- and postoperative changes in spinal range of motion and the factors contributing to these changes. Comparison of pre- and postoperative spinal range of motion showed a decrease in thoracic spine range of motion postoperatively. Perioperative factors involved in the reduced thoracic spine range of motion were examined, demonstrating a correlation with postoperative day 3 CRP measurements. The elevated CRP may be associated with a reduction in thoracic spine range of motion due to edema around the wound and thoracic area.
Spine range of motion
Mannen et al. (12) and Brasiliense et al. (13) previously used thoracic specimens to investigate changes to spinal range of motion during sternotomy and reported an increase in thoracic spine range of motion due to disruption of thoracic cage stability. In addition, Liebsch et al. (14) investigated thoracic spine range of motion before sternal manipulation, after median sternotomy, and after sternal wire fastening. They reported that thoracic stability improved after wire fastening compared to that after median sternotomy, and thoracic spine hypermobility was reduced; however, it did not return to baseline. In the present study, only thoracic spine range of motion was significantly decreased postoperatively, a result that differed from previous studies using thoracic specimens. Thoracic spine specimens may not reflect actual range of motion because surrounding muscles and soft tissues are excluded. It is possible that perioperative biological reactions, which have not yet been analyzed, may be influencing the results.
Range of motion changes and perioperative factors
A factor analysis of several perioperative factors revealed that the decreased thoracic spine range of motion was associated with a higher CRP on the third postoperative day, indicating that the degree of inflammation was important. CRP is a type of acute phase protein which is synthesized in the liver and released into the blood when inflammation or tissue destruction occurs. The postoperative rise in CRP could be interpreted as an immune response to prevent cellular damage and destroy pathogens that may have infiltrated the body during the surgical intervention. CRP is widely used in clinical practice as an inflammatory marker and begins to rise within a few hours of surgery, reaching a maximum value between 48 and 72 hours postoperatively. Therefore, an elevated CRP on the third postoperative day is a useful predictor of increased postoperative complications and poor prognosis (26,27). There are multiple possible mechanisms for the correlation between elevated CRP 3 days postoperatively and the thoracic kyphosis angle change in the postoperative forward-bending position. The first mechanism is enhanced and prolonged edema. During inflammation, chemical transmitters such as histamine and serotonin released from mast cells and other substances cause vasodilation and increased vascular permeability, leading to edema (28). Since the participants in this study underwent cardiac surgery, their circulatory function was already impaired (29), further prolonging any edema. Edema is one factor affecting joint range of motion (30). Since the sternotomy surgical site was located on the anterior aspect of the chest, it is possible that localized inflammatory edema around the wound limited thoracic spine movement in the trunk forward-bending position. Second, the higher the inflammatory marker value, the more likely that scarring will progress (31). Scarring of the surgical wound may have reduced thoracic cage movement due to the reduced mobility of the soft tissue around the wound. Third, muscle weakness due to protein catabolism may have occurred. The higher the inflammatory marker level, the higher the protein catabolism, and the more likely that postoperative muscle weakness will occur (32). Thoracic spine mobility requires pelvic fixation and control by the muscles connecting the pelvis and thoracic spine (33); protein catabolism may have affected these muscles.
We performed a correlation subanalysis investigating the amount of change in thoracic spine range of motion, CRP on the third postoperative day, and sex, because many previous studies have reported differing postural characteristics in men and women (34,35). The results showed that the range of thoracic spine motion decreased as CRP increased, especially in women. There were no differences in CRP levels between men and women on the third postoperative day, suggesting that women are more likely than men to experience changes in the same inflammation markers. It is possible that women were more susceptible to a reduction in mobility due to lower muscle strength and morphological differences associated with breast tissue in the chest area. However, there were methodological differences between the surgeries completed in men and women included in this study, including the method of fixation after sternotomy. Therefore, further research is needed in this area.
Strengths and limitations
The purpose of this study was to compare the range of motion of the spine before and after a median sternotomy and to analyze the factors that contribute to this range of motion. This is the first in vivo study to investigate the effects of a midline sternotomy and associated factors. Participants who experienced no effect on forward bending movements were included, and multiple adjustment variables were employed during multiple regression analysis and subanalysis. However, this study has several limitations. First, the study only included individuals with high baseline physical function. Due to the aging Japanese population, the number of patients with comorbidities and impaired physical function undergoing cardiac surgery is increasing. Individuals with poorer baseline function who were excluded due to their inability to stand or bend forward may have differing results. Second, participants had a wide range of indications for surgery, including surgeries with parasternal blood vessel manipulation; the impact that surgical indications have on outcomes need to be further investigated. Third, long-term changes were unknown as our study focused only on the perioperative period. The incised sternum fuses in approximately 3 months and the range of motion is then no longer limited; thus, the loss of range of motion may gradually improve. Fourth, the standard error of the measurement instrument used in this study, the Spinal Mouse, has been reported in a wide range from 1.0 to 5.5 in thoracic flexion (17,19). This suggests that the results of this study may be within the measurement error range and should be interpreted with caution.
Implications and actions needed
We believe that further research involving different measurement methods, increased sample sizes, and long-term follow-up is required. These studies will allow us to examine the significance of new rehabilitation interventions and perioperative management.
Conclusions
This study was conducted on patients who underwent cardiac surgery through a median sternotomy with the aim of analyzing pre- and postoperative changes in spinal range of motion and the factors contributing to these changes. Comparison of pre- and postoperative spinal range of motion showed a decrease in thoracic spine range of motion postoperatively. Perioperative factors involved in the reduced thoracic spine range of motion were examined, demonstrating a correlation with postoperative day 3 CRP measurements. The elevated CRP may be associated with a reduction in thoracic spine range of motion due to edema around the wound and thoracic area. However, the results of this study could also be due to standard errors of the equipment and further investigation and study is required.
Acknowledgments
We would like to thank the Faculty of Cardiac Surgery and Rehabilitation at the International University of Health and Welfare for their advice and guidance. We would also like to thank all participants for their cooperation.
Funding: This research was supported by a
Footnote
Reporting Checklist: The authors have completed the STROBE reporting checklist. Available at https://jtd.amegroups.com/article/view/10.21037/jtd-23-1439/rc
Data Sharing Statement: Available at https://jtd.amegroups.com/article/view/10.21037/jtd-23-1439/dss
Peer Review File: Available at https://jtd.amegroups.com/article/view/10.21037/jtd-23-1439/prf
Conflicts of Interest: All authors have completed the ICMJE uniform disclosure form (available at https://jtd.amegroups.com/article/view/10.21037/jtd-23-1439/coif). A.I. reports Grant-in-Aid for Scientific Research (JSPS KAKENHI grant No. 19K19842). The other authors have no conflicts of interest to declare.
Ethical Statement: The authors are accountable for all aspects of the work in ensuring that questions related to the accuracy or integrity of any part of the work are appropriately investigated and resolved. The study was conducted in accordance with the Declaration of Helsinki (as revised in 2013). This study was approved by the ethical committee of the International University of Health and Welfare (approval No. 17-Io-138) and informed consents were obtained from all individual participants.
Open Access Statement: This is an Open Access article distributed in accordance with the Creative Commons Attribution-NonCommercial-NoDerivs 4.0 International License (CC BY-NC-ND 4.0), which permits the non-commercial replication and distribution of the article with the strict proviso that no changes or edits are made and the original work is properly cited (including links to both the formal publication through the relevant DOI and the license). See: https://creativecommons.org/licenses/by-nc-nd/4.0/.
References
- Ministry of Health, Labour and Welfare. Overview of the 2020 patient survey. [Cited September 12, 2023]. Available online: https://www.mhlw.go.jp/toukei/saikin/hw/kanja/20/index.html
- The Japanese Circulation Society. Report on the survey of cardiovascular disease clinical practice in FY2021. [Cited September 12, 2023]. Available online: https://www.j-circ.or.jp/jittai_chosa/media/jittai_chosa2020web_1.pdf
- Mannen EM, Anderson JT, Arnold PM, et al. Mechanical analysis of the human cadaveric thoracic spine with intact rib cage. J Biomech 2015;48:2060-6. [Crossref] [PubMed]
- Culham EG, Jimenez HA, King CE. Thoracic kyphosis, rib mobility, and lung volumes in normal women and women with osteoporosis. Spine (Phila Pa 1976) 1994;19:1250-5. [Crossref] [PubMed]
- Attali V, Clavel L, Rouch P, et al. Compensation of Respiratory-Related Postural Perturbation Is Achieved by Maintenance of Head-to-Pelvis Alignment in Healthy Humans. Front Physiol 2019;10:441. [Crossref] [PubMed]
- Yang SR, Kim K, Park SJ, et al. The effect of thoracic spine mobilization and stabilization exercise on the muscular strength and flexibility of the trunk of chronic low back pain patients. J Phys Ther Sci 2015;27:3851-4. [Crossref] [PubMed]
- Hira K, Nagata K, Hashizume H, et al. Relationship of sagittal spinal alignment with low back pain and physical performance in the general population. Sci Rep 2021;11:20604. [Crossref] [PubMed]
- Sangtarash F, Manshadi FD, Sadeghi A. The relationship of thoracic kyphosis to gait performance and quality of life in women with osteoporosis. Osteoporos Int 2015;26:2203-8. [Crossref] [PubMed]
- van der Jagt-Willems HC, de Groot MH, van Campen JP, et al. Associations between vertebral fractures, increased thoracic kyphosis, a flexed posture and falls in older adults: a prospective cohort study. BMC Geriatr 2015;15:34. [Crossref] [PubMed]
- Brocki BC, Thorup CB, Andreasen JJ. Precautions related to midline sternotomy in cardiac surgery: a review of mechanical stress factors leading to sternal complications. Eur J Cardiovasc Nurs 2010;9:77-84. [Crossref] [PubMed]
- Ito A, Iijima S. Changes in spinal alignment one month post abdominal surgery: A prospective cohort study. Medicine (Baltimore) 2023;102:e33674. [Crossref] [PubMed]
- Mannen EM, Anderson JT, Arnold PM, et al. Mechanical Contribution of the Rib Cage in the Human Cadaveric Thoracic Spine. Spine (Phila Pa 1976) 2015;40:E760-6. [Crossref] [PubMed]
- Brasiliense LB, Lazaro BC, Reyes PM, et al. Biomechanical contribution of the rib cage to thoracic stability. Spine (Phila Pa 1976) 2011;36:E1686-93. [Crossref] [PubMed]
- Liebsch C, Graf N, Wilke HJ. EUROSPINE 2016 FULL PAPER AWARD: Wire cerclage can restore the stability of the thoracic spine after median sternotomy: an in vitro study with entire rib cage specimens. Eur Spine J 2017;26:1401-7. [Crossref] [PubMed]
- Ferreira LO, Vasconcelos VW, Lima JS, et al. Biochemical Changes in Cardiopulmonary Bypass in Cardiac Surgery: New Insights. J Pers Med 2023;13:1506. [Crossref] [PubMed]
- Halfwerk FR, Wielens N, Hulskotte S, et al. A mobilization poster stimulates early in-hospital rehabilitation after cardiac surgery: a prospective sequential-group study. J Cardiothorac Surg 2023;18:83. [Crossref] [PubMed]
- Mannion AF, Knecht K, Balaban G, et al. A new skin-surface device for measuring the curvature and global and segmental ranges of motion of the spine: reliability of measurements and comparison with data reviewed from the literature. Eur Spine J 2004;13:122-36. [Crossref] [PubMed]
- Kellis E, Adamou G, Tzilios G, et al. Reliability of spinal range of motion in healthy boys using a skin-surface device. J Manipulative Physiol Ther 2008;31:570-6. [Crossref] [PubMed]
- Bayartai ME, Luomajoki H, Tringali G, et al. Differences in spinal posture and mobility between adults with obesity and normal weight individuals. Sci Rep 2023;13:13409. [Crossref] [PubMed]
- Desai NB, Bhat PSS. Clinical Equivalence of Trubond® and Ethibond® Braided Polyester Sutures for Valvular Prosthesis Fixation During Aortic or Mitral Valve Replacement: A Single-Blind Randomized Controlled Trial. Cureus 2023;15:e41117. [Crossref] [PubMed]
- Eraqi M, Diab AH, Matschke K, et al. Confirmation of Safety of Titanium Wire in Sternotomy Closure, A Randomized Prospective Study. Thorac Cardiovasc Surg 2023; Epub ahead of print. [Crossref]
- Takahara S, Sasaki K, Saito T, et al. Clinical assessment of efficacy of poly-L-lactide sternal pin on sternal stability and post-operative pain: a prospective randomized trial in cardiovascular surgery. J Thorac Dis 2022;14:76-89. [Crossref] [PubMed]
- Chou SS, Sena MJ, Wong MS. Use of SternaLock plating system in acute treatment of unstable traumatic sternal fractures. Ann Thorac Surg 2011;91:597-9. [Crossref] [PubMed]
- Morimoto K, Matsushita T, Masuda S, et al. The Novel Technique of Sternal Closure With Absorbable Mesh for Osteoporotic Patients. Heart Lung Circ 2021;30:e65-7. [Crossref] [PubMed]
- The Japanese Circulation Society. Cardiovascular rehabilitation guideline. 2012. Available online: https://www.jacr.jp/pdf/RH_JCS2012_nohara_h_2015.01.14.pdf
- Kano K, Tamagawa H, Sawazaki S, et al. The Postoperative C-Reactive Protein Level Is an Early Predictor of Infectious Complications after Gastric Cancer Resection. Gan To Kagaku Ryoho 2015;42:1256-8.
- van Winsen M, McSorley ST, McLeod R, et al. Postoperative C-reactive protein concentrations to predict infective complications following gastrectomy for cancer. J Surg Oncol 2021;124:1060-9. [Crossref] [PubMed]
- Dudek-Makuch M, Studzińska-Sroka E. Horse chestnut-efficacy and safety in chronic venous insufficiency: an overview. Rev Bras Farmacogn 2015;25:533-41.
- Moreo A, Gordini V, Ciliberto GR, et al. Left ventricular performance in chronic mitral regurgitation: temporal response to valve repair and prognostic value of early postoperative echocardiographic parameters. Ital Heart J 2000;1:122-7.
- Sobiech M, Czępińska A, Zieliński G, et al. Does Application of Lymphatic Drainage with Kinesiology Taping Have Any Effect on the Extent of Edema and Range of Motion in Early Postoperative Recovery following Primary Endoprosthetics of the Knee Joint? J Clin Med 2022;11:3456. [Crossref] [PubMed]
- Ogawa R. Keloid and Hypertrophic Scars Are the Result of Chronic Inflammation in the Reticular Dermis. Int J Mol Sci 2017;18:606. [Crossref] [PubMed]
- Kramer F, Torzewski J, Kamenz J, et al. Interleukin-1beta stimulates acute phase response and C-reactive protein synthesis by inducing an NFkappaB- and C/EBPbeta-dependent autocrine interleukin-6 loop. Mol Immunol 2008;45:2678-89. [Crossref] [PubMed]
- Mohanty PP, Pattnaik M. Mobilisation of the thoracic spine in the management of spondylolisthesis. J Bodyw Mov Ther 2016;20:598-603. [Crossref] [PubMed]
- Hay O, Dar G, Abbas J, et al. The Lumbar Lordosis in Males and Females, Revisited. PLoS One 2015;10:e0133685. [Crossref] [PubMed]
- Yukawa Y, Kato F, Suda K, et al. Normative data for parameters of sagittal spinal alignment in healthy subjects: an analysis of gender specific differences and changes with aging in 626 asymptomatic individuals. Eur Spine J 2018;27:426-32. [Crossref] [PubMed]