A risk score for predicting extracorporeal membrane oxygenation support before lung transplantation
Highlight box
Key findings
• Establishing a reliable and practical risk score for predicting intraoperative extracorporeal membrane oxygenation (ECMO) support before lung transplantation.
What is known and what is new?
• ECMO has been used more and more in lung transplantation. Implementing ECMO without planning or choosing the wrong patients for ECMO implementation could waste medical resources, raise medical costs, and increase the risk of postoperative complications and mortality.
• Our results reveal this simple risk score model as an effective predictor for the need of intraoperative ECMO and stratify high-risk patients who require ECMO support.
What is the implication, and what should change now?
• Before lung transplantation, it is essential to identify the risk factors linked to intraoperative ECMO requirements and promptly intervene in high-risk patients.
Introduction
Background
Lung transplantation was first introduced in 1963 (1), and the first successful lung transplantation was performed in Toronto in 1983 (2). Lung transplantation is recognized worldwide as an effective method for treating end-stage lung disease, improving the quality of life, and prolonging patient survival (3). However, due to preoperative respiratory failure, pulmonary hypertension, and cardiac dysfunction, critically ill patients often require cardiopulmonary support to manage hemodynamic fluctuations and maintain oxygenation during lung transplantation (4,5).
Extracorporeal membrane oxygenation (ECMO) has been implemented in clinical practice since 1972 (6), which has become an indispensable auxiliary support technology in the clinical treatment of critically ill patients (7-10) to provide both blood flow support and in vitro gas exchange. However, ECMO is an invasive procedure that is associated with significant complications. Patients receiving ECMO have a higher risk of secondary bleeding due to systemic anticoagulation and lack of coagulation factors. Blood transfusion may increase the incidence of primary graft dysfunction (PGD) in patients undergoing lung transplant patients (11-13). Other complications include cardiac tamponade, infection, thrombosis, and gas embolism, which may delay postoperative recovery and increase mortality (14-16).
Rationale and knowledge gap
Implementation of ECMO during transplantation without prediction or improper patient selection may lead to wastage of medical resources, increased medical costs, and an increased incidence of postoperative complications and mortality (17,18). Therefore, it is necessary to identify the risk factors associated with intraoperative ECMO requirements before lung transplantation and provide timely intervention in high-risk patients. However, only a few studies have addressed this issue. Currently, no clinical risk model can predict ECMO support for lung transplantation.
Objective
This study’s primary outcome is identifying risk factors for predicting ECMO support at lung transplantation and developing an accurate and convenient risk score to identify high-risk patients who need intraoperative ECMO support. We present this article in accordance with the TRIPOD reporting checklist (available at https://jtd.amegroups.com/article/view/10.21037/jtd-23-452/rc).
Methods
Study design
This large single-center retrospective cohort study included patients who underwent lung transplantation at the Guangzhou Institute of Respiratory Diseases between January 1, 2016, and July 31, 2021. Patients enrolled in this study had indications for lung transplantation after careful evaluation and were ≥18 years old. Patients with multiple organ transplantation or retransplantation, those who had received ECMO as a bridge to transplantation in the intensive care unit (ICU), those who received urgent intraoperative ECMO after receiving a lung transplant on one side, and those younger than 18 were excluded from this study.
Patients were excluded if their preoperative examination results were invalid or unavailable, or their perioperative medical records were incomplete. The data collector was blinded to the primary outcomes.
Ethical declaration
This study was approved by the Medical Ethics Committee of The First Affiliated Hospital of Guangzhou Medical University on December 14, 2021 (No. GYFYY-2021-K-45). The requirement for informed consent was waived because of its retrospective nature. The study was conducted in accordance with the Declaration of Helsinki (as revised in 2013).
Main procedures of anesthesia and surgery
In addition to routine monitoring, the radial artery was punctured to monitor the invasive arterial pressure. Anesthesia was induced sequentially using midazolam, sufentanil, etomidate, and rocuronium. A double-lumen bronchial catheter was inserted into the right place under fiberoptic bronchoscopy guidance. The tidal volume (VT), breath rate, and fraction of inspired oxygen (FiO2) were set at 6–8 mL/kg, 12–16 breaths/min, and 100% respectively. A central and pulmonary catheter was inserted into the internal jugular vein, and a pulse-indicating continuous cardiac output (PiCCO) catheter was inserted into the femoral artery.
Anesthesia was maintained bispectral index values between 40 and 60 with dexmedetomidine 0.5–1 µg/kg/h, propofol target-controlled infusion 2–3 µg/mL, and remifentanil 0.05–0.2 µg/kg/min.
Based on the preoperative clinical evaluation, the surgeon decides whether the patient will undergo single-lung or double-lung transplantation. After the primary lung was removed, the donor lungs were implanted. The trachea, pulmonary artery, and pulmonary vein were anastomosed. At the end of the surgery, the patient was transferred to the ICU after the double-lumen bronchial tube was replaced with a single-lumen tube.
Primary outcome and candidate predictors
Data of patients who underwent lung transplantation at our hospital were systematically reviewed. The primary outcome was the provision of ECMO support during lung transplantation. The criteria for intra-operative ECMO support were as follows: hemodynamic instability, severe hypoxemia (impaired gas exchange, hyperperfusion, and inability to tolerate single lung ventilation) after optimization of patient cardiopulmonary conditions, or a combination of the following conditions: hypercapnia [partial pressure of carbon dioxide in arterial blood (PaCO2) >60 mmHg, pH <7.2], arterial oxygen saturation (SaO2) <85%, and cardiac index (CI) levels <2 L/min/m2 (15).
The preoperative characteristics and post-anesthesia variables were included as the candidate predictors, including age, body mass index (BMI), sex, primary diagnosis, surgical type, the physical status of the American Society of Anesthesiologists, comorbidities, the result of pulmonary ventilation-perfusion scan, pro-B-type natriuretic peptide (pro-BNP), creatine kinase isoenzyme-MB (CKMB), cardiac troponin I, myocardial enzyme, left ventricular ejection fraction, the results of hepatic and renal function, hemocytes examination, procalcitonin concentration, and arterial blood gas analysis, the saturation of pulse oximetry, invasive mean arterial pressure, central venous pressure (CVP), heart rate, pulmonary artery systolic pressure (PASP), and peak airway pressure.
Statistical analysis
Data were analyzed using SAS version 9.4. All analyses were in line with the complete case input. All patients enrolled in the study were divided in a ratio of 7:3 to establish the development and validation datasets. According to the development datasets, risk and risk score models were created. A risk model was constructed following the univariate analysis with candidate predictors (P<0.05). The bootstrap method was used to select the best subset of risk factors. The scoring method of the risk-scoring model was based on the development a risk model that resembled the previous scoring method (19). Continuous variables were defined as clinically meaningful for scoring. The degree of correlation between the score level and the risk of using ECMO was assessed using Pearson’s contingency coefficient, and the trend was examined using the Cochran-Armitage test.
The prediction accuracy of the risk model and risk score model was assessed using C-statistics and calibrated using Hosmer-Lemeshow χ2 statistics and calibration plots. The split-sample method was used to verify the risk-scoring model and assess its stability. DeLong’s nonparametric method (20) was used to compare the area under the receiver operating characteristic (ROC) curve.
Results
This study enrolled 248 lung transplant patients (Figure 1).
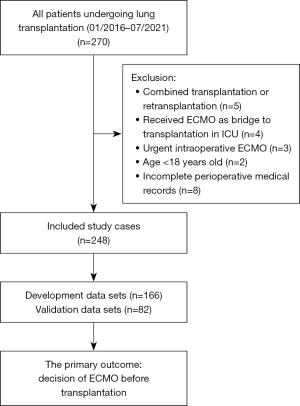
The development of the risk model and risk score
Of the enrolled 248 patients, 103 (41.5%) received intraoperative ECMO support. The development datasets included 166 patients, of whom 69 (41.6%) received intraoperative ECMO support, in contrast, the validation datasets included 82 patients, of whom 34 (41.5%) received intraoperative ECMO support. (Table S1). The Bootstrap technique was used to select variables in the developmental dataset. Primary disease diagnosis, PASP, sex, surgical type, CKMB, and pro-BNP were screened out to establish the risk model, the best subset of risk factors (Table 1). The risk scores for all predictive variables are shown in Table 2.
Table 1
Variables | Univariate analysis | Multivariate analysis | |||||
---|---|---|---|---|---|---|---|
OR | 95% CI | P value | OR | 95% CI | P value | ||
Primary disease diagnosis | |||||||
COPD | Ref | Ref | |||||
NSIP | 10.636 | 3.596–31.460 | <0.0001 | 6.045 | 1.773–20.617 | 0.004 | |
Silicosis | 9.000 | 1.918–42.235 | 0.005 | 6.399 | 0.976–41.934 | 0.053 | |
Bronchiectasis | 18.000 | 3.958–81.861 | <0.0001 | 3.207 | 0.521–19.764 | 0.209 | |
IPF | 14.250 | 4.409–46.052 | <0.0001 | 10.887 | 2.747–43.156 | 0.001 | |
Lymphangiomyoma | 6.000 | 1.501–23.990 | 0.011 | 1.468 | 0.243–8.872 | 0.676 | |
PASP | 1.057 | 1.027–1.088 | <0.0001 | 1.050 | 1.011–1.090 | 0.012 | |
Female vs. male | 4.227 | 1.726–10.353 | 0.002 | 5.905 | 1.582–22.040 | 0.008 | |
Surgical type | |||||||
Double-LTx | Ref | Ref | |||||
Right-LTx | 0.199 | 0.087–0.456 | <0.0001 | 0.215 | 0.074–0.625 | 0.005 | |
Left-LTx | 0.343 | 0.161–0.731 | 0.006 | 0.375 | 0.131–1.073 | 0.067 | |
Log CKMB† | 1.087 | 0.624–1.894 | 0.769 | 1.678 | 0.783–3.599 | 0.183 | |
Log pro-BNP† | 1.402 | 1.143–1.719 | 0.001 | 1.418 | 1.090–1.845 | 0.009 |
†, the natural logarithmic transformations of CKMB and pro-BNP were made because of their extreme positive skewness. ECMO, extracorporeal membrane oxygenation; OR, odds ratio; CI, confidence interval; COPD, chronic obstructive pulmonary disease; NSIP, nonspecific interstitial pneumonia; IPF, idiopathic pulmonary fibrosis; PASP, pulmonary artery systolic pressure; LTx, lung transplantation; CKMB, creatine kinase isoenzyme-MB; pro-BNP, pro-B-type natriuretic peptide.
Table 2
Risk factors | Score |
---|---|
Surgical type | |
Double-LTx | 6 |
Right-LTx | 0 |
Left-LTx | 2 |
Primary disease diagnosis | |
COPD | 0 |
NSIP | 7 |
Silicosis | 8 |
Bronchiectasis | 5 |
IPF | 10 |
Lymphangiomyoma | 2 |
Gender | |
Female | 7 |
Male | 0 |
PASP | |
≤30 mmHg | 0 |
31–50 mmHg | 4 |
51–70 mmHg | 8 |
>70 mmHg | 12 |
CKMB | |
≤12 U/L | 0 |
13–24 U/L | 1 |
>24 U/L | 2 |
Pro-BNP | |
≤125 pg/mL | 0 |
126–450 pg/mL | 1 |
451–900 pg/mL | 2 |
901–1,800 pg/mL | 3 |
>1,800 pg/mL | 4 |
LTx, lung transplantation; COPD, chronic obstructive pulmonary disease; NSIP, nonspecific interstitial pneumonia; IPF, idiopathic pulmonary fibrosis; PASP, pulmonary artery systolic pressure; CKMB, creatine kinase isoenzyme-MB; pro-BNP, pro-B-type natriuretic peptide.
Risk score validation
Based on the development data sets, the risk score model was used for discrimination, with a high χ2 statistic calibration of 5.624 (P=0.689) and a C-statistic of 0.850 [95% confidence interval (CI): 0.792–0.908] (Figure 2). When the risk score model was applied to the split sample from the validation datasets, the validation results were the same as those from the development datasets, accompanied by a high χ2 statistic calibration of 4.584 (P=0.801) and a C-statistic of 0.842 (95% CI: 0.759–0.924) (Figure 2). The ROC curves for the development and validation datasets were similar (Figure 2), and the percentage of intraoperative ECMO support use had no significant difference between the two groups.
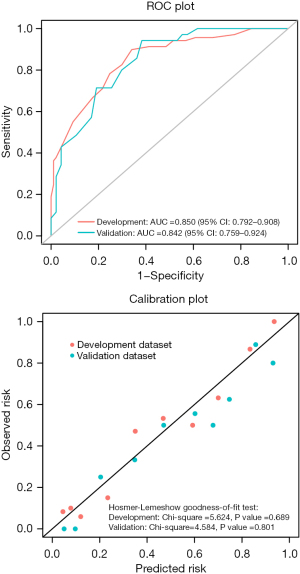
Clinical significance of the risk score model
According to the risk score model, the risk scores were divided into three levels suitable for clinical application according to the risk score model (Figures 3,4). Based on the score distribution of intraoperative ECMO use, three levels were classified as low-risk (0–10], moderate-risk (10–20], and high-risk (20–41] (Table S2), with total risk scores ranging from 0 (lowest risk) to 41 (highest risk) (Table 2). The associated predictions of intraoperative ECMO use ranged from 1.5% to 99.7%. The correlation between the risk level and predicted risk probability was successively defined as low-risk (1.5–12.2%), moderate-risk (15.1–62.2%), and high-risk (67.8–99.7%) (Table 3).
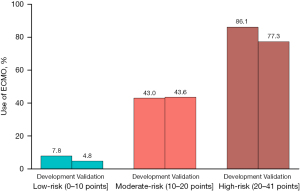
Table 3
Risk level | Risk score level (points) | Predicted risk (%) |
---|---|---|
Low-risk | (0–10] | 1.5–12.2 |
Moderate-risk | (10–20] | 15.1–62.2 |
High-risk | (20–41] | 67.8–99.7 |
ECMO, extracorporeal membrane oxygenation.
Discussion
Key findings
ECMO can provide cardiopulmonary support to patients with heart and respiratory failures through external gas exchange and power pumps (6,8,21). Intraoperative ECMO can overcome right heart failure, excessive pulmonary hypertension or hypercapnia, and global hypoxia during one-lung ventilation, effectively improving the oxygenation state of the body, maintaining the systemic blood supply and hemodynamics in a relatively stable state, improving the success rate of lung transplantation, and improving the prognosis of lung transplant recipient (22-25). Our institute is one of China’s largest lung transplantation centers (26).
However, when considering the implementation of ECMO during lung transplantation, the judgment is frequently based on previous experience. Therefore, there is an urgent need to establish a scientific evaluation model for clinical decision-making. This study established and validated a risk score model according to six available factors (primary disease diagnosis, PASP, sex, surgical type, CKMB, and pro-BNP levels) that can predict the need for intraoperative ECMO before lung transplant. We classified low-, moderate-, and high-risk levels that clinicians can easily consult.
This study aimed to predict the need for intraoperative ECMO in patients undergoing lung transplantation based on preoperative characteristics and variables after anesthesia. This simple and effective risk score can immediately identify high-risk patients and grade their risk, ultimately prompting clinicians to initiate early treatment.
Strengths and limitations
This study had some limitations. Firstly, the number of patients undergoing lung transplantation was relatively small. Because of the insufficient number of donor lung organs, it was impossible to obtain a large sample size. Second, this retrospective study was conducted at a single center, which might have led to some bias. Although clinical practice between different transplant centers influences the administration of ECMO due to different policies or preferences, our transplantation center implemented transplantation according to the guidelines of the International Society for Heart and Lung Transplantation; therefore, the risk model is still adaptable to other centers. Thirdly, a split-sample method was used to verify the model. This is an easy method to test for overfitting without ensuring the generality of the individual queues. Fourth, pulmonary function values were not included in the analysis due to the many patients with preoperative end-stage lung disease, leading to failure to complete the pulmonary function examination. If they had been included in the analysis, many patients without pulmonary function examinations might have been excluded, leading to deviations in the results. Pulmonary function was assessed by arterial blood gas analysis instead of a pulmonary function test.
Comparison with similar researches
ECMO is a reliable and effective strategy for supporting lung function; however, severe PGD significantly impacts early and late outcomes (27). The timing of intraoperative ECMO may also affect postoperative outcomes. Fessler et al. reported that patients who received emergency intraoperative ECMO support due to complications had a worse prognosis than those who did not need it (28). ECMO can improve survival when used preemptively at the start of transplantation in patients with significant extracorporeal life support needs and surgically in patients with cardiopulmonary instability following cross-clapping of the pulmonary artery.
Vienna found that intraoperative ECMO-supported lung transplantation had a higher survival rate than transplants without intraoperative support, and recommended that ECMO can be extended until the early period after surgery (29). Hoetzenecker et al. suggested that routine intraoperative ECMO could reduce the incidence of primary PGD and lead to good graft function and interim outcomes in lung transplant recipients (30).
In addition, it has been found that recipients requiring ECMO support during lung transplantation tend to have more serious pulmonary hypertension (31,32). Patients with severe pulmonary hypertension are at high risk of pulmonary reperfusion edema in the early post-transplantation period due to acute right heart failure caused by an increased right heart load during surgery. CKMB and pro-BNP are commonly used indices to evaluate cardiac function, the higher the values, the higher the possibility of cardiac insufficiency. In lung transplant patients, increased CVP caused by cardiac insufficiency can lead to increased blood perfusion pressure, increased risk of pulmonary edema, postoperative renal failure, and failure of other failures.
Increased CVP can, in turn, aggravate pulmonary vascular resistance, cause interstitial edema, further increase airway resistance, decrease SaO2, and form a hemodynamic vicious circle. ECMO-assisted diversion can effectively reduce pulmonary hypertension and blood perfusion pressure in new lungs, stabilize patients’ blood circulation system, and reduce perioperative cardiac failure (29,33,34). Salman reported that routine prophylactic application of ECMO support during lung transplantation for patients with primary pulmonary hypertension can reduce perioperative mortality and improve cardiac function recovery 1 year after surgery (24).
Patients receiving ECMO support may have higher mortality rates. Bermudez retrospectively assessed and reported that the 1-year survival rate of patients with and without ECMO support was 80.9% and 86.1% respectively (35). Munich reported 11.1% perioperative mortality and 81.5% 1-year survival rate with ECMO support, compared to 4.5% and 81.8% without ECMO support, respectively (36). Ius reported that the overall survival rates at 1- and 4-year were 83% and 68% for patients who underwent ECMO preoperatively, 82% and 69% when ECMO was administered intraoperatively, and 93% and 73% for those who did not receive ECMO (37).
However, several other retrospective single-center observational studies have shown that the survival rates of patients receiving ECMO-bridging transplantation are comparable to those of patients who do not (38-40). A systematic review found that the survival rate of patients supported by ECMO varied from 10% to 50% after surgery, and from 50% to 90% 1 year after transplantation (41). The success rate of ECMO at different stages highlights the need for careful patient selection, especially considering the potential role of disease processes on prognosis (42).
Explanations of findings
In this study, the recommended risk scores showed a high level of variability and good calibration in the development and validation datasets, and the risk scores were more accurate in predicting the need for intraoperative ECMO. The risk score model established in this study, combined with the six preoperative and operative factors, has a more comprehensive prediction ability, which can provide more scientific guidance for the clinical decision to use ECMO. At low-risk (score ≤10), patients can complete lung transplantation without ECMO, perioperative management becomes simple, ECMO-related complications can be avoided, medical resources can be saved, and the economic burden of patients can be reduced.
The risk level is moderate if the score is between 10–20. In this case, more real-time clinical information, such as patient volume status, degree of hemodynamic fluctuation, amplitude of pulmonary artery pressure increase when clapping pulmonary artery, and oxygenation during single-lung ventilation, should be combined to evaluate whether patients should use ECMO. The risk level is high when the score is >20 points.
Implications and actions needed
Patients undergoing lung transplantation are advised to receive ECMO before surgery to improve their overall survival or even use ECMO bridging transplantation (43,44). There is no unified evaluation index for intraoperative ECMO support, and most centers use this index based on their own experiences according to the patient’s preoperative and postoperative conditions. Establishing this risk-scoring model is an auxiliary method to further support decisions based on experience. This would be a useful tool to avoid unnecessary intraoperative ECMO, which is costly and carries the risk of complications, and to avoid urgent intraoperative cannulations by identifying patients requiring extracorporeal life support during transplantation.
Conclusions
This simple risk-scoring model, established based on six available factors (primary disease diagnosis, PASP, sex, surgical type, CKMB, and pro-BNP), effectively predicts the need for intraoperative ECMO. This can help clinicians discriminate between high-, moderate-, and low-risk patients and perform appropriate early interventions while optimizing the allocation of healthcare resources.
Acknowledgments
We are grateful for the help of Dr. Yinfen Li and Dr. Qinglong Dong for the support of clinical data collection and for the contributions of all the participants in this observational study.
Funding: None.
Footnote
Reporting Checklist: The authors have completed the TRIPOD reporting checklist. Available at https://jtd.amegroups.com/article/view/10.21037/jtd-23-452/rc
Data Sharing Statement: Available at https://jtd.amegroups.com/article/view/10.21037/jtd-23-452/dss
Peer Review File: Available at https://jtd.amegroups.com/article/view/10.21037/jtd-23-452/prf
Conflicts of Interest: All authors have completed the ICMJE uniform disclosure form (available at https://jtd.amegroups.com/article/view/10.21037/jtd-23-452/coif). The authors have no conflicts of interest to declare.
Ethical Statement: The authors are accountable for all aspects of the work in ensuring that questions related to the accuracy or integrity of any part of the work are appropriately investigated and resolved. The study was conducted in accordance with the Declaration of Helsinki (as revised in 2013). This study was approved by the Medical Ethics Committee of The First Affiliated Hospital of Guangzhou Medical University on December 14, 2021 (No. GYFYY-2021-K-45). The requirement for informed consent was waived because of its retrospective nature.
Open Access Statement: This is an Open Access article distributed in accordance with the Creative Commons Attribution-NonCommercial-NoDerivs 4.0 International License (CC BY-NC-ND 4.0), which permits the non-commercial replication and distribution of the article with the strict provision that no changes or edits are made and the original work is properly cited (including links to both the formal publication through the relevant DOI and the license). See: https://creativecommons.org/licenses/by-nc-nd/4.0/.
References
- Hardy JD, Webb WR, Dalton ML Jr, et al. Lung homotransplantation in man. JAMA 1963;186:1065-74. [Crossref] [PubMed]
- Goldberg M, Lima O, Morgan E, et al. A comparison between cyclosporin A and methylprednisolone plus azathioprine on bronchial healing following canine lung autotransplantation. J Thorac Cardiovasc Surg 1983;85:821-6.
- Chambers DC, Cherikh WS, Goldfarb SB, et al. The International Thoracic Organ Transplant Registry of the International Society for Heart and Lung Transplantation: Thirty-fifth adult lung and heart-lung transplant report-2018; Focus theme: Multiorgan Transplantation. J Heart Lung Transplant 2018;37:1169-83. [Crossref] [PubMed]
- Kirklin JK, Cantor R, Mohacsi P, et al. First Annual IMACS Report: A global International Society for Heart and Lung Transplantation Registry for Mechanical Circulatory Support. J Heart Lung Transplant 2016;35:407-12. [Crossref] [PubMed]
- Ostadal P, Rokyta R, Karasek J, et al. Extracorporeal Membrane Oxygenation in the Therapy of Cardiogenic Shock: Results of the ECMO-CS Randomized Clinical Trial. Circulation 2023;147:454-64. [Crossref] [PubMed]
- Hill JD, O'Brien TG, Murray JJ, et al. Prolonged extracorporeal oxygenation for acute post-traumatic respiratory failure (shock-lung syndrome). Use of the Bramson membrane lung. N Engl J Med 1972;286:629-34. [Crossref] [PubMed]
- Australia and New Zealand Extracorporeal Membrane Oxygenation (ANZ ECMO) Influenza Investigators. Extracorporeal Membrane Oxygenation for 2009 Influenza A(H1N1) Acute Respiratory Distress Syndrome. JAMA 2009;302:1888-95. [Crossref] [PubMed]
- Betit P. Technical Advances in the Field of ECMO. Respir Care 2018;63:1162-73. [Crossref] [PubMed]
- Supady A, Combes A, Barbaro RP, et al. Respiratory indications for ECMO: focus on COVID-19. Intensive Care Med 2022;48:1326-37. [Crossref] [PubMed]
- Lozano-Espinosa M, Antolín-Amérigo D, Riera J, et al. Extracorporeal membrane oxygenation (ECMO) and beyond in near fatal asthma: A comprehensive review. Respir Med 2023;215:107246. [Crossref] [PubMed]
- Elmaleh Y, De Tymowski C, Zappella N, et al. Blood transfusion of the donor is associated with stage 3 primary graft dysfunction after lung transplantation. Clin Transplant 2021;35:e14407. [Crossref] [PubMed]
- Seay T, Guinn N, Maisonave Y, et al. The Association of Increased FFP:RBC Transfusion Ratio to Primary Graft Dysfunction in Bleeding Lung Transplantation Patients. J Cardiothorac Vasc Anesth 2020;34:3024-32. [Crossref] [PubMed]
- Zhao CY, Sheng KJ, Bao T, et al. Commercial and novel anticoagulant ECMO coatings: a review. J Mater Chem B 2023;11:4832-41. [Crossref] [PubMed]
- Bittner HB, Binner C, Lehmann S, et al. Replacing cardiopulmonary bypass with extracorporeal membrane oxygenation in lung transplantation operations. Eur J Cardiothorac Surg 2007;31:462-7; discussion 467. [Crossref] [PubMed]
- Brogan TV, Thiagarajan RR, Rycus PT, et al. Extracorporeal membrane oxygenation in adults with severe respiratory failure: a multi-center database. Intensive Care Med 2009;35:2105-14. [Crossref] [PubMed]
- Scaravilli V, Fumagalli J, Rosso L, et al. Heparin-Free Lung Transplantation on Venovenous Extracorporeal Membrane Oxygenation Bridge. ASAIO J 2021;67:e191-7. [Crossref] [PubMed]
- Hayanga JWA, Shigemura N, Aboagye JK, et al. ECMO Support in Lung Transplantation: A Contemporary Analysis of Hospital Charges in the United States. Ann Thorac Surg 2017;104:1033-9. [Crossref] [PubMed]
- George TJ, Beaty CA, Kilic A, et al. Outcomes and temporal trends among high-risk patients after lung transplantation in the United States. J Heart Lung Transplant 2012;31:1182-91. [Crossref] [PubMed]
- Sullivan LM, Massaro JM, D'Agostino RB Sr. Presentation of multivariate data for clinical use: The Framingham Study risk score functions. Stat Med 2004;23:1631-60. [Crossref] [PubMed]
- DeLong ER, DeLong DM, Clarke-Pearson DL. Comparing the areas under two or more correlated receiver operating characteristic curves: a nonparametric approach. Biometrics 1988;44:837-45.
- Salna M, Bacchetta M. Extracorporeal lung support. Curr Opin Anaesthesiol 2017;30:50-7. [Crossref] [PubMed]
- Napp LC, Kühn C, Bauersachs J. ECMO in cardiac arrest and cardiogenic shock. Herz 2017;42:27-44. [Crossref] [PubMed]
- Butt W, MacLaren G. Extracorporeal membrane oxygenation 2016: an update. F1000Res 2016;5:F1000 Faculty Rev-750.
- Salman J, Ius F, Sommer W, et al. Mid-term results of bilateral lung transplant with postoperatively extended intraoperative extracorporeal membrane oxygenation for severe pulmonary hypertension. Eur J Cardiothorac Surg 2017;52:163-70. [Crossref] [PubMed]
- McFadden PM, Greene CL. The evolution of intraoperative support in lung transplantation: Cardiopulmonary bypass to extracorporeal membrane oxygenation. J Thorac Cardiovasc Surg 2015;149:1158-60. [Crossref] [PubMed]
- Zhang R, Xu Y, Sang L, et al. Factors associated with intraoperative extracorporeal membrane oxygenation support during lung transplantation. Respir Res 2020;21:85. [Crossref] [PubMed]
- Boffini M, Simonato E, Ricci D, et al. Extracorporeal membrane oxygenation after lung transplantation: risk factors and outcomes analysis. Ann Cardiothorac Surg 2019;8:54-61. [Crossref] [PubMed]
- Fessler J, Sage E, Roux A, et al. Is Extracorporeal Membrane Oxygenation Withdrawal a Safe Option After Double-Lung Transplantation? Ann Thorac Surg 2020;110:1167-74. [Crossref] [PubMed]
- Hoetzenecker K, Schwarz S, Muckenhuber M, et al. Intraoperative extracorporeal membrane oxygenation and the possibility of postoperative prolongation improve survival in bilateral lung transplantation. J Thorac Cardiovasc Surg 2018;155:2193-2206.e3. [Crossref] [PubMed]
- Hoetzenecker K, Benazzo A, Stork T, et al. Bilateral lung transplantation on intraoperative extracorporeal membrane oxygenator: An observational study. J Thorac Cardiovasc Surg 2020;160:320-327.e1. [Crossref] [PubMed]
- Fang A, Studer S, Kawut SM, et al. Elevated pulmonary artery pressure is a risk factor for primary graft dysfunction following lung transplantation for idiopathic pulmonary fibrosis. Chest 2011;139:782-7. [Crossref] [PubMed]
- Whelan TP, Dunitz JM, Kelly RF, et al. Effect of preoperative pulmonary artery pressure on early survival after lung transplantation for idiopathic pulmonary fibrosis. J Heart Lung Transplant 2005;24:1269-74. [Crossref] [PubMed]
- Sultan S, Tseng S, Stanziola AA, et al. Pulmonary Hypertension: The Role of Lung Transplantation. Heart Fail Clin 2018;14:327-31. [Crossref] [PubMed]
- Welker CC, Huang J, Boswell MR, et al. Left Ventricular Decompression in VA-ECMO: Analysis of Techniques and Outcomes. J Cardiothorac Vasc Anesth 2022;36:4192-7. [Crossref] [PubMed]
- Bermudez CA, Shiose A, Esper SA, et al. Outcomes of intraoperative venoarterial extracorporeal membrane oxygenation versus cardiopulmonary bypass during lung transplantation. Ann Thorac Surg 2014;98:1936-42; discussion 1942-3. [Crossref] [PubMed]
- Hoechter DJ, von Dossow V, Winter HThe Munich Lung Transplant Group, et al. Intraoperative Extracorporeal Circulation in Lung Transplantation. Thorac Cardiovasc Surg 2015;63:706-14. [Crossref] [PubMed]
- Ius F, Sommer W, Tudorache I, et al. Five-year experience with intraoperative extracorporeal membrane oxygenation in lung transplantation: Indications and midterm results. J Heart Lung Transplant 2016;35:49-58. [Crossref] [PubMed]
- Javidfar J, Brodie D, Iribarne A, et al. Extracorporeal membrane oxygenation as a bridge to lung transplantation and recovery. J Thorac Cardiovasc Surg 2012;144:716-21. [Crossref] [PubMed]
- Dellgren G, Riise GC, Swärd K, et al. Extracorporeal membrane oxygenation as a bridge to lung transplantation: a long-term study. Eur J Cardiothorac Surg 2015;47:95-100; discussion 100. [Crossref] [PubMed]
- Mohite PN, Sabashnikov A, Reed A, et al. Extracorporeal Life Support in "Awake" Patients as a Bridge to Lung Transplant. Thorac Cardiovasc Surg 2015;63:699-705. [Crossref] [PubMed]
- Chiumello D, Coppola S, Froio S, et al. Extracorporeal life support as bridge to lung transplantation: a systematic review. Crit Care 2015;19:19. [Crossref] [PubMed]
- Abrams D, Brodie D, Arcasoy SM. Extracorporeal Life Support in Lung Transplantation. Clin Chest Med 2017;38:655-66. [Crossref] [PubMed]
- Bharat A, Machuca TN, Querrey M, et al. Early outcomes after lung transplantation for severe COVID-19: a series of the first consecutive cases from four countries. Lancet Respir Med 2021;9:487-97. [Crossref] [PubMed]
- Kurihara C, Manerikar A, Querrey M, et al. Clinical Characteristics and Outcomes of Patients With COVID-19-Associated Acute Respiratory Distress Syndrome Who Underwent Lung Transplant. JAMA 2022;327:652-61. [Crossref] [PubMed]