Association between regulatory T cells and ischemic heart disease: a Mendelian randomization study
Highlight box
Key findings
• Elevated CD28 expression on CD4 regulatory T cells (Tregs) may be a protective factor against ischemic heart disease (IHD).
What is known, and what is new?
• The dysfunction of Tregs leads to inflammation and unhealthy aging, thereby promoting the formation of atherosclerotic plaques and accelerating their progression.
• Elevated CD28 expression on CD4 Tregs may serve as a novel molecular modifier and influence the occurrence of IHD.
What is the implication, and what should change now?
• This finding can advance our understanding of the immunopathology underlying IHD and accelerate the future development of cell-based therapies targeting the disease.
Introduction
Ischemic heart disease (IHD) is the leading contributor to the global burden of cardiovascular disease, which is primarily caused by coronary heart disease (CHD) (1,2). In IHD, coronary artery stenosis or occlusion leads to reduced myocardial blood perfusion, resulting in a series of clinical ischemic symptoms (3). With advancing age, the prevalence of IHD significantly increases, as it is an aging-related disease (4).
Aging-related diseases usually manifest as a sustained inflammatory response that ultimately disrupts tissue homeostasis (5). This inflammatory response is a crucial component in the development of IHD, involving both innate and acquired immune responses (6). T cell subsets play a regulatory role in atherosclerotic plaque formation and maintenance (6). Notably, there is growing interest in the function of regulatory T cells (Tregs) in IHD (7,8). Tregs are inhibitory CD4+ T cells, mainly regulated by forkhead box P3 (Foxp3) expression. Tregs act as regulatory cells whose dysfunction leads to inflammation and unhealthy aging, which triggers the promotion of atherosclerotic plaque formation and accelerates its progression (8-10). However, further investigation is required to determine the relationship between different Treg subtypes—each with distinct differentiation characteristics—and IHD, and clarifying the direction of their causal interaction may be highly beneficial.
Mendelian randomization (MR) employs genetic variants as proxies for exposure to determine the causal impact of those exposures on the outcome of interest (11-13). In this way, MR overcomes previous research limitations and is thus highly suited to determining the role and causal relationship between Tregs and IHD.
Therefore, this study aims to conduct a two-sample MR study using two different genome-wide association studies (GWAS) to further explore the causal association between molecular characteristics in peripheral Treg and IHD risk. Through this study, we hope to gain a deeper understanding of the role of Treg-related molecules in the pathogenesis of IHD and provide new ideas and methods for the prevention and treatment of IHD. We present this article in accordance with the STROBE-MR (Strengthening the Reporting of Observational Studies in Epidemiology Using Mendelian Randomization) reporting checklist (14) (available at https://jtd.amegroups.com/article/view/10.21037/jtd-23-1790/rc).
Methods
Study design
We conducted a two-sample bidirectional MR study to assess the cause–effect interaction between 51 Treg subtypes and IHD. As shown in Figure 1, there are three major assumptions that the MR approach needs to meet: (I) the association hypothesis, that is, single-nucleotide polymorphisms (SNPs) are robustly related to exposure; (II) the independence hypothesis, in which SNPs should be independent of confounding factors; and (III) the exclusivity hypothesis, in which SNPs are not associated with outcome (15). The study was conducted in accordance with the Declaration of Helsinki (as revised in 2013).
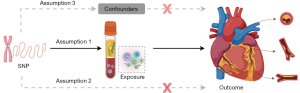
Data source
We retrieved the data from studies that provide publicly accessible summary GWAS data. The comprehensive Treg subtypes (refer to table available at https://cdn.amegroups.cn/static/public/jtd-23-974-1.xlsx) were obtained from the SardiNIA study, which consists of GWAS data collected from 3,757 individuals (57% female) representing the general population residing on the central east coast of Sardinia, Italy (16). This cohort included 118 absolute cell counts, 389 MFIs of surface antigens, 32 morphological parameters, and 192 relative counts (ratios between cell levels), amounting to a total of 731 cell traits evaluated in this population. This data contains expression levels of cellular markers for different Treg subtypes, while the phenotype ascertainment method involves antibody staining of peripheral blood from normal individuals and flow cytometry processing. Tregs were distinguished by their elevated levels of CD25 and diminished expression of CD127 surface antigens. They were further subclassified into activated, resting, and secreting subpopulations based on CD45RA expression. Moreover, CD8 T cells in this cohort were categorized based on the expression of CD28 and CD45RA antigens. The elevated levels of CD25 and the lack of CD127 on CD28 negative CD8 cells were also examined. Finally, Treg subsets, alongside CD4+ and CD8+ T cells, underwent further stratification according to their expression of CD39. The GWAS summary-level data for IHD were sourced from the IEU open GWAS project (https://gwas.mrcieu.ac.uk/datasets/finn-b-I9_ISCHHEART), which contains 30,952 cases and 187,845 controls, all of which are European ancestry. IHD is described as coronary thrombosis, which is “the coagulation of blood in any of the coronary vessels”. The presence of a blood thrombus usually leads to myocardial infarction.
Instrumental variable (IV) selection
To determine qualified instrumental SNPs, we conducted a comprehensive set of quality control measures to identify the most impartial and representative instrumental genetic variables. First, the genome-wide significance threshold for potential SNPs was set to P<5.00E−08. Next, we conducted a clumping procedure (using a threshold of r2<0.001 and a window width of 10,000 kb) to identify independent SNPs, employing European reference samples obtained from the 1000 Genomes Project for this filtration step. Thirdly, we extracted exposure SNPs from the outcome GWAS summary data. In cases where a special exposure SNP was absent in the outcome GWAS, we substituted it with a proxy SNP that possessed linkage disequilibrium (LD) to the exposure SNP (with a minimum LD r2 value of 0.8). The next step involved harmonizing the exposure SNPs and outcome SNPs, while excluding ambiguous SNPs whose effect allele could not be determined. Palindromic SNPs were explicitly examined in the original datasets to avoid any unintended reverse effects. Additionally, we computed the F-statistic for each Treg characteristic, and only instrumental SNPs with an F-statistic greater than ten were considered qualified. The F-statistic was derived using the following formula: F = R2(n − k − 1)/k(1 − R2), where R2 represents the exposure variation elucidated by the instrumental SNPs, n stands for the sample size, and k denotes the number of IVs (17). These rigorously chosen SNPs served as IVs in the subsequent analysis.
Analysis of two-sample MR
We employed multiple MR methods to assess the causal effects of exposure variables on the outcome. When only one instrumental SNP was accessible, we utilized the Wald ratio method. However, when multiple SNPs were present, we opted for the inverse variance-weighted (IVW) method, which is commonly used in meta-analysis of the SNP-specific Wald estimates while assuming balanced pleiotropy. All causal estimates are displayed as odds ratios (OR) alongside 95% confidence intervals (CIs) for the dichotomous phenotype outcome. If exposures had more than three SNPs accessible, we conducted sensitivity analyses using alternative MR methods, albeit at the expense of reduced statistical power. These methods included weight median (18), weighted mode (19), simple mode, MR Egger regression (20), and Mendelian Randomization Pleiotropy Residual Sum and Outlier (MR-PRESSO) (21). In order to verify the validity of the assumption that exposure causes outcomes, we also conducted a reverse MR analysis. Finally, the statistical power of each exposure was calculated with a bilateral type-I error rate of α=0.05 (22).
Statistical analysis
All statistical analysis was performed with the “TwoSampleMR” package for MR analyses in R version 4.2.2 (The R Foundation for Statistical Computing, Vienna, Austria) (23). The P values as exposures (Treg subtypes) were adjusted using a false-discovery rate (FDR) method due to repetitive comparisons with the outcome (IHD). Statistical significance was determined by an FDR-corrected P value <0.05, indicating robust causal evidence.
Results
Selection of IVs
According to the above selection criteria, a total of 197 SNPs were selected as IVs associated with 51 Treg subtypes. The detailed characteristics of the instrumental SNPs are shown in table available at https://cdn.amegroups.cn/static/public/jtd-23-974-1.xlsx. Table available at https://cdn.amegroups.cn/static/public/jtd-23-974-2.xlsx summarizes the results of the MR study and sensitivity analysis for all the subtypes.
Two-sample MR analysis of Tregs and IHD
As seen in Table 1 and Figure 2, 13 significant variables, including CD28 on activated & secreting CD4 Tregs, CD28 on activated CD4 Tregs, CD28 on CD4 Tregs, CD28 on resting CD4 Tregs, CD25 on CD39+ CD4 Tregs, CD25 on activated CD39+ CD4 Tregs, CD4 on CD4 Tregs, CD4 on resting CD4 Tregs, CD4 on activated CD4 Tregs, CD4 on secreting CD4 Tregs, CD4 on activated and secreting CD4 Tregs, CD25 on secreting CD4 Tregs, and CD25 on secreting CD39+ CD4 Tregs, were found to be potentially associated with IHD (all P values <0.05). None of these exposures had instrumental SNPs exceeding 2 in number. Therefore, we use the Wald ratio or IVW methods for the MR analysis without employing proxy SNPs. All selected instrumental variants showed strong F-statistics (range, 34.01–272.99, median 51.89) with the exposure, as shown in Table 1. After FDR adjustment, we identified 4 Treg subtypes that exhibited protective effects against IHD occurrence (CD28 on activated & secreting CD4 Tregs [odds ratio (OR) =0.89; 95% confidence interval (CI): 0.82–0.96; P=3.10E−03; adjusted P=0.04], CD28 on activated CD4 Tregs (OR =0.87; 95% CI: 0.80–0.95; P=3.10E−03; adjusted P=0.04), CD28 on CD4 Tregs (OR =0.87; 95% CI: 0.80–0.96; P=3.41E−03; adjusted P=0.04), CD28 on resting CD4 Treg cell (OR =0.91; 95% CI: 0.85–0.97; P=3.48E−03; adjusted P=0.04).
Table 1
Exposure | Method | No. of SNPs | P value | OR (95% CI) | Adjusted P value | F |
---|---|---|---|---|---|---|
CD28 on activated & secreting CD4 Tregs | Wald ratio | 1 | 3.10E−03 | 0.89 (0.82, 0.96) | 0.04 | 162.82 |
CD28 on activated CD4 Tregs | Wald ratio | 1 | 3.10E−03 | 0.87 (0.80, 0.95) | 0.04 | 124.61 |
CD28 on CD4 Tregs | Wald ratio | 1 | 3.41E−03 | 0.87 (0.80, 0.96) | 0.04 | 121.11 |
CD28 on resting CD4 Tregs | Wald ratio | 1 | 3.48E−03 | 0.91 (0.85, 0.97) | 0.04 | 272.99 |
CD25 on CD39+ CD4 Tregs | Wald ratio | 1 | 8.42E−03 | 1.18 (1.04, 1.34) | 0.07 | 51.89 |
CD25 on activated CD39+ CD4 Tregs | Wald ratio | 1 | 8.42E−03 | 1.19 (1.05, 1.36) | 0.07 | 45.95 |
CD4 on CD4 Tregs | Wald ratio | 1 | 2.47E−02 | 0.89 (0.81, 0.99) | 0.11 | 64.75 |
CD4 on resting CD4 Tregs | Wald ratio | 1 | 2.47E−02 | 0.86 (0.76, 0.98) | 0.11 | 37.46 |
CD4 on activated CD4 Tregs | Wald ratio | 1 | 2.47E−02 | 0.90 (0.81, 0.99) | 0.11 | 68.19 |
CD4 on secreting CD4 Tregs | Wald ratio | 1 | 2.47E−02 | 0.87 (0.78, 0.98) | 0.11 | 46.11 |
CD4 on activated and secreting CD4 Tregs | Wald ratio | 1 | 2.47E−02 | 0.89 (0.81, 0.99) | 0.11 | 62.04 |
CD25 on secreting CD4 Tregs | IVW | 2 | 3.12E−02 | 1.08 (1.01, 1.17) | 0.13 | 43.79 |
CD25 on secreting CD39+ CD4 Tregs | IVW | 2 | 3.41E−02 | 1.09 (1.01, 1.17) | 0.13 | 38.53 |
MR, Mendelian randomization; Treg, regulatory T cell; SNP, single-nucleotide polymorphism; OR, odds ratio; CI, confidence interval; IVW, inverse variance-weighted.
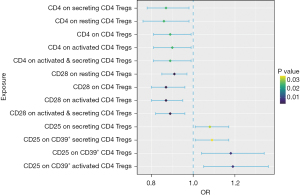
Reverse MR analysis
Reverse MR analysis identified eight significant variables (Table 2). However, these associations were nonsignificant after the FDR correction was applied (all adjusted P values >0.05). The detailed reverse MR results are shown in table available at https://cdn.amegroups.cn/static/public/jtd-23-974-3.xlsx and table available at https://cdn.amegroups.cn/static/public/jtd-23-974-4.xlsx.
Table 2
Outcome | No. of SNPs | P value | OR (95% CI) | Adjusted P value |
---|---|---|---|---|
CD4 on secreting CD4 Tregs | 26 | 3.77E−03 | 1.20 (1.06, 1.35) | 0.08 |
CD4 on activated CD39+ Tregs | 26 | 4.17E−03 | 1.21 (1.06, 1.37) | 0.08 |
CD4 on activated and secreting CD4 Tregs | 26 | 5.39E−03 | 1.21 (1.06, 1.38) | 0.08 |
CD39+ on secreting CD4 Tregs | 26 | 7.27E−03 | 0.85 (0.76, 0.96) | 0.08 |
CD4 on CD4 Tregs | 26 | 8.34E−03 | 1.20 (1.05, 1.38) | 0.08 |
CD4 on activated CD4 Tregs | 26 | 9.05E−03 | 1.20 (1.05, 1.38) | 0.08 |
CD39+ on secreting CD4 Tregs | 26 | 2.88E−02 | 0.88 (0.79, 0.99) | 0.21 |
CD39+ on activated CD4 Tregs | 26 | 4.13E−02 | 0.88 (0.77, 0.99) | 0.26 |
MR, Mendelian randomization; IHD, ischemic heart disease; Treg, regulatory T cell; IVW, inverse variance-weighted; SNP, single-nucleotide polymorphism; OR, odds ratio; CI, confidence interval.
Discussion
IHD is primarily associated with diseases caused by metabolic disorders, such as hypertension, hyperlipidemia, hyperglycemia, and obesity (24). Currently, pharmaceutical agents and surgical intervention are the main treatment options for IHD (25). Stem cells and stem cell-derived exosomes have been widely used over decades in the treatment research of IHD, and it has been confirmed that cell therapy can improve patients’ cardiac condition (26-28). In addition, immune cells and inflammatory cytokine production play a crucial role in the development of IHD. As promising therapeutic targets, Tregs have garnered increasing attention due to their ability to suppress innate and adaptive immune responses, mitigate excessive inflammation, and promote tissue repair following myocardial ischemia. As the first bidirectional MR analysis of IHD and Tregs, our study clarified the causal relationships between IHD and 51 Tregs with variable differentiation characteristics. Using genetic variants as IVs, MR analysis allows for establishing causal inferences concerning the impacts of modifiable risk factors, thereby helping to address specific types of confounding (15).
Our analysis extensively assessed the causal relationship between Treg traits and IHD, and four protective factors (high expression of CD28 on CD4 Tregs, including total, activated & secreting, activated and resting CD4 Tregs) were identified in our study. CD28, a crucial molecule for T cell activation, is expressed on T cells and binds to the antigen-presenting cells’ CD80 and CD86 molecules. This interaction plays a vital role in facilitating T cell activation. This finding matched the observations of earlier studies. For instance, experimental studies conducted on mice have demonstrated that Tregs can mitigate the development of atherosclerosis, enhance plaque vulnerability, and ameliorate acute ischemic myocardial injury (29-34). Conversely, depletion of Tregs has been shown to promote atherosclerosis progression, impede plaque remodeling and contraction, and disrupt the resolution of inflammation (35,36). Previous research has indicated that reduced CD28 levels on specific subsets of T cells are linked to an increased likelihood of developing inflammatory bowel disease but may exert a protective effect against multiple sclerosis (37-43). Moreover, reduced CD28 expression on resting Tregs increases susceptibility to primary biliary cholangitis (44). However, whether Tregs with high CD28 expression can reduce the risk of IHD has not been clarified and requires further investigation.
Although ischemic myocardial injury can be mitigated by suppressing inflammatory responses of specific immune cells, it remains a challenging therapeutic task due to the various context-dependent functional consequences of different immune cells. Several studies have proposed that dysfunctional and proinflammatory Tregs may contribute to adverse cardiac remodeling in patients with IHD and chronic heart failure (45,46). In our study, we identified Tregs with high CD25 expression as a potential risk factor for IHD (P<0.05; adjusted P value >0.05) (Table 1).
Previous experimental results have also demonstrated that atherosclerosis can disrupt the regulation of Tregs, thereby exacerbating the progression of atherosclerosis (8,46,47). After an ischemic injury occurs, immune cells infiltrate the affected tissues and release inflammatory cytokines, collectively establishing an inflammatory network that plays a role in the continuation of IHD (48). Although the adjusted P value did not reach statistical significance in our study, our reverse MR results suggested that IHD might alter the abundance of different molecular features of Tregs in peripheral blood, such as secreting and activated induced CD39+ CD4 Tregs or Tregs with higher CD4 expression (P<0.05; adjusted P>0.05).
Although the treatments of IHD have been significantly improved, the incidence of IHD is consistently rising due to multiple factors; including the increment of the aged population, changes in lifestyle, and the improvement of survivability in patients with CHD. Furthermore, in patients who are not effectively treated, e.g., patients with complex coronary lesions, advanced age, and a greater number of comorbidities, the novel treatment is in urgent need to reach a better outcome. Extensive research has been conducted regarding the genetic foundations of T cell functions and subtypes. This has led to significant advancements in genetically engineered T cell immunotherapies that have demonstrated remarkable clinical efficacy (49). In the context of ischemic injury, Tregs play a vital role in promoting tissue repair by inhibiting the activity of various immune cell types to prevent excessive immune response, dampening inflammatory signaling pathways, and mitigating tissue damage (50). Clinical studies of Treg-based treatments for IHD are currently underway, and emerging small-molecule and biologic therapies for Tregs are expected to be used for IHD.
There are certain limitations to consider in this study. First, the GWAS pooled data used were obtained from European populations, and therefore, the conclusions cannot be generalized to other population types. Second, there is potential horizontal pleiotropy in the exposure of proxy SNPs, which could have introduced potential bias in the results.
Our study had pre-specifically planned to perform the sensitivity analysis if more than 2 SNP variants were identified; although, none of the exposure had instrumental SNPs exceeding 2 in number. Third, the results of this study cannot reflect the treatment effect of immunotherapy based on specific Tregs subtypes. Further investigation is encouraged to explore this potential treatment strategy.
Conclusions
This comprehensive bidirectional MR study highlighted the role of high CD28 expression on CD4 Tregs as a protective factor against IHD occurrence. This discovery holds promise for enhancing our comprehension of the immunopathology underlying IHD and may drive the advancement of future cellular therapies associated with this disease.
Acknowledgments
We would like to thank all investigators of the GWAS summary datasets used in this study for publicly sharing their research.
Funding: None.
Footnote
Reporting Checklist: The authors have completed the STROBE-MR reporting checklist. Available at https://jtd.amegroups.com/article/view/10.21037/jtd-23-1790/rc
Peer Review File: Available at https://jtd.amegroups.com/article/view/10.21037/jtd-23-1790/prf
Conflicts of Interest: All authors have completed the ICMJE uniform disclosure form (available at https://jtd.amegroups.com/article/view/10.21037/jtd-23-1790/coif). The authors have no conflicts of interest to declare.
Ethical Statement: The authors are accountable for all aspects of the work in ensuring that questions related to the accuracy or integrity of any part of the work are appropriately investigated and resolved. The study was conducted in accordance with the Declaration of Helsinki (as revised in 2013).
Open Access Statement: This is an Open Access article distributed in accordance with the Creative Commons Attribution-NonCommercial-NoDerivs 4.0 International License (CC BY-NC-ND 4.0), which permits the non-commercial replication and distribution of the article with the strict proviso that no changes or edits are made and the original work is properly cited (including links to both the formal publication through the relevant DOI and the license). See: https://creativecommons.org/licenses/by-nc-nd/4.0/.
References
- Benjamin EJ, Blaha MJ, Chiuve SE, et al. Heart Disease and Stroke Statistics-2017 Update: A Report From the American Heart Association. Circulation 2017;135:e146-603. [Crossref] [PubMed]
- Mogensen UM, Gong J, Jhund PS, et al. Effect of sacubitril/valsartan on recurrent events in the Prospective comparison of ARNI with ACEI to Determine Impact on Global Mortality and morbidity in Heart Failure trial (PARADIGM-HF). Eur J Heart Fail 2018;20:760-8. [Crossref] [PubMed]
- Zhang N, Yang C, Liu YJ, et al. Analysis of susceptibility genes and myocardial infarction risk correlation of ischemic cardiomyopathy based on bioinformatics. J Thorac Dis 2022;14:3445-53. [Crossref] [PubMed]
- Fu X, Wang J, Jiang S, et al. Mortality trend analysis of ischemic heart disease in China between 2010 and 2019: a joinpoint analysis. BMC Public Health 2023;23:644. [Crossref] [PubMed]
- Furman D, Campisi J, Verdin E, et al. Chronic inflammation in the etiology of disease across the life span. Nat Med 2019;25:1822-32. [Crossref] [PubMed]
- Libby P. Inflammation in atherosclerosis. Nature 2002;420:868-74. [Crossref] [PubMed]
- Saigusa R, Winkels H, Ley K. T cell subsets and functions in atherosclerosis. Nat Rev Cardiol 2020;17:387-401. [Crossref] [PubMed]
- Bansal SS, Ismahil MA, Goel M, et al. Dysfunctional and Proinflammatory Regulatory T-Lymphocytes Are Essential for Adverse Cardiac Remodeling in Ischemic Cardiomyopathy. Circulation 2019;139:206-21. [Crossref] [PubMed]
- Zhuang R, Feinberg MW. Regulatory T cells in ischemic cardiovascular injury and repair. J Mol Cell Cardiol 2020;147:1-11. [Crossref] [PubMed]
- Amin HZ, Sasaki N, Hirata KI, Regulatory T. Cell Immunity in Atherosclerosis. Acta Med Indones 2017;49:63-8.
- Zhao J, Hou Y, Xie T, et al. Genome-wide Mendelian randomization identifies putatively causal gut microbiota for multiple peptic ulcer diseases. Front Immunol 2023;14:1260780. [Crossref] [PubMed]
- Gao L, Di X, Gao L, et al. The Frailty Index and colon cancer: a 2-sample Mendelian-randomization study. J Gastrointest Oncol 2023;14:798-805. [Crossref] [PubMed]
- Peng H, Wu X, Wen Y, et al. Age at first birth and lung cancer: a two-sample Mendelian randomization study. Transl Lung Cancer Res 2021;10:1720-33. [Crossref] [PubMed]
- Skrivankova VW, Richmond RC, Woolf BAR, et al. Strengthening the Reporting of Observational Studies in Epidemiology Using Mendelian Randomization: The STROBE-MR Statement. JAMA 2021;326:1614-21. [Crossref] [PubMed]
- Davies NM, Holmes MV, Davey Smith G. Reading Mendelian randomisation studies: a guide, glossary, and checklist for clinicians. BMJ 2018;362:k601. [Crossref] [PubMed]
- Orrù V, Steri M, Sidore C, et al. Complex genetic signatures in immune cells underlie autoimmunity and inform therapy. Nat Genet 2020;52:1036-45. [Crossref] [PubMed]
- Pierce BL, Ahsan H, Vanderweele TJ. Power and instrument strength requirements for Mendelian randomization studies using multiple genetic variants. Int J Epidemiol 2011;40:740-52. [Crossref] [PubMed]
- Bowden J, Davey Smith G, Haycock PC, et al. Consistent Estimation in Mendelian Randomization with Some Invalid Instruments Using a Weighted Median Estimator. Genet Epidemiol 2016;40:304-14. [Crossref] [PubMed]
- Hartwig FP, Davey Smith G, Bowden J. Robust inference in summary data Mendelian randomization via the zero modal pleiotropy assumption. Int J Epidemiol 2017;46:1985-98. [Crossref] [PubMed]
- Bowden J, Davey Smith G, Burgess S. Mendelian randomization with invalid instruments: effect estimation and bias detection through Egger regression. Int J Epidemiol 2015;44:512-25. [Crossref] [PubMed]
- Verbanck M, Chen CY, Neale B, et al. Detection of widespread horizontal pleiotropy in causal relationships inferred from Mendelian randomization between complex traits and diseases. Nat Genet 2018;50:693-8. [Crossref] [PubMed]
- Burgess S. Sample size and power calculations in Mendelian randomization with a single instrumental variable and a binary outcome. Int J Epidemiol 2014;43:922-9. [Crossref] [PubMed]
- Hemani G, Zheng J, Elsworth B, et al. The MR-Base platform supports systematic causal inference across the human phenome. Elife 2018;7:e34408. [Crossref] [PubMed]
- Wang W, Hu M, Liu H, et al. Global Burden of Disease Study 2019 suggests that metabolic risk factors are the leading drivers of the burden of ischemic heart disease. Cell Metab 2021;33:1943-1956.e2. [Crossref] [PubMed]
- Al-Lamee RK, Foley M, Rajkumar CA, et al. Revascularization in stable coronary artery disease. BMJ 2022;377:e067085. [Crossref] [PubMed]
- Chang D, Fan T, Gao S, et al. Application of mesenchymal stem cell sheet to treatment of ischemic heart disease. Stem Cell Res Ther 2021;12:384. [Crossref] [PubMed]
- Dehkordi NR, Dehkordi NR, Farjoo MH. Therapeutic properties of stem cell-derived exosomes in ischemic heart disease. Eur J Pharmacol 2022;920:174839. [Crossref] [PubMed]
- Poomani MS, Mariappan I, Perumal R, et al. Mesenchymal Stem Cell (MSCs) Therapy for Ischemic Heart Disease: A Promising Frontier. Glob Heart 2022;17:19. [Crossref] [PubMed]
- Ait-Oufella H, Salomon BL, Potteaux S, et al. Natural regulatory T cells control the development of atherosclerosis in mice. Nat Med 2006;12:178-80. [Crossref] [PubMed]
- Yin M, Zhang J, Wang Y, et al. Deficient CD4+CD25+ T regulatory cell function in patients with abdominal aortic aneurysms. Arterioscler Thromb Vasc Biol 2010;30:1825-31. [Crossref] [PubMed]
- Dinh TN, Kyaw TS, Kanellakis P, et al. Cytokine therapy with interleukin-2/anti-interleukin-2 monoclonal antibody complexes expands CD4+CD25+Foxp3+ regulatory T cells and attenuates development and progression of atherosclerosis. Circulation 2012;126:1256-66. [Crossref] [PubMed]
- Meng X, Li W, Yang J, et al. Regulatory T cells prevent plaque disruption in apolipoprotein E-knockout mice. Int J Cardiol 2013;168:2684-92. [Crossref] [PubMed]
- Weirather J, Hofmann UD, Beyersdorf N, et al. Foxp3+ CD4+ T cells improve healing after myocardial infarction by modulating monocyte/macrophage differentiation. Circ Res 2014;115:55-67. [Crossref] [PubMed]
- Meng X, Yang J, Dong M, et al. Regulatory T cells in cardiovascular diseases. Nat Rev Cardiol 2016;13:167-79. [Crossref] [PubMed]
- Wigren M, Rattik S, Yao Mattisson I, et al. Lack of Ability to Present Antigens on Major Histocompatibility Complex Class II Molecules Aggravates Atherosclerosis in ApoE(-/-) Mice. Circulation 2019;139:2554-66. [Crossref] [PubMed]
- Sharma M, Schlegel MP, Afonso MS, et al. Regulatory T Cells License Macrophage Pro-Resolving Functions During Atherosclerosis Regression. Circ Res 2020;127:335-53. [Crossref] [PubMed]
- Trynka G, Hunt KA, Bockett NA, et al. Dense genotyping identifies and localizes multiple common and rare variant association signals in celiac disease. Nat Genet 2011;43:1193-201. [Crossref] [PubMed]
- Eyre S, Bowes J, Diogo D, et al. High-density genetic mapping identifies new susceptibility loci for rheumatoid arthritis. Nat Genet 2012;44:1336-40. [Crossref] [PubMed]
- International Multiple Sclerosis Genetics Consortium (IMSGC). Analysis of immune-related loci identifies 48 new susceptibility variants for multiple sclerosis. Nat Genet 2013;45:1353-60. [Crossref] [PubMed]
- Liu JZ, Hov JR, Folseraas T, et al. Dense genotyping of immune-related disease regions identifies nine new risk loci for primary sclerosing cholangitis. Nat Genet 2013;45:670-5. [Crossref] [PubMed]
- Okada Y, Wu D, Trynka G, et al. Genetics of rheumatoid arthritis contributes to biology and drug discovery. Nature 2014;506:376-81. [Crossref] [PubMed]
- Liu JZ, van Sommeren S, Huang H, et al. Association analyses identify 38 susceptibility loci for inflammatory bowel disease and highlight shared genetic risk across populations. Nat Genet 2015;47:979-86. [Crossref] [PubMed]
- Ellinghaus D, Jostins L, Spain SL, et al. Analysis of five chronic inflammatory diseases identifies 27 new associations and highlights disease-specific patterns at shared loci. Nat Genet 2016;48:510-8. [Crossref] [PubMed]
- Qiu F, Tang R, Zuo X, et al. A genome-wide association study identifies six novel risk loci for primary biliary cholangitis. Nat Commun 2017;8:14828. [Crossref] [PubMed]
- Cao Z, Wara AK, Icli B, et al. Kruppel-like factor KLF10 targets transforming growth factor-beta1 to regulate CD4(+)CD25(-) T cells and T regulatory cells. J Biol Chem 2009;284:24914-24. [Crossref] [PubMed]
- Butcher MJ, Filipowicz AR, Waseem TC, et al. Atherosclerosis-Driven Treg Plasticity Results in Formation of a Dysfunctional Subset of Plastic IFNγ+ Th1/Tregs. Circ Res 2016;119:1190-203. [Crossref] [PubMed]
- Mor A, Planer D, Luboshits G, et al. Role of naturally occurring CD4+ CD25+ regulatory T cells in experimental atherosclerosis. Arterioscler Thromb Vasc Biol 2007;27:893-900. [Crossref] [PubMed]
- Swirski FK, Nahrendorf M. Leukocyte behavior in atherosclerosis, myocardial infarction, and heart failure. Science 2013;339:161-6. [Crossref] [PubMed]
- Ellis GI, Sheppard NC, Riley JL. Genetic engineering of T cells for immunotherapy. Nat Rev Genet 2021;22:427-47. [Crossref] [PubMed]
- Zhou X, Bailey-Bucktrout S, Jeker LT, et al. Plasticity of CD4(+) FoxP3(+) T cells. Curr Opin Immunol 2009;21:281-5. [Crossref] [PubMed]