In search for “healthy” landing zones for coronary stent placement: are the largest intrasegmental lumens adequate?
Highlight box
Key findings
• Current lesion definition is not consistent with healthy segmental reference sites.
What is known and what is new?
• Finding the landing zones with low plaque burden is essential for stent placement.
• Additional thin-cap fibroatheromas appear at infarct-related lesion borders.
What is the implication, and what should change now?
• We recommend using external elastic membrane instead of maximal lumens to find appropriate landings.
Introduction
Background
From a pathologist’s perspective, coronary atherosclerosis is a focal instead of a diffuse intimal disease (1). In this context, a coronary lesion is supposed to be enclosed between proximal and distal reference segments (RSs) that are the sites with the largest lumens within the same vessel segment, although not with the least plaque (2,3). When performing a percutaneous coronary intervention (PCI), the distance covered by stenting should correspond with the lesion extent. If the stent is not optimally placed (i.e., “normal to normal”, there remains a substantial risk of serious post-procedural complications (4). In diffuse coronary artery disease (CAD), it is quite challenging to accurately determine lesion borders and, consequently, landing zones for stent placement.
Rationale and knowledge gap
Angiography is a standard method for assessing coronary anatomy and guiding PCI. Nevertheless, evaluating the lesion extent, composition, and plaque burden (PB) seems to be inadequate. Intravascular imaging with ultrasound (IVUS) and optical coherence tomography (OCT) are alternatives for assessment, they have both superior resolution and are extensively used. IVUS can precisely assess vessel structure and PB; atherosclerosis, for example, was found even in angiographically normal RSs (5). OCT provides exceptional axis (10–15 µm) and lateral resolution (25–40 µm), and OCT images share impressive similarity with histology (6). Several studies suggested that optimal landing zones should contain RSs with PB not exceeding 40% to 50% (7,8). Despite its somewhat limited ability to determine the total PB, OCT allows the calculation of the plaque-free wall (PFW); indeed, a PFW angle ≥220° has been proposed as a surrogate indicator for the PB <40% (9).
Objective
In contrast to the main bodies of coronary lesions, a paucity of literature reviews the identification of lesion boundaries. The objective of our study was to characterize by OCT the borders of culprit lesions that conformed to the definition above (2,3). We sought to appraise the adequacy of RSs to provide suitable landing zones. Finally, we explored the impact of the selected zones on peri-procedural complication. This manuscript is written following the STROBE reporting checklist (available at https://jtd.amegroups.com/article/view/10.21037/jtd-23-924/rc).
Methods
Study design and population
We conducted a retrospective study to investigate the borders of the culprit lesions in patients who underwent elective or urgent PCI at our institution from February 6, 2015, through October 9, 2020. Baseline and post-stenting OCT were mandatory for inclusion in the study. Identification of the culprits involved electrocardiography, non-invasive tests, and lesion morphology. Patients with Type 1 myocardial infarction (MI) (10) were assigned to Group 1, and those with stable angina were placed in Group 2. Individuals with MI in the same myocardial areas, previous PCI or surgical coronary revascularization of culprit arteries, non-obstructive or non-culprit lesions, missing initial or post-stenting pullbacks, or suboptimal OCT examinations were excluded (Figure S1). Clinical data were collected by reviewing medical records, and procedural details were entered into a database at the time of PCI. Two independent researchers comprehensively analyzed the OCT images; in case of disagreement, a consensus had to be reached. The study was conducted in accordance with the Declaration of Helsinki (as revised in 2013). The retrospective analysis was approved by the Institutional Review Board of General Hospital Dr. Franc Derganc, Ul. Padlih borcev 13A, 5290 Šempeter pri Gorici (SBG 4/2023). The individual consent was waived because of the retrospective manner of the analysis.
Diagnostic and therapeutic procedures
Invasive procedures were performed with standard radiographic equipment, technique, and catheters. Coronary angiography was completed in multiple projections, and the view showing the worst stenosis was used for further analysis. OCT was performed using a commercially available Fourier-domain OCT system (Ilumien Optis, Abbott Vascular, Santa Clara, CA, USA) and a 2.7-F imaging catheter (Dragonfly Duo/Optis, Abbott Vascular, Santa Clara, CA, USA). In occluded vessels, a predilatation with a small balloon (i.e., ≤2.0 mm) was allowed to enable the passage of the imaging probe. Automated pullback at the speed of 20 mm/s, assisted by a power contrast injector, was accomplished after nitroglycerin administration to examine the culprit vessel in a survey or high-resolution mode. Baseline and post-stenting examinations were digitally stored for further analysis. PCI was performed according to contemporary practice. Antiplatelet and antithrombin drugs were given at the start of the procedure, and thrombo-aspiration was used in the case of a high thrombus burden. Six PCI operators were instructed to use the largest intra-segmental lumens as the landing zones, according to the practice at the time, and avoid extensive lipid-rich tissues. The technicians provided the required measurements, while the selection of the landing zones and stents was the operator’s decision.
Angiographic analysis
Angiographic definitions are explained in the Appendix 1. Quantitative analysis with an edge detection system was used to compute percent diameter stenosis (%DS). Lesion length was measured as a distance between seemingly normal proximal and distal sites. The lesions were graded as discreet (<10 mm), tubular (10–20 mm), and diffuse (>20 mm) (11). They were located in 15 segments according to the American Heart Association reporting system (12). The grades of blood flow were assessed by the Thrombolysis in Myocardial Infarction (TIMI) Grade Flow scoring system (13); grades ≤2 were considered to be impaired flow. Post-stenting edge dissections were categorized according to the National Heart, Lung, and Blood Institute classification system (14).
OCT analysis
OCT definitions are described in Appendix 1. Quantitative analysis of culprit lesions was performed at RSs and minimum lumens with proprietary software (Abbott Vascular, Santa Clara, CA, USA). Segments were screened in the lumen profile, and the OCT system automatically tracked lumen contours, located maximum and minimum lumens, and computed percent area stenosis (%AS). The length was measured as the distance from proximal to distal RSs [lumen-based approach (LBA)] or, from proximal to distal intra-segmental border sites showing sufficient external elastic membrane [EEM-based approach (EBA)]. Qualitative analysis was performed at RSs and adjacent marginal segments (AMSs) up to 5 mm beyond RSs (Figure 1). The analysis involved border visibility, presence and types of atherosclerotic plaques, and post-stenting edge dissections. Border visibility was acceptable if the EEM appeared in ≥180° of the vessel circumference; EEM in ≥220° indicated a PB <40% (9,15). Normal vessel wall was distinguished by a three-layered structure with a maximum thickness of ≤300 µm; a width up to 600 µm was considered a non-atherosclerotic adjustment (16). Plaques were classified as fibrous plaque (FP), fibrocalcific plaque (FCP), thick-cap fibroatheroma (ThCFA), thin-cap fibroatheroma (TCFA), and calcified nodules (3). TCFA were defined as a lipid-rich plaque with a fibrous cap thickness of <65 µm and a maximum lipid arc >90° (2). The landing zones were optimal (“normal to normal”) or acceptable (PFW angle ≥220° without extensive lipid-rich tissues) (7-9).
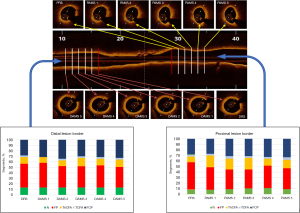
Edge dissections were defined as disruptions of the luminal vessel surface 5 mm beyond stent edges; they were further divided into major or minor dissections (6).
Endpoints
The OCT endpoint was a proportion of optimal (“normal to normal”) and acceptable landing zones (PFW angle ≥220°) in the whole group, patients with acute MI, and those with stable angina. Similarly, the PCI endpoint was a proportion of periprocedural complications (i.e., TIMI blood flow ≤2, edge dissections) in the whole group, patients with acute MI, and those with stable angina.
Statistical analysis
Continuous variables were shown as means with standard deviations or medians with interquartile ranges when the distribution was not normal. Categorical variables were displayed as frequencies with percentages. A Student’s t-test and χ2 test or Fischer exact test were used to compare continuous and categorical variables, respectively. Logistic regression analysis was used to predict the risk of peri-procedural complications (TIMI flow grades ≤2 and stent edge dissections). All tests were two-tailed, and statistical significance was considered for P values <0.05. All analyses were performed using IBM SPSS software (version 20.0, SPSS Inc., Chicago, IL, USA).
Results
A total of 67/101 (66%) patients planned for single culprit lesion PCI with available OCT were enrolled in the study; 34 (34%) patients were excluded for various reasons (Figure S1). Thirty-four patients (Group 1) presented with acute MI, 11 with ST-segment elevation MI (STEMI), and 18 with non-ST-segment elevation MI. The remaining 33 patients (Group 2) had stable angina. Clinical characteristics are summarized in Table 1. Most patients were males in their mid-sixties, and nearly 20% had diabetes. Group 2 patients were older, expressed different risk factors, suffered from long-standing symptoms, and some were already taking antiplatelet drugs and statins.
Table 1
Variables | All patients (n=67) | Group 1 (n=34) | Group 2 (n=33) | P value |
---|---|---|---|---|
Age, years | 63.5±11.3 | 60.3±12.6 | 66.8±8.9 | 0.018 |
Male | 44 (65.7) | 22 (64.7) | 22 (66.7) | 0.866 |
Hypertension | 48 (71.6) | 20 (58.8) | 28 (84.8) | 0.018 |
Hypercholest | 28 (41.8) | 13 (38.2) | 15 (45.5) | 0.549 |
Diabetes | 12 (17.9) | 5 (14.7) | 7 (21.2) | 0.487 |
Smoking | 13 (19.4) | 10 (29.4) | 3 (9.1) | 0.035 |
Prior AP | 28 (41.8) | 1 (2.9) | 27 (81.8) | <0.001 |
Prior MI | 11 (16.4) | 0 | 11 (33.3) | <0.001 |
Prior PCI | 17 (25.4) | 1 (2.9) | 16 (48.5) | <0.001 |
Prior aspirin | 27 (40.3) | 3 (8.8) | 24 (72.7) | <0.001 |
Prior thyenopiridins | 6 (9.0) | 0 | 6 (18.2) | 0.009 |
Prior statins | 18 (26.9) | 5 (14.7) | 13 (39.4) | 0.023 |
1–3 VD | ||||
1-VD | 46 (68.7) | 23 (67.6) | 23 (69.7) | 0.856 |
2-VD | 16 (23.9) | 7 (20.6) | 9 (27.3) | 0.521 |
3-VD | 5 (7.5) | 4 (11.8) | 1 (3.0) | 0.174 |
Culprit | 0.032 | |||
LCA | 5 (7.5) | 2 (5.9) | 3 (9.1) | |
LAD | 35 (52.2) | 14 (41.2) | 21 (63.6 | |
LCX | 13 (19.4) | 6 (17.6) | 7 (21.2) | |
RCA | 14 (20.9) | 12 (35.3) | 2 (6.1 | |
Segment | 0.221 | |||
1 | 2 (3.0) | 2 (5.9) | 0 | |
2 | 10 (14.9) | 9 (26.5) | 1 (3.0) | |
3 | 2 (3.0) | 1 (2.9) | 1 (3.0) | |
5 | 5 (7.5) | 2 (5.9) | 3 (9.1) | |
6 | 19 (28.4) | 8 (23.5) | 11 (33.3) | |
7 | 15 (22.4) | 5 (14.7) | 10 (30.3) | |
8 | 1 (1.5) | 1 (2.9) | 0 | |
11 | 7 (10.4) | 3 (8.8) | 4 (12.1) | |
12 | 2 (3.0) | 1 (2.9) | 1 (3.0) | |
13 | 4 (6.0) | 2 (5.9) | 2 (6.1) | |
Proximal segment | 60 (89.6) | 31 (91.2) | 29 (87.9) | 0.659 |
%DS | 90 [80–99] | 97 [90–100] | 80 [70–90] | <0.001 |
Occlusion | 12 (17.9) | 12 (35.3) | 0 | <0.001 |
Length | 0.182 | |||
Discreet, <10 mm | 6 (9.0) | 1 (2.9) | 5 (15.2) | |
Tubular, 10–20 mm | 39 (58.2) | 20 (58.8) | 19 (57.6) | |
Diffuse, >20 mm | 22 (32.8) | 13 (38.2) | 9 (27.3) |
Values are mean ± standard deviation, median [25th, 75th percentiles], or n (%), as appropriate. Groups 1 and 2 are compared using unpaired t-test for equality of means or χ2 test. Group 1, patients with acute MI; Group 2, patients with stable AP. Hypercholest, hypercholesterolemia; AP, angina pectoris; MI, myocardial infarction; PCI, percutaneous coronary intervention; 1–3 VD, number of vessels with angiographically significant coronary artery stenosis; LCA, left coronary artery; LAD, left anterior descending coronary artery; LCX, left circumflex coronary artery; RCA, right coronary artery; %DS, percent diameter stenosis.
Angiographic characteristics are shown in Table 1. For all patients, culprit lesions usually appeared in proximal segments, their median %DS was 90%, and only 9% of lesions were discreet. In Group 1, the culprits were narrower and more evenly distributed between epicardial arteries.
Quantitative OCT characteristics are shown in Table 2. The lesions appeared in sizeable arteries with significant tapering (median cross-sectional area, proximally 7.63 mm2vs. distally 6.84 mm2, P<0.001). Luminal dimensions at the borders were comparable in both study groups, while %AS was significantly greater in Group 1 (P=0.019). Lesion lengths were measured by two OCT methods that diverged significantly (median length, LBA 17 mm vs. EBA 19 mm, P<0.001); insufficient EEM visibility at RSs prevented EBA calculations in 16 (24%) patients. Importantly, LBA dimensions closely resembled angiographic measurements.
Table 2
Variables | All patients (n=67) | Group 1 (n=34) | Group 2 (n=33) | P value |
---|---|---|---|---|
Quantitative OCT characteristics† | ||||
Lesion diameter and area | ||||
Prox D, mm | 3.34 [3.05–3.79] | 3.29 [3.04–3.75] | 3.41 [3.10–3.87] | 0.845 |
Min D, mm | 1.68 [1.36–2.18] | 1.55 [1.15–1.96] | 1.83 [1.51–2.27] | 0.215 |
Dist D, mm | 3.13 [2.67–3.64] | 3.20 [2.60–3.74] | 3.1 [2.72–3.45] | 0.408 |
Prox area, mm2 | 7.63 [6.03–9.85] | 7.47 [6.22–10.38] | 7.83 [5.84–9.76] | 0.410 |
Min area, mm2 | 1.59 [1.15–2.74] | 1.42 [0.86–1.95] | 1.97 [1.36–3.06] | 0.249 |
Dist area, mm2 | 6.84 [5.38–8.54] | 6.62 [4.26–9.03] | 6.85 [5.63–8.25] | 0.795 |
%AS | 78 [69–86] | 83 [76.30–89] | 74 [65–84] | 0.019 |
Lesion length | ||||
Angio length, mm | 17.5 [13.2–23.1] | 19.8 [15.1–27.7] | 16.5 [13.2–20.1] | 0.013 |
LBA length, mm | 17 [13–23] | 18 [13–25] | 17 [13.5–21] | 0.676 |
EBA length‡, mm | 19 [15–26] | 20 [16–28] | 18.5 [14.3–23.5] | 0.562 |
Qualitative characteristics† | ||||
Thin-cap fibroatheromas at reference and adjacent marginal segments | ||||
All TCFA | 13 (19.4) | 10 (29.4) | 3 (9.1) | 0.035 |
RS | 5 (7.5) | 5 (14.7) | 0 | 0.022 |
Prox RS | 3 (4.5) | 3 (8.8) | 0 | 0.081 |
Dist RS | 2 (3.0) | 2 (5.9) | 0 | 0.157 |
AMS | 11 (16.4) | 8 (23.5) | 3 (9.1) | 0.111 |
Prox AMS | 5 (7.5) | 5 (14.7) | 0 | 0.022 |
Dist AMS | 7 (10.4) | 4 (11.8) | 3 (9.1) | 0.721 |
Values are n (%) or median (25th, 75th percentiles), as appropriate. Groups 1 and 2 are compared using unpaired t-test for equality of means or χ2 test. †, the majority of qualitative characteristics are shown in Table S2. Group 1, patients with acute myocardial infarction; Group 2, patients with stable angina. Prox D, proximal reference diameter; Min D, minimal lesion diameter; Dist D, distal reference diameter; Prox Area, proximal reference area; Min Area, minimal lesion area; Dist Area, distal reference area; %AS, percent area stenosis; Angio length, distance between angiographically determined reference segments; LBA length, distance between reference segments determined by lumen-based approach; EBA length, distance between reference segments determined by external elastic membrane-based approach (‡, data collected from 51 patients); TCFA, thin-cap fibroadenoma; RS, reference segments; Prox RS, proximal reference segments; Dist RS, distal reference segments; AMS, adjacent marginal segments; Prox AMS, proximal adjacent marginal segments; Dist AMS, distal adjacent marginal segments.
EEM visibility at the lesion borders is presented in Table S1. Acceptable visibility (EEM ≥180°) was confirmed in 67% of the proximal and 55% of the distal RSs; exploring distal, but not proximal AMSs, somewhat improved visibility. Acceptable PB (PFW angle ≥220°) was discovered in 28% of the proximal and 31% of the distal RSs; PB increased at proximal AMSs by 32% (P=0.023), while it decreased distally by 33% (P=0.005). There were no significant differences between the groups.
The vessel wall composition at the lesion borders is shown in Tables S2-S7 and Figure 2. The normal structure was surprisingly uncommon: it was discovered in 9% of the proximal and 13.5% of the distal RSs and remained infrequent at AMSs. Plaques prevailing at both borders were FP, followed by FCP and ThCFA. TCFA were found at minimal lumens in 24 (35.8%) patients and at lesion borders in 13 (19.4%) patients; they occurred in 7.6% of RSs and 16.4% of AMSs (Table 2). In Group 1, as compared with Group 2, TCFA accumulations were more frequent at minimum lumens (67.6% vs. 3.0%, P<0.001) as well as proximal borders (29.4% vs. 9.1%, P=0.035).
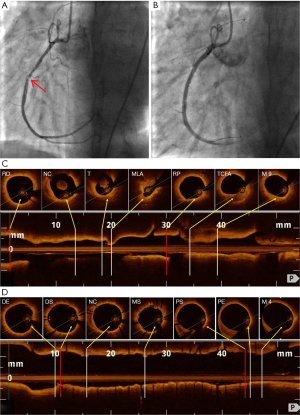
OCT discovered optimal landing zones in 9% of proximal and 13.5% of distal RSs. Acceptable landing zones appeared in 28% of proximal and 31% of distal RSs. No significant differences were seen between the study groups.
PCI characteristics are displayed in Table 3. Overall, the median stent diameter was 3.5 mm, and the cumulative length was 22.5 mm. The stents were larger and slightly longer in Group 1. At the end of PCI, TIMI flow ≤2 was detected in 5 (7.5%) enrolled patients, more frequent in Group 1 (14.7% vs. 0% in Group 2, P=0.029). OCT discovered more stent edge dissections than angiography (26.9% vs. 19.4%, respectively; P=0.024). Based on OCT, the dissections were more frequent in Group 1 (38.2% vs. 15.2% in Group 2, P=0.039), and nearly half required additional stenting.
Table 3
Variables | All patients (n=67) | Group 1 (n=34) | Group 2 (n=33) | P value |
---|---|---|---|---|
Stents, n | 1.2±0.7 | 1.3±0.7 | 1.0±0.6 | 0.065 |
Stent diam, mm | 3.5 (3.0–3.5) | 3.5 (3.0–3.9) | 3.0 (3.0–3.5) | 0.008 |
Cumul length, mm | 22.5 (18.0–32.5) | 24.5 (18.0–37.5) | 20.0 (15.0–27.5) | 0.166 |
TIMI flow 0–3 | 2.9±0.2 | 2.8±0.3 | 3.0±0.0 | 0.029 |
TIMI flow ≤2 | 5 (7.5) | 5 (14.7) | 0 (0) | 0.029 |
Ang dissect | 13 (19.4) | 8 (23.5) | 5 (15.2) | 0.386 |
Ang dissect type | 0.705 | |||
A | 7 (10.4) | 4 (11.8) | 3 (9.1) | |
B | 5 (7.5) | 3 (8.8) | 2 (6.1) | |
C | 1 (1.5) | 1 (2.9) | 0 (0) | |
OCT dissect | 18 (26.9) | 13 (38.2) | 5 (15.2) | 0.039 |
OCT dissect type | 0.101 | |||
Major | 8 (11.9) | 6 (17.6) | 2 (6.1) | |
Minor | 10 (14.9) | 7 (20.6) | 3 (9.1) |
Values are mean ± standard deviation, median (25th, 75th percentiles), or n (%), as appropriate. Groups 1 and 2 are compared using unpaired t-test for equality of means or χ2 test. Group 1, patients with acute myocardial infarction; Group 2, patients with stable angina. Stent diam, stent diameter; Cumul length, cumulative stent length; TIMI, thrombolysis in myocardial infarction; Ang dissect, edge dissections detected by angiography; OCT dissect, edge dissections detected by optical coherence tomography.
Finally, logistic regression analysis was used to predict peri-procedural complications (Table S8). Using univariate analysis, the risk of TIMI flow ≤2 following stenting diminished in patients without STEMI [odds ratio (ORs) =0.049, 95% confidence interval (CI): 0.005–0.49; P=0.010] or without TCFA at proximal RSs (OR =0.028, 95% CI: 0.002–0.4; P=0.008); however, multivariate analysis ascertained that only STEMI absence reduced the risk for impaired flow after stenting (OR =0.032, 95% CI: 0.002–0.825; P=0.037). As determined by univariate analysis, stent edge dissections were associated with several clinical factors and larger stent diameters (OR =3.98, 95% CI: 1.12–14.11; P=0.032); however, none of the listed variables were able to predict dissections by multivariate analysis.
Discussion
Key findings
Searching for “healthy” landing zones has been appreciated as one of the fundamental principles of efficient stent implantation (2). Our results indicated that (I) normal vessel wall enabling optimal stent placement was infrequent at the largest intra-segmental lumens delineating the culprit lesions; (II) PB at those sites exceeded recommended threshold in approximately 70% of study participants; (III) tissue composition at the culprit borders was similar in patients with stable angina and acute MI except for increased clusters of TCFA in the latter group; (IV) RSs determined by the largest intra-segmental lumens underestimated true lesion lengths; (V) available variables allowed us to anticipate only post-procedural blood flow impairment.
Strengths and limitations
A balanced structure of the study population and detailed analysis of the data acquired by histology resembling OCT imaging were perceived to be the strength of our study. The proportional equipoise between patients with stable angina and acute MI was difficult to obtain; however, it enabled a comparison between two different pathologic conditions. Limitations of our study included those inherent to retrospective analysis and single-center studies. The sample size was small and lack statistical power to make inferences other than the generation of a hypothesis. The precise placement of the stents over the selected landing zones was not confirmed by the co-registration of angiography and OCT. Finally, the adequacy of our stent sizing/placing technique was tested only on peri-procedural complications.
Comparison with similar research
Complying with the classification proposed by Radu (16), we only detected the normal structure in 9% of the proximal and 13.5% of the distal RSs. In a similar IVUS study (5), the normal appearance occurred in 6.8% of the angiographically disease-free RSs. Unsurprisingly, we found atherosclerotic plaques in 91% of the proximal and 86% of the distal RSs. Similar results were published by Gonzalo and colleagues (17). Given the limited penetration depth of the near-infrared light used by OCT, the accurate assessment of PB is hardly realistic. However, a significant correlation between PB (assessed by IVUS) and PFW (determined by OCT) has been recently reported; PFW <220° indicated a suboptimal landing zone in 78% of cases, whereas PFW ≥220° prevented excessive stenting in 84% of cases (9). We detected PFW ≥220° in only 28% of the proximal and 31% of the distal RSs; PB increased in the proximal AMSs by 32% and decreased distally by 33%. It might be argued that the majority of intra-segmental RSs did not represent the optimal landing zones.
In contrast to the main body of the culprit lesions, there is a paucity of data on TCFA at their boundaries. A systematic examination of the coronary arteries with IVUS showed that TCFA are typically clustered at the proximal vessel segments (18). In patients with STEMI, stent placement failed to cover the whole lesion length in about 50% of all procedures, leaving behind stent edges necrotic cores and TCFA (19). In our study, TCFA were detected at RSs and related AMSs in approximately 20% of all patients, particularly in Group 1.
The LBA method is always available, while EBA requires at least 180° of the EEM to be visible. The Ilumien III trial (20) found sufficient EEM visibility in 84% of patients. Conversely, we recognized EEM ≥180° in 67% of the proximal and 55% of the distal RSs.
There is an unsettled balance between adequate lesion coverage and using excessively long stents. Diffuse coronary stenoses are associated with a 17% increased risk of major adverse cardiac events for every 10 mm of stent deployed (21). Angiography and LBA yield shorter stent lengths but are associated with an increased risk of geometric miss. If the 180° EEM visibility at the LBA-determined RSs had been applied, the lesion length could be measured in 76% of our cases. We suggest that the landing zones with a ≥220° EEM visibility (i.e., PFW angle ≥220°) should be pursued, even outside the target segments.
Previous studies linked the TCFA-containing lesions with a risk of distal embolization and Type IVa MI (22). Indeed, in our study, TCFA at proximal lesion borders extending beyond the culprit minimal lumens were associated with peri-procedural blood flow impairment (Figure 2). However, according to multivariate analysis and data in the literature (23), a complex pathogenetic mechanism generating flow deterioration has been suggested. The incidence of stent edge dissections depends on the diagnostic method used: it was reported to be 1.7% to 4.8% for angiography, 7.8% to 20% for IVUS, and 15.5% to 23.6% for OCT (24). The risk of dissection is six times higher when the stent is placed over a diseased region abundant with FCP, lipid-rich plaques, and TCFA (18). The frequency of dissections in our study was comparable to other results; however, despite the higher rates in the acute MI group, we did not show any association with the plaque composition at the lesion borders.
Explanation of findings
The normal vessel wall is a three-layered structure composed of the intima, media, and adventitia with a thickness <250 µm (25). An excellent agreement in measuring the intima was reported between histology and OCT (26). In the latter, a cut-off of 300 µm was applied to differentiate between the normal intima and adaptive changes and >600 µm to identify the pathological intimal thickness that denotes the end of the “healthy” vessel wall (16).
The distance to be covered by stenting should correspond with the lesion extent; otherwise, there remains a substantial risk of post-procedural MI, restenosis, and stent thrombosis (4). Many pathologists perceive atherosclerosis as a focal disease (1); however, the accumulated evidence does not comply with this limited view. First, accurate lesion borders are difficult to establish with angiography as the plaques are invisible until the lesion occupies 40% to 50% of the vessel cross-section (8). Furthermore, when atherosclerosis appears at any vessel site, it is almost impossible to find a normal segment (5). In patients with advanced CAD, diffuse “plaquing” was revealed in 90% of the arteries (27). Finally, invasive measurements of coronary blood flow allowed the pattern of CAD to be characterized as focal, diffuse, or mixed distribution (28).
TCFA are considered precursors of plaque rupture and, consequently, unstable clinical presentations. They are defined as lesions with fibrous caps <65 µm, large lipid cores, and macrophage infiltration (29). Currently, OCT is the only imaging modality with sufficient resolution to identify TCFA. In their seminal study, Jang et al. found TCFA in 72% of the acute MI patients and only 20% of their stable counterparts (30).
The important role of OCT imaging is to recognize the culprit lesions, assess their morphology, find suitable landing zones, and enable accurate stent sizing/placing. A healthy vessel wall is certainly the best landing zone, though a moderate amount of PB seems acceptable (6). PCI can be associated with a small but not negligible incidence of peri-procedural complications. For example, blood flow deterioration was reported in 0.6% to 5% of elective PCI and 12% to 32% of primary PCI. Atherothrombotic burden has been identified as a major determinant of coronary micro-embolization, particularly when plaques are crushed during PCI (31).
Implications and actions needed
Angiography and LBA-determined RSs, obviously, did not enable the optimal stent placement in our study. If the current lesion definition had been used, both methods underestimated PB and lesion length. Clinical benefits of the EBA method extending across the lesion segments seem highly probable (32), but require long-term follow-up validation in a sufficiently large population (33).
Conclusions
The borders of the culprit lesions, according to the current definition (2,3), showed normal vessel structure and acceptable PB infrequently. The lesion boundaries shared similar tissue composition in stable and unstable patients except for higher TCFA accumulation in acute MI. Angiography and LBA-determined RSs did not enable optimal stent placement. Clinical benefits of the EBA method require long-term follow-up validation.
Acknowledgments
The authors thank Jessica A. Moody, PhD and Michelle Gehring, PhD, ELS of The University of Texas Health Science Center at Houston for editorial and submission support.
Funding: None.
Footnote
Reporting Checklist: The authors have completed the STROBE reporting checklist. Available at https://jtd.amegroups.com/article/view/10.21037/jtd-23-924/rc
Data Sharing Statement: Available at https://jtd.amegroups.com/article/view/10.21037/jtd-23-924/dss
Peer Review File: Available at https://jtd.amegroups.com/article/view/10.21037/jtd-23-924/prf
Conflicts of Interest: All authors have completed the ICMJE uniform disclosure form (available at https://jtd.amegroups.com/article/view/10.21037/jtd-23-924/coif). I.D.G. has received grants from Abbott Laboratories, Medtronic Laboratories, and Cryolife Inc. Payments have been provided to the institution in the form of grants and travel support for meetings; none are related to the creation of this manuscript. He is the Program Director for the Brano Heart Failure Forum and has received support related to travel. B.K. has received institutional grants from Saranas, Edwards LifeSciences, LLC, BioVentrix, Inc and Boston Scientific; none are related to the creation of this manuscript. The other authors have no conflicts of interest to declare.
Ethical Statement: The authors are accountable for all aspects of the work in ensuring that questions related to the accuracy or integrity of any part of the work are appropriately investigated and resolved. The study was conducted in accordance with the Declaration of Helsinki (as revised in 2013). The retrospective analysis was approved by the Institutional Review Board of General Hospital Dr. Franc Derganc, Ul. Padlih borcev 13A, 5290 Šempeter pri Gorici (SBG 4/2023). The individual consent was waived because of the retrospective manner of the analysis.
Open Access Statement: This is an Open Access article distributed in accordance with the Creative Commons Attribution-NonCommercial-NoDerivs 4.0 International License (CC BY-NC-ND 4.0), which permits the non-commercial replication and distribution of the article with the strict proviso that no changes or edits are made and the original work is properly cited (including links to both the formal publication through the relevant DOI and the license). See: https://creativecommons.org/licenses/by-nc-nd/4.0/.
References
- Davies MJ, Woolf N. Atherosclerosis: what is it and why does it occur? Br Heart J 1993;69:S3-11. [Crossref] [PubMed]
- Prati F, Romagnoli E, La Manna A, et al. Long-term consequences of optical coherence tomography findings during percutaneous coronary intervention: the Centro Per La Lotta Contro L'infarto - Optimization Of Percutaneous Coronary Intervention (CLI-OPCI) LATE study. EuroIntervention 2018;14:e443-51. [Crossref] [PubMed]
- Tearney GJ, Regar E, Akasaka T, et al. Consensus standards for acquisition, measurement, and reporting of intravascular optical coherence tomography studies: a report from the International Working Group for Intravascular Optical Coherence Tomography Standardization and Validation. J Am Coll Cardiol 2012;59:1058-72. Erratum in: J Am Coll Cardiol 2012;59:1662. [Crossref] [PubMed]
- Mintz GS. Intravascular ultrasound and outcomes after drug-eluting stent implantation. Coron Artery Dis 2017;28:346-52. [Crossref] [PubMed]
- Mintz GS, Guagliumi G. Intravascular imaging in coronary artery disease. Lancet 2017;390:793-809. [Crossref] [PubMed]
- Räber L, Mintz GS, Koskinas KC, et al. Clinical use of intracoronary imaging. Part 1: guidance and optimization of coronary interventions. An expert consensus document of the European Association of Percutaneous Cardiovascular Interventions. Eur Heart J 2018;39:3281-300. [Crossref] [PubMed]
- Morino Y, Tamiya S, Masuda N, et al. Intravascular ultrasound criteria for determination of optimal longitudinal positioning of sirolimus-eluting stents. Circ J 2010;74:1609-16. [Crossref] [PubMed]
- Wentzel JJ, Gijsen FJH, van der Giessen R, et al. Positive remodeling at 3 year follow up is associated with plaque free coronary wall segment at baseline: A serial IVUS study. Atherosclerosis. 2014;236:82-90. [Crossref] [PubMed]
- Hoogendoorn A, Gnanadesigan M, Zahnd G, et al. OCT-measured plaque free wall angle is indicative for plaque burden: overcoming the main limitation of OCT? Int J Cardiovasc Imaging 2016;32:1477-81. [Crossref] [PubMed]
- Thygesen K, Alpert JS, Jaffe AS, et al. Fourth Universal Definition of Myocardial Infarction (2018). J Am Coll Cardiol 2018;72:2231-64. [Crossref] [PubMed]
- Ryan TJ, Faxon DP, Gunnar RM, et al. Guidelines for percutaneous transluminal coronary angioplasty. A report of the American College of Cardiology/American Heart Association Task Force on Assessment of Diagnostic and Therapeutic Cardiovascular Procedures (Subcommittee on Percutaneous Transluminal Coronary Angioplasty). Circulation 1988;78:486-502. [Crossref] [PubMed]
- Austen WG, Edwards JE, Frye RL, et al. A reporting system on patients evaluated for coronary artery disease. Report of the Ad Hoc Committee for Grading of Coronary Artery Disease, Council on Cardiovascular Surgery, American Heart Association. Circulation 1975;51:5-40. [Crossref] [PubMed]
- The Thrombolysis in Myocardial Infarction (TIMI) trial. Phase I findings. N Engl J Med 1985;312:932-6. [Crossref] [PubMed]
- Rogers JH, Lasala JM. Coronary artery dissection and perforation complicating percutaneous coronary intervention. J Invasive Cardiol 2004;16:493-9.
- Otake H, Kubo T, Takahashi H, et al. Optical Frequency Domain Imaging Versus Intravascular Ultrasound in Percutaneous Coronary Intervention (OPINION Trial): Results From the OPINION Imaging Study. JACC Cardiovasc Imaging 2018;11:111-23. [Crossref] [PubMed]
- Radu MD. The Clinical Atlas of Intravascular Optical Coherence Tomography for iPad. Eur Heart J 2012;33:1174-5. [Crossref] [PubMed]
- Gonzalo N, Serruys PW, Okamura T, et al. Relation between plaque type and dissections at the edges after stent implantation: an optical coherence tomography study. Int J Cardiol 2011;150:151-5. [Crossref] [PubMed]
- Kume T, Okura H, Yamada R, et al. Frequency and spatial distribution of thin-cap fibroatheroma assessed by 3-vessel intravascular ultrasound and optical coherence tomography: an ex vivo validation and an initial in vivo feasibility study. Circ J 2009;73:1086-91. [Crossref] [PubMed]
- Legutko J, Jakala J, Mintz GS, et al. Virtual histology-intravascular ultrasound assessment of lesion coverage after angiographically-guided stent implantation in patients with ST Elevation myocardial infarction undergoing primary percutaneous coronary intervention. Am J Cardiol 2012;109:1405-10. [Crossref] [PubMed]
- Ali ZA, Maehara A, Généreux P, et al. Optical coherence tomography compared with intravascular ultrasound and with angiography to guide coronary stent implantation (ILUMIEN III: OPTIMIZE PCI): a randomised controlled trial. Lancet 2016;388:2618-28. [Crossref] [PubMed]
- Dingli P, Gonzalo N, Escaned J. Intravascular Ultrasound-guided Management of Diffuse Stenosis. Radcliffe Cardiology 2018:1-8.
- Porto I, Di Vito L, Burzotta F, et al. Predictors of periprocedural (type IVa) myocardial infarction, as assessed by frequency-domain optical coherence tomography. Circ Cardiovasc Interv 2012;5:89-96, S1-6.
- Elakabawi K, Huang X, Shah SA, et al. Predictors of suboptimal coronary blood flow after primary angioplasty and its implications on short-term outcomes in patients with acute anterior STEMI. BMC Cardiovasc Disord 2020;20:391. [Crossref] [PubMed]
- Kobayashi N, Mintz GS, Witzenbichler B, et al. Prevalence, Features, and Prognostic Importance of Edge Dissection After Drug-Eluting Stent Implantation. An ADAPT-DES Intravascular Ultrasound Substudy. Circ Cardiovasc Interv. 2016;9:e003553. [Crossref] [PubMed]
- Velican D, Velican C. Comparative study on age-related changes and atherosclerotic involvement of the coronary arteries of male and female subjects up to 40 years of age. Atherosclerosis 1981;38:39-50. [Crossref] [PubMed]
- Kume T, Akasaka T, Kawamoto T, et al. Assessment of coronary intima--media thickness by optical coherence tomography: comparison with intravascular ultrasound. Circ J 2005;69:903-7. [Crossref] [PubMed]
- Arnett EN, Isner JM, Redwood DR, et al. Coronary artery narrowing in coronary heart disease: comparison of cineangiographic and necropsy findings. Ann Intern Med 1979;91:350-6. [Crossref] [PubMed]
- Al-Lamee R, Rajkumar CA, Ganesananthan S, et al. Optimising physiological endpoints of percutaneous coronary intervention. EuroIntervention 2021;16:e1470-83. [Crossref] [PubMed]
- Virmani R, Burke AP, Kolodgie FD, et al. Pathology of the thin-cap fibroatheroma: a type of vulnerable plaque. J Interv Cardiol 2003;16:267-72. [Crossref] [PubMed]
- Jang IK, Tearney GJ, MacNeill B, et al. In vivo characterization of coronary atherosclerotic plaque by use of optical coherence tomography. Circulation 2005;111:1551-5. [Crossref] [PubMed]
- Harrison RW, Aggarwal A, Ou FS, et al. Incidence and outcomes of no-reflow phenomenon during percutaneous coronary intervention among patients with acute myocardial infarction. Am J Cardiol 2013;111:178-84. [Crossref] [PubMed]
- Prati F, Romagnoli E, Biccirè FG, et al. Clinical outcomes of suboptimal stent deployment as assessed by optical coherence tomography: long-term results of the CLI-OPCI registry. EuroIntervention 2022;18:e150-7. [Crossref] [PubMed]
- Ali Z, Landmesser U, Karimi Galougahi K, et al. Optical coherence tomography-guided coronary stent implantation compared to angiography: a multicentre randomised trial in PCI - design and rationale of ILUMIEN IV: OPTIMAL PCI. EuroIntervention 2021;16:1092-9. [Crossref] [PubMed]