Pectus excavatum and carinatum: a narrative review of epidemiology, etiopathogenesis, clinical features, and classification
Introduction
A wide variety of anterior congenital chest wall deformities exists (1,2). Although present at birth in most cases, the deformities often become more prominent during the growth spurt. Anterior chest wall deformities may have cardiopulmonary consequences for which many patients seek (surgical) correction as a diminished cardiopulmonary function interferes with their daily activities (1). In addition, impaired quality of life due to body image disturbances caused by the aesthetic appearance of the deformity is prominent, particularly in the adolescent group, and this alone may dictate (surgical) intervention (3-5).
Pectus excavatum, or “funnel chest”, is the most common anterior chest wall deformity, and is characterized by an inward displacement of the sternum and adjacent costal cartilage (1,2). In contrast, pectus carinatum, the second most common deformity, is characterized by a protrusion of the sternum and costal cartilage. Other deformities in the broad spectrum of congenital chest wall deformities include more miscellaneous deformities like Poland syndrome and cleft sternum (1). Pectus excavatum can be surgically corrected via the classic open Ravitch procedure or minimally invasive repair of pectus excavatum (MIRPE) can be performed via the Nuss procedure. The Nuss procedure is nowadays considered as the treatment of choice as the sternum is remodeled by the placement of a retrosternal bar while the costal cartilages are preserved, and comes with considerably less morbidity, including scarring, when compared to the Ravitch procedure. Pectus carinatum can also be corrected via the Ravitch procedure, but due to surgical morbidity, it is preferred to perform minimally invasive repair of pectus carinatum (MIRPC) via the Abramson procedure. In the Abramson procedure, a presternal correctional bar is implanted and secured to rib-attached stabilizers to redress the sternum. The procedure has undergone several modifications (e.g., pectus carinatum compression system) since it was first described to optimize safety and results (6,7).
Over the years, numerous studies have been conducted, aiming to optimize provided care by better understanding the epidemiology, etiopathogenesis, and clinical features of these deformities. This report aims to provide an overview of what is currently known about the clinical features, epidemiology, etiopathogenesis, and classification of the most common chest wall deformities (i.e., pectus excavatum and carinatum) and highlight the gaps in knowledge. We present this article in accordance with the Narrative Review reporting checklist (available at https://jtd.amegroups.com/article/view/10.21037/jtd-23-957/rc).
Methods
An electronic search was conducted in the PubMed and Embase databases on June 12th, 2023, for each of the following domains: clinical presentation, epidemiology, classification, and etiopathogenesis (Table 1). Furthermore, the search terms “pectus excavatum”, “pectus carinatum”, “congenital chest wall disorder”, and the medical subject headings (MeSH) terms “funnel chest” and “pectus carinatum” were used to identify relevant studies. Titles and abstracts of all articles were screened for eligibility. No publication date restrictions were imposed. Studies deemed irrelevant to the above-mentioned domains, or pectus excavatum or carinatum were excluded. In total, 147 articles were included in this narrative review.
Table 1
Items | Specification |
---|---|
Date of search | June 12th, 2023 |
Databases and other sources searched | PubMed and Embase |
Search terms used | MeSH terms: “funnel chest” and “pectus carinatum” |
Free text search terms: “pectus excavatum”, “pectus carinatum”, “congenital chest wall disorder”, “clinical presentation”, “epidemiology”, “classification”, and “etiopathogenesis” | |
Timeframe | No publication date restrictions were imposed |
Inclusion and exclusion criteria | Inclusion: all studies related to pectus excavatum or carinatum and the above-mentioned domains |
Exclusion: studies deemed irrelevant to the above-mentioned domains, or pectus excavatum or carinatum | |
Selection process | N.J. conducted the literature search and analysis. All authors reviewed the final list of studies included in the review |
MeSH, medical subject headings.
Pectus excavatum
Epidemiology
Pectus excavatum accounts for approximately 65–95% of all chest wall deformities depending on the population being studied (2,8,9). Males are reported to be affected three to five times more often than females. No direct racial predisposition has been observed, although the deformity is more frequently described in Caucasians than among African Americans, Hispanics, or Asians (10,11).
In literature, incidence rates of up to 8 per 1,000 live births have been reported (10,12). However, the true incidence and prevalence of pectus excavatum are not well-established yet and may be much higher than the currently described rates (up to 5% based on radiologic reports), as no large-scale, population-based studies have been conducted (13). Due to the absence of a clear definition of pectus excavatum and the key factor in diagnosing being visual examination, a well-founded estimation of the prevalence of the deformity is challenging (14). The dynamic nature of the deformity, which in most patients becomes more prominent and noticeable during early childhood and adolescence, further complicates determination of the number of cases in a population (15). Furthermore, there is still limited awareness of the deformity among the general population as well as healthcare providers, which may add to underreporting or misdiagnosing the deformity. While the global interest in pectus excavatum is currently higher than in pectus carinatum, which might reflect the real-world prevalence, the global trend in internet searches related to the topic pectus excavatum is decreasing each year (14).
Etiopathogenesis
Over the years, several hypotheses for the development of pectus excavatum have been proposed. The first written account of the deformity dates back to 1596 when Bauhinus [1609] documented his observations and proposed hypertension of the diaphragm during embryonic development as the underlying pathophysiologic mechanism (16). A multitude of hypotheses have been introduced since then, which can be divided into intrinsic or extrinsic underlying pathogenic factors. In the 19th century, Williams [1872] and Langer [1880] attributed the onset of the deformity to intrauterine pressure on the sternum, resulting from abnormal embryo positioning (e.g., compression of the chin or knee) (17,18). Eggel [1870], on the other hand, suggested that nutritional disturbances or developmental failure could lead to weakness of the sternum which ultimately results in a depression (19). During that era, several other hypotheses were postulated highlighting diseases such as syphilis, (fetal) rickets, poliomyelitis, mediastinitis, and upper airway obstructions as the underlying cause (20-24). Others advocated that the deformity is the result of long periods of mechanical stress on the sternum as seen in cobblers who use the anterior part of their chest as a working surface (25,26). They even considered the deformity a professional disease (i.e., cobblers’ chest). However, these hypotheses were not widely supported and disproved by later studies. Curschmann [1936] was the first one to report an association between pectus excavatum and connective tissue disorders (27). In 1936, he described a case of pectus excavatum with concurrent arachnodactyly and ectopia.
Retraction hypothesis
During the first half of the 20th century, the leading theory was retraction of the sternum and adjacent costal cartilage. Retraction was attributed to either direct extensive traction by the diaphragm, or negative pressure in the anterior mediastinum due to left displacement of the heart (28,29). Woillez [1860] proposed a contracture of the central diaphragmatic tendon as the reason for the deformity, and as mentioned before, Bauhinus [1609] already documented observations of a diaphragmatic pull in 1596 (16,30). However, it was only until 1939 that Brown [1939] popularized the diaphragmatic retraction hypothesis by reporting a thickened substernal ligament (31,32). He even presented a surgical procedure (i.e., Brown procedure) in which the diaphragm is released from its sternal attachments (32). This hypothesis was further advanced by Brodkin [1951], who noted an abnormal anterior portion of the diaphragm (33). This part of the diaphragm, which develops from the septum transversum, was deficient in muscle tissue and more tendinous in pectus excavatum patients. He argued that inspiratory contractions of this abnormal part of the diaphragm were the underlying cause of the deformity. He also conducted tests with procaine injections into the phrenic nerve, showing diminished inspiratory retraction of the sternum when the diaphragm was paralyzed (34). Chin [1957] supported Brodkin’s observations by comparing diaphragmatic muscle biopsies from pectus excavatum patients to healthy controls, revealing that muscle tissue was partially replaced by fibrous tissue (35). A further hypothesis was developed by Jackson et al. [1959], who suggested that an imbalance between diaphragmatic and pectoral muscle tension, thus pectoral spasms or weakness, provokes either pectus excavatum or carinatum (36).
Overgrowth theory
In the second half of the 20th century, the diaphragmatic retraction theory was questioned as the procedure proposed by Brown failed to correct the deformity (37-40). In most cases, the point of maximum depression was located more cranially than the diaphragmatic insertion, and no diaphragmatic ligament was visible. Nowadays leading hypotheses focus on overgrowth or growth disturbances of parts of the anterior thoracic wall. In 1873, Flesch [1873] was the first one to mention overgrowth of the ribs as the underlying cause for pectus excavatum (41). He argued that abnormally long ribs, with lateral chest wall fixation, would result in a depression of the sternum. Versé [1910] drew attention to an abnormally long sternum, which would in turn lead to an inward displacement of the sternum (42). More recent studies also revealed an abnormal sternum in pectus excavatum patients when compared to healthy controls in terms of shape and curvature patterns (43-45). Sweet [1944], on the other hand, hypothesized that the deformity was due to an overgrowth of the costal cartilage (40). The costal cartilage overgrowth hypothesis has however been brought into question by the results of studies on costal cartilage lengths measured using three-dimensional (3D)-computed tomography (CT) images (46-49). While the costal cartilage overgrowth hypothesis assumes that costal cartilage lengths are longer at the site of overgrowth, these studies showed an indifferent or even shorter costal cartilage length when compared to the contralateral costal cartilage or the cartilage of healthy individuals. Karakılıç et al. [2018], found no correlation between the costal cartilage length and the severity of the deformity (49). On the contrary, Park et al. [2015] and Kondo et al. [2020] observed longer than normal costal cartilage in pectus excavatum patients, leaving the costal cartilage overgrowth hypothesis a controversial topic (50,51).
Growth disturbance theory
In 1967, Mullard [1967] presented several objections to the diaphragmatic retraction theory and favored a new theory, the growth disturbance theory (52). He pleaded that a growth disturbance of the costal cartilage affects the structure and function of the costosternal junction, resulting in pectus excavatum.
Histologic studies of the costal cartilage have revealed these abnormalities in structure, function, and physical features, but with high variability in findings depending on the component that was analyzed, and the methods used. Geisbe et al. [1967] were the first to publish the results of a histologic analysis on costal cartilage samples from patients who underwent the Ravitch procedure (53). Their findings suggested premature aging with age-dependent degeneration being advanced by 10–12 years, and highly correlating with the severity of the deformity. This raised the question if the found cartilage disturbances were of metabolic origin, or the result of altered mechanical stress (53-55). Later histologic studies focus on the cellular component or extracellular matrix of costal cartilage. Although early studies on the cellular component report disturbances of the chondrocytes, most recent studies state that there are no numerical or structural differences in chondrocytes between pectus excavatum patients and healthy controls (2,56-59). Tocchioni et al. [2013] argued that even if differences in hypolacunar and hyperlacunar zones are found, they are more likely the result of asymmetric loading of the cartilage than being the primary origin (59). However, indifferences in chondrocyte structure do not rule out the involvement of the cellular component in the etiology of pectus deformities as chondrocytes regulate the synthesis and metabolism of the extracellular matrix that they are embedded in.
In contrast to the histological studies on the cellular component, almost all studies on the composition and structure of the extracellular matrix revealed significant disturbances. Alterations in the collagen network were found, which affect the strength, physical integrity, and stability of the cartilage (60-62). The ionic content of the extracellular matrix also plays an important role in the biomechanical properties of the cartilage as it determines the cartilages absorption capacity of water (63). A study on trace elements in the costal cartilage samples of pectus excavatum patients showed decreased levels of zinc, and levels of magnesium and calcium were increased which was observed along with a decreased volume of immobilized water (64). Besides its role in the absorption of water, zinc is also known to enhance cartilage growth and maturation as it is part of many enzymes (65,66). David et al. [2011] found a disturbance in the sulfation pattern of proteoglycans in the extracellular matrix, while these negatively charged proteoglycans normally attract cations and water (57). This might be a reason for the altered ionic content and thus water absorption capacity of the costal cartilage in pectus excavatum patients.
Researchers have expressed divergent views regarding the overgrowth and growth disturbance theories. However, these hypotheses are not necessarily mutually exclusive as both hyperplastic growth and metabolic disturbances resulting in diminished biomechanical properties may play a role in the costal cartilage deformation of pectus excavatum patients.
Genetic predisposition
Genetic predisposition of pectus excavatum is likely to exist, as up to 54% of pectus excavatum patients have a positive family history (67,68). In 1820, Coulson [1820] was the first to propose the role of genetic factors in the development of pectus excavatum (69). Family tree analysis on the inheritance of the deformity shows multiple patterns of inheritance in different family studies. As such, simple Mendelian inheritance was observed for some families, with autosomal recessive, dominant with reduced penetrance, and X-linked genes involved (70). Abnormalities were found on chromosomes 5, 15, and 17, and the long arm of chromosome 18q (71,72). Genes that are possibly involved are GAL3ST4, TINAG, and Gpr126/Adgrg6 (67,68,72-74). GAL3ST4 gene plays a role in glycoprotein sulfation which influences cartilage strength and stability. In an animal model, pectus excavatum and adolescent idiopathic scoliosis were obtained by deleting the Gpr126 gene (75). The direct link between this gene and the pectus deformity has not been established, though it has been proposed that a deficiency in Gpr126 leads to upregulation of the GAL3ST4 gene (57,75). TINAG gene mutations were found via whole-exome sequencing in a family with multiple cases of pectus excavatum (76). TINAG gene is associated with cell development, adhesion, and extracellular matrix formation. However, no direct pathway in which this gene leads to the development of pectus excavatum could be demonstrated.
Nevertheless, in most family studies, a multifactorial inheritance with unknown attributive factors was demonstrated (70). While pectus excavatum is an isolated disease in most cases, in syndromic diseases like Marfan syndrome, the deformity is seen as a clinical manifestation of the affected gene [i.e., fibrillin-1 (FBN1) gene in patients with FBN1-related Marfan syndrome] (77,78). Syndromes that have a considerable overlap with Marfan syndrome in clinical features and are also associated with the development of pectus excavatum or carinatum are Ehlers-Danlos syndrome, Loeys-Dietz syndrome, and Shprintzen-Goldberg syndrome (79-85). Loeys-Dietz syndrome is the result of a heterozygous pathogenic variant in SMAD2, SMAD3, TGFB2, TGFB3, TGFBR1, or TGFBR2, while the SKI gene is affected in Shprintzen-Goldberg syndrome. Over 20 genetic defects have been described in Ehlers-Danlos patients. Even though genetic studies are still in their infancy for isolated pectus excavatum and other chest wall disorders, new techniques such as high throughput sequencing, which make whole genome association studies possible, are promising tools for the future (86).
Clinical presentation
Typically, pectus excavatum patients are slim and tall, with a pot-bellied appearance and forward-drifting shoulders (Figure 1). Often rib flare is seen in conjunction with the deformity. Due to the sternal depression, the sternovertebral distance is decreased (87). In severe cases, leftward displacement of the heart and an imprint of the sternum in the right-ventricle and -atrium are observed. This primarily leads to diastolic dysfunction and associated exercise intolerance. Valvular dysfunction such as mitral valve prolapse is also reported (88,89). Other symptoms such as chest pain and dyspnea which can be a result of anatomic changes are difficult to objectify (2). The deformity and the possible symptoms tend to worsen with age due to the remodeling of the thoracic cage over time. Some patients, conversely, may not experience symptoms until later in life when other causes contribute to cardiopulmonary complaints (e.g., increased cardiac compression due to progressive kyphosis, or in conjunction with chronic obstructive pulmonary disease (COPD) or heart failure due to alternative causes). Furthermore, it is important to note that patients often report no symptoms at rest, but only during exercise (90).
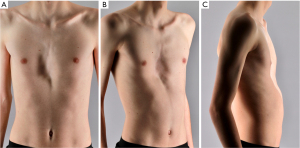
While pectus excavatum is often an isolated anomaly, as mentioned before, it can be associated with other musculoskeletal diseases, particularly scoliosis and connective tissue disorders (e.g., Marfan syndrome, Ehlers-Danlos syndrome) (72,91-93). The deformity has been associated with over 27 genetic conditions and even more genetic defects, which are thoroughly described along with their clinical signs in the reviews by Kotzot [2009] and Billar et al. [2021] (86,94). Recognition of an underlying genetic disorder may be challenging since indicative signs can be subtle or overlooked (e.g., thin skin or extensive bruising). However, the fact that the deformity can be part of a genetic disease is of clinical significance as for example comorbid cardiovascular disease could result in major cardiovascular complications when a surgical correction is performed (e.g., severe bleedings due to vascular fragility). Common cardiovascular comorbidities are aortic-arterial aneurysms and arterial tortuosity which are present in 52–90% of Loeys-Dietz patients. Aortic aneurysm or mitral valve prolapse can be found in a substantial part of Marfan and Ehlers-Danlos patients (94). Referral for genetic counseling should be performed upon suspicion of an underlying genetic condition.
Cardiopulmonary function
Currently, it is still a matter of debate whether cardiopulmonary function in pectus excavatum patients can be improved by surgical repair of the deformity. It has been shown via optoelectronic plethysmography that the Nuss procedure enhances respiratory mechanics, resulting in an increased intrathoracic volume and a decreased reliance on abdominal breathing (95,96). Furthermore, a study by Neviere et al. [2013] demonstrated increased inspiratory muscle strength after repair by the Ravitch procedure with subsequent improvement in oxygen consumption, favoring the theory that surgical repair of pectus excavatum can improve cardiopulmonary function (97).
Regarding static pulmonary function, conflicting evidence is reported. The restrictive ventilatory defect that pectus excavatum patients experience can be evaluated using pulmonary function testing, which is subject to the timing of postoperative measurement, type of surgical repair, and severity of the deformity (98). Studies reporting short-term follow-up outcomes after implantation of the Nuss bar show a worsening in pulmonary function, possibly due to postoperative pain and the restrictive presence of the bars themselves (99,100). Borowitz et al. [2003] provided mid-term (i.e., 6 months) and long-term (i.e., 12 months) outcomes after bar implantation, which showed an improvement in the initial decline in pulmonary function (101). Furthermore, recent large cohort studies demonstrate significant improvement in pulmonary function after bar removal compared to preoperative status (102-106). In contrast, the meta-analysis by Malek et al. [2006] reports no significant improvement in pulmonary function, however, multiple surgical techniques, including the Nuss and Ravitch technique were compiled (107). The open Ravitch procedure has a less favorable outcome regarding pulmonary function than the Nuss procedure, which might be due an increased chest wall rigidity after cartilage removal (108). Also, many of the negative reports on pulmonary function included in this meta-analysis consist of small non-stratified samples.
Improvement in cardiac function for patients with cardiac compression has been reported by numerous large studies using echocardiography and magnetic resonance imaging (MRI) (109-113). An increase in right ventricle volume from 41.1±13 to 54.6±15.6 mL was reported by Jaroszewski et al. [2022] and an increase in cardiac output from 58.4% to 66.2% by Krueger et al. [2010] (114,115). Improvement in cardiac function in pectus excavatum patients ≥30 years of age may be even greater. Furthermore, since most studies were performed with patients in a supine position and at rest, the gain in cardiac function is likely even greater in daily life.
Patients themselves report an improvement in exercise tolerance (3-5,116-118), along with an increased quality of life after surgical repair of the deformity by either the Nuss procedure or Ravitch procedure (119). Quantitative studies evaluating exercise capacity mainly focus on peak oxygen uptake (i.e., VO2max) and oxygen pulse as cardiopulmonary exercise testing measures (98). Unfortunately, the number of studies with samples of more than 20 patients with at least 1-year follow-up is limited (n=6), and only one addresses the adult pectus population (97,105,106,114,120,121). However, all but one of them demonstrated an improvement in oxygen uptake and/or oxygen pulse. The single study reporting a decrease in relative oxygen uptake after the Nuss procedure did not reach statistical significance after correction for kilogram lean body weight (99).
Classification
Congenital chest wall deformities are classified according to the shape of the deformity. This also holds true for pectus excavatum, which is assigned various classification titles based on the aesthetic appearance of the deformity (122,123). While the cup-shaped type features a deep and localized depression, the saucer-shaped type has a shallower and wider concavity. The trench-like title is used to describe a more elongated deformity and the Grand Canyon type refers to an extreme, eccentric canal-like deformity (123,124).
Alternatively, the deformity can be graded to the degree of (a)symmetry. The asymmetric presentation of pectus excavatum is characterized by a sternal rotation and/or uneven height of the sides of the concavity. Asymmetric pectus excavatum may be further subdivided based on the relationship between the center of the sternum and the point of maximum depression, namely: eccentric [i.e., focal, broad-flat, long canal (Grand Canyon type)], unbalanced, or combined (123). However, classification into morphologic subtypes or degree of (a)symmetry is subject to considerable inter- and intra-observer disagreements due to the visual examination aspect (125).
Furthermore, pectus excavatum can be classified according to the severity of the depression. Multiple indexes can be used to do so, with the Haller index being the most widely applied (124). The second-most used index is the pectus correction index (PCI) (126). The Haller index was first described by Haller et al. [1987] and is calculated by dividing the maximum transverse diameter at the deepest point of the deformity by the minimum anteroposterior distance (127). The deepest point of depression is not necessarily related to the sternum and can also be localized around the interchondral joints (128). This index was conventionally obtained from CT. Nowadays, chest radiographs are used to calculate the Haller index as values calculated with both imaging techniques highly correlate (129). Moreover, 3D optical surface imaging can be used to obtain an external Haller index (130). There is also a tool available to semi-automatically calculate classic indexes from MRI images (131).
The Haller index was designed as a tool to determine surgical candidacy, with a Haller index of ≥3.25 qualifying for surgery (87). However, limitations of the Haller index include the absence of a direct relationship with physical or psychological complaints and dependence on the thoracic shape, vertebral level at which it is measured, age, and sex (132). The Haller index was further developed into the PCI by St Peter et al. [2011] (126). In contrast to the Haller index, thorax width is not included in the equation since it has a weak correlation with the depth of the deformity. The PCI is obtained by drawing a horizontal line across the anterior spine. After that, two distances are measured: the minimum distance between the posterior sternum and the anterior spine, and the distance between the inner margin of the anterior portion of the chest and the horizontal line. The last distance is obtained from the same CT slice as chosen for the minimum distance between the posterior sternum and the anterior spine. To generate the PCI, the difference between these two distances is divided by the chest’s maximum prominence, and then multiplied by 100. St Peter et al. stated that a PCI of 10% could be used to define the deformity (126). Another study comparing the PCI to the Haller index reported a strong correlation between both indexes and that a PCI of 28% is equivalent to a Haller index of 3.25 suggesting a surgical threshold (132). However, both indexes lack the consideration of cardiopulmonary compression and morphologic features like (a)symmetry for determining surgical candidacy. The cardiac compression index (CCI) and sternal depression index (SDI) were developed in an attempt to include cardiac compression in the classification of the deformity (133). However, no anatomic index can include subjective symptoms or the physiologic effect of alterations in cardiac shape. Currently, patients’ symptomatology and the presence of cardiopulmonary compression are in most countries leading in the decision to perform surgery instead of an anatomic threshold (134).
Beyond classification of the deformity into different subtypes, recent studies on 3D optical surface imaging provide a base for external quantitative measurements of all morphologic features (i.e., pectus depth, width, length, volume, position, steepness, flaring, asymmetry and mean cross-sectional area) of the deformity (135). Also, models to predict the presence of cardiopulmonary compromise using 3D imaging techniques have been developed enabling classification of the deformity into pectus excavatum with or without cardiopulmonary compression based on morphological features (136). 3D optical surface imaging thus seems promising as it provides both a detailed description of the deformity needed for surgical planning and information on the presence of cardiopulmonary compression without the harmful effects of radiation. However, reference values for all morphological parameters in pectus excavatum patients compared to the normative population need to be established as well as the gap in knowledge on how morphological parameters effect the treatment outcome.
Pectus carinatum
Epidemiology
Pectus carinatum occurs two to four times less frequently than pectus excavatum (137). This is however considerably depending on the population being studied. For example, one study, conducted in Brazil, reported a higher prevalence of pectus carinatum compared to pectus excavatum (0.9% vs. 0.04%) (138). Incidence is estimated at 1 per 2,500 live births, with males having a four-fold incidence compared to females (139). More recent studies using CT, report prevalence rates for pectus carinatum of 2% to 5% for the presence of asymmetrical prominence of costal cartilage (140). Statements made on difficulties with accurately assessing the true incidence of pectus excavatum also hold true for pectus carinatum.
Etiopathogenesis
In contrast to pectus excavatum, literature in this domain on pectus carinatum is scarce. The etiology of pectus carinatum is unknown so far, although it has been suggested to have similar origins as pectus excavatum (i.e., overgrowth or growth disturbances of the costal cartilage) (139). Genetic predisposition through a positive family history of chest wall deformities has also been suggested for the development of pectus carinatum, as this was the case in up to 33% of pectus carinatum patients (8,140).
Clinical presentation
Pectus carinatum, in contrast to pectus excavatum, is characterized by the protrusion of the sternum with possible rib involvement (Figure 2) (1,2,141). Patients with this deformity often present with fewer symptoms compared to their pectus excavatum counterparts. When presenting with symptoms these often pertain to thoracic pain (mainly during sleeping in a prone position), and cosmetic discomfort (139,141). The deformity is also generally mild in childhood and becomes more prominent during adolescence (2). As the deformity is obvious and can be seen through clothing, many teenagers seek correction of the deformity. Conservative external bracing treatment (dynamic or static) is often considered the initial treatment option with success rates exceeding 70% when therapy compliance is high. Pain, skin problems, shame, and discomfort have been shown to add to patient non-compliance, negatively affecting therapy success rates (142). Surgical repair via the Abramson or Ravitch procedure is seen as a subsequent step in the treatment protocol after bracing therapy fails or for patients who are not eligible for non-surgical repair (e.g., in case of a rigid chest wall). A systematic review on aesthetic outcomes after minimally invasive repair by the Abramson procedure reported high success rates defined by patient satisfaction with the aesthetic outcome (143). Improvement of body image disturbances and quality of life after surgical correction are also commonly described (144,145). However, there is a lack of research on the long-term outcomes of pectus carinatum, including the effectiveness of non-surgical and surgical treatment options compared to control groups, recurrence rates, and potential complications.
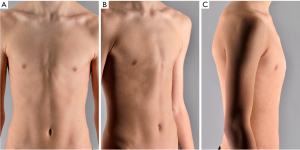
Classification
This deformity involves the entire anterior chest wall and can be subdivided into two principal types: chondromanubrial and chondrogladiolar. The chondrogladiolar type is the most prevalent variant and comprises up to 95% of the cases. It is characterized by an angulation at the sternal corpus with the maximal point of protrusion at middle or lower portion of the sternum (139,141,146). This type of pectus carinatum is usually symmetric and can be associated with the relative lateral depression of the adjacent ribs. The Haller index is also used to describe the severity of this deformity, utilizing the maximum anteroposterior length of the chest wall in the equation instead of the minimum distance between the vertebrae and sternum (146).
While pectus arcuatum, also known as Currarino Silverman syndrome, was previously classified as a pectus carinatum subtype, it is nowadays seen as a separate entity. The deformity is characterized by the protrusion of the junction between the manubrium, and the sternal body and its adjacent ribs. The lower portion of the sternum is relatively short and depressed, and therefore this intriguing deformity is sometimes described as a combined pectus excavatum and carinatum (146,147). The angle of Louise (i.e., the angle between the manubrium and sternal body) is thus less than the normal 145–175 degrees (2).
Conclusions
In the past few decades, substantial progress has been made in understanding the etiopathogenesis of pectus excavatum and carinatum. Many studies have been conducted on epidemiology, classification, and clinical features. However, there are still unchartered domains and considerable gaps in knowledge that must be addressed. This includes confirmation of the current hypotheses on etiopathogenesis by thorough biomedical and genetic studies, and most importantly the establishment of a clear definition and classification system for both deformities. By addressing these gaps in knowledge, we can enhance our understanding of these challenging conditions and further improve patient outcomes.
Acknowledgments
The authors gratefully acknowledge Ernst van Loon and Mirianne Curfs-Theunissen, Medical Photographers (Zuyderland Medical Centre, Heerlen, The Netherlands), for the acquisition of the medical photographs.
Funding: None.
Footnote
Provenance and Peer Review: This article was commissioned by the editorial office, Journal of Thoracic Disease, for the series “Minimally Invasive Treatment of Pectus Deformities”. The article has undergone external peer review.
Reporting Checklist: The authors have completed the Narrative Review reporting checklist. Available at https://jtd.amegroups.com/article/view/10.21037/jtd-23-957/rc
Peer Review File: Available at https://jtd.amegroups.com/article/view/10.21037/jtd-23-957/prf
Conflicts of Interest: All authors have completed the ICMJE uniform disclosure form (available at https://jtd.amegroups.com/article/view/10.21037/jtd-23-957/coif). The series “Minimally Invasive Treatment of Pectus Deformities” was commissioned by the editorial office without any funding or sponsorship. E.R.d.L. and J.H.T.D. served as unpaid Guest Editors of the series. The authors have no other conflicts of interest to declare.
Ethical Statement: The authors are accountable for all aspects of the work in ensuring that questions related to the accuracy or integrity of any part of the work are appropriately investigated and resolved. All clinical procedures described in this study were performed in accordance with the ethical standards of the institutional and/or national research committee(s) and with the Helsinki Declaration (as revised in 2013). Written informed consent was obtained from the patients for the publication of this article and accompanying images.
Open Access Statement: This is an Open Access article distributed in accordance with the Creative Commons Attribution-NonCommercial-NoDerivs 4.0 International License (CC BY-NC-ND 4.0), which permits the non-commercial replication and distribution of the article with the strict proviso that no changes or edits are made and the original work is properly cited (including links to both the formal publication through the relevant DOI and the license). See: https://creativecommons.org/licenses/by-nc-nd/4.0/.
References
- Shamberger RC. Congenital chest wall deformities. Curr Probl Surg 1996;33:469-542. [Crossref] [PubMed]
- Fokin AA, Steuerwald NM, Ahrens WA, et al. Anatomical, histologic, and genetic characteristics of congenital chest wall deformities. Semin Thorac Cardiovasc Surg 2009;21:44-57. [Crossref] [PubMed]
- Kuru P, Bostanci K, Ermerak NO, et al. Quality of life improves after minimally invasive repair of pectus excavatum. Asian Cardiovasc Thorac Ann 2015;23:302-7. [Crossref] [PubMed]
- Jacobsen EB, Thastum M, Jeppesen JH, et al. Health-related quality of life in children and adolescents undergoing surgery for pectus excavatum. Eur J Pediatr Surg 2010;20:85-91. [Crossref] [PubMed]
- Krasopoulos G, Dusmet M, Ladas G, et al. Nuss procedure improves the quality of life in young male adults with pectus excavatum deformity. Eur J Cardiothorac Surg 2006;29:1-5. [Crossref] [PubMed]
- Janssen N, Daemen JHT, Franssen AJPM, et al. Modification of the Abramson procedure for minimally invasive repair of pectus carinatum: introduction of a pectus carinatum compression system. J Thorac Dis 2023;15:4120-9. [Crossref] [PubMed]
- Skrzypczak P, Kasprzyk M, Piwkowski C. The new steel bar in pectus carinatum repair and a review of current methods of correcting chest deformations. J Thorac Dis 2022;14:3671-3. [Crossref] [PubMed]
- Goretsky MJ, Kelly RE Jr, Croitoru D, et al. Chest wall anomalies: pectus excavatum and pectus carinatum. Adolesc Med Clin 2004;15:455-71. [Crossref] [PubMed]
- Westphal FL, Lima LC, Lima Neto JC, et al. Prevalence of pectus carinatum and pectus excavatum in students in the city of Manaus, Brazil. J Bras Pneumol 2009;35:221-6. [Crossref] [PubMed]
- Koumbourlis AC, Stolar CJ. Lung growth and function in children and adolescents with idiopathic pectus excavatum. Pediatr Pulmonol 2004;38:339-43. [Crossref] [PubMed]
- Fonkalsrud EW. Current management of pectus excavatum. World J Surg 2003;27:502-8. [Crossref] [PubMed]
- Kelly RE Jr, Lawson ML, Paidas CN, et al. Pectus excavatum in a 112-year autopsy series: anatomic findings and the effect on survival. J Pediatr Surg 2005;40:1275-8. [Crossref] [PubMed]
- Biavati M, Kozlitina J, Alder AC, et al. Prevalence of pectus excavatum in an adult population-based cohort estimated from radiographic indices of chest wall shape. PLoS One 2020;15:e0232575. [Crossref] [PubMed]
- Skrzypczak P, Kamiński M, Pawlak K, et al. Seasonal interest in pectus excavatum and pectus carinatum: a retrospective analysis of Google Trends data. J Thorac Dis 2021;13:1036-44. [Crossref] [PubMed]
- Nuss D, Obermeyer RJ, Kelly RE Jr. Pectus excavatum from a pediatric surgeon's perspective. Ann Cardiothorac Surg 2016;5:493-500. [Crossref] [PubMed]
- Bauhinus J. Observatio. In: Ioannis Schenckii a Grafenberg. editor. Johannes Observatorium medicarum, rararum, novarum, admirabilium, et montrosarum, liber secundus. Frankfurt: De partibus vitalibus, thorace contentis; 1609:322.
- Williams CT. Congenital malformation of the thorax great depression of the sternum. Trans Path Soc 1872;24:50.
- Langer EZ, Zuckerkandel E. Untersuchungen über den mißbildeten Brustkorb des. Herrn JW Wiener med Zeit 1880;49:515.
- Eggel. Eine seltene Missbildung des thorax. Archiv für Pathologische Anatomie und Physiologie und für Klinische Medicin 1870;49:230-6.
- Kuhns JG. Funnel Chest Deformity. N Engl J Med 1931;204:1077-82.
- Kelley SW. Surgical Diseases of Children: A Modern Treatise on Pediatric Surgery. Ann Surg 1910;51:438.
- Chapman EM, Dill DB, Graybiel A. The decrease in functional capacity of the lungs and heart resulting from deformities of the chest: Pulmonocardiac failure. Medicine 1939;18:167-202.
- Kuzemko JA. Adenoid chest syndrome. Br Med J 1970;3:109. [Crossref] [PubMed]
- Olsen KD, Kern EB, O'Connell EJ. Pectus excavatum: resolution after surgical removal of upper airway obstruction. Laryngoscope 1980;90:832-7.
- Kloetzel K, Cassetari L, Lopeś JA. Pectus excavatum (funnel chest) as an occupational disease. J Occup Med 1973;15:118-9.
- Evans W. The heart in sternal depression. Br Heart J 1946;8:162-70.
- Curschmann H. Über erbliche Arachnodaktylie. Nervenarzt 1936;9:624.
- Wooler GH, Mashhour YA, Garcia JB, et al. Pectus excavatum. Thorax 1969;24:557-62. [Crossref] [PubMed]
- Dor V, Cohen J, Schmitt R, et al. Funnel-chest and cardio-pericardial pathology, apropos of 2 cases treated by pericardioplasty and parietal remodelling. Arch Mal Coeur Vaiss 1975;68:217-23.
- Woillez E. Sur un cas de difformité thoracique considerable avec déplacement inoffensif de plusieurs organes et signes stéthoscopiques particuliers. L’Union Médicale Journal Intérêt Corp Med 1860;6:515-21.
- Brown AL, Cook O. Funnel chest (pectus excavatum) in infancy and adult life. Calif Med 1951;74:174-8.
- Brown AL. Pectus excavatum (funnel chest): anatomic basis; surgical treatment of the incipient stage in infancy; and correction of the deformity in the fully developed stage. J Thorac Surg 1939;9:164-84.
- Brodkin HA. Congenital chondrosternal depression (funnel chest) Its treatment by phrenosternolysis and chondrosternoplasty. Dis Chest 1951;19:288-306. [Crossref] [PubMed]
- Brodkin HA. Congenital anterior chest wall deformities of diaphragmatic origin. Dis Chest 1953;24:259-77. [Crossref] [PubMed]
- Chin EF. Surgery of funnel chest and congenital sternal prominence. Br J Surg 1957;44:360-76. [Crossref] [PubMed]
- Jackson JL, George RE, Hewlett TH, et al. Pectus excavatum: surgical experiences in thirty-four cases. Am J Surg 1959;98:664-76. [Crossref] [PubMed]
- Mahoney EB, Emerson GL. Surgical treatment of the congenital funnel-chest deformity. AMA Arch Surg 1953;67:317-29. [Crossref] [PubMed]
- Lindskog GE, Felton WL 2nd. Considerations in the surgical treatment of pectus excavatum. Ann Surg 1955;142:654-9; discussion, 659-61. [Crossref] [PubMed]
- Kopp CE. The management of pectus excavatum. Surg Clin North Am 1956;1627-37.
- Sweet RH. Pectus Excavatum: Report of Two Cases Successfully Operated Upon. Ann Surg 1944;119:922-34. [Crossref] [PubMed]
- Flesch M. Über eine seltene Missbildung des Thorax. Archiv für Pathologische Anatomie und Physiologie und für Klinische Medicin 1873;57:289-94.
- Versé M. Über die kongenitale Trichterbrust mit besonderer Berücksichtigung der normal-anatomischen Verhältnisse. Beitr Path Anat 1910;48:311-42.
- Haje SA, Harcke HT, Bowen JR. Growth disturbance of the sternum and pectus deformities: imaging studies and clinical correlation. Pediatr Radiol 1999;29:334-41. [Crossref] [PubMed]
- Lee C, Zavala-Garcia A, Teekappanavar N, et al. Measurement of sternal curvature angle on patients with pectus excavatum. Pediatr Surg Int 2017;33:65-7. [Crossref] [PubMed]
- Haje DP, Teixeira KO, Silva M, et al. Analysis of sternal curvature patterns in patients with pectus and control. Acta Ortop Bras 2021;29:258-62. [Crossref] [PubMed]
- Eisinger RS, Harris T, Rajderkar DA, et al. Against the Overgrowth Hypothesis: Shorter Costal Cartilage Lengths in Pectus Excavatum. J Surg Res 2019;235:93-7. [Crossref] [PubMed]
- Nakaoka T, Uemura S, Yano T, et al. Does overgrowth of costal cartilage cause pectus excavatum? A study on the lengths of ribs and costal cartilages in asymmetric patients. J Pediatr Surg 2009;44:1333-6. [Crossref] [PubMed]
- Nakaoka T, Uemura S, Yoshida T, et al. Overgrowth of costal cartilage is not the etiology of pectus excavatum. J Pediatr Surg 2010;45:2015-8. [Crossref] [PubMed]
- Karakılıç A, Karaçam V, Ersöz H, et al. Determination of severity of deformity with rib length to costal cartilage length ratio in thorax deformities. Turk Gogus Kalp Damar Cerrahisi Derg 2018;26:279-85. [Crossref] [PubMed]
- Park CH, Kim TH, Haam SJ, et al. Rib overgrowth may be a contributing factor for pectus excavatum: Evaluation of prepubertal patients younger than 10 years old. J Pediatr Surg 2015;50:1945-8. [Crossref] [PubMed]
- Kondo S, Takagi D, Osaga S, et al. The costochondral length in patients with pectus excavatum is longer than that of the normal thorax. Pediatr Surg Int 2020;36:305-16. [Crossref] [PubMed]
- Mullard K. Observations on the aetiology of pectus excavatum and other chest deformities, and a method of recording them. Br J Surg 1967;54:115-20. [Crossref] [PubMed]
- Geisbe H, Buddecke E, Flach A, et al. 88. Biochemical, morphological and physical as well as animal experimental studies on the pathogenesis of funnel chest. Langenbecks Arch Chir 1967;319:536-41. [Crossref] [PubMed]
- Geisbe H, Mildenberger H, Flach A, et al. The aetiology and pathogenesis of funnel chest. Prog Pediatr Surg 1971;3:13-26.
- Müller G, Flach A, Geisbe H. Morphological studies on resected rib cartilage in funnel chest. Frankf Z Pathol 1967;76:164-78.
- Serafin J, Swiatkowski J, Majkusiak R, et al. 40-year experience in surgical treatment of congenital chest deformations--ethiopathogenesis, operative techniques and clinical results. Acta Chir Orthop Traumatol Cech 2003;70:207-13.
- David VL, Izvernariu DA, Popoiu CM, et al. Morphologic, morphometrical and histochemical proprieties of the costal cartilage in children with pectus excavatum. Rom J Morphol Embryol 2011;52:625-9.
- Borisova NV, Pokrovskaya AYa, Zakharova EYa, et al. Analysis of collagen hydroxypyridinium crosslinks in samples of tissues and urine of patients with inherited connective tissue disorders. Connect Tissue Res 1994;30:177-90. [Crossref] [PubMed]
- Tocchioni F, Ghionzoli M, Calosi L, et al. Rib cartilage characterization in patients affected by pectus excavatum. Anat Rec (Hoboken) 2013;296:1813-20. [Crossref] [PubMed]
- Kulik IO, Plyakin VA, Sarukhanyan OO, et al. Etiology and pathogenesis of pectus excavatum in children. Traumatology and Orthopedics of Russia 2013;19:136-41.
- Borisova NV, Pokrovskaya AY, Zakharova EY, et al. Analysis of hydroxypyridine cross-links in human costal cartilage collagen. Bull Exp Biol Med 1993;115:566-9.
- Feng J, Hu T, Liu W, et al. The biomechanical, morphologic, and histochemical properties of the costal cartilages in children with pectus excavatum. J Pediatr Surg 2001;36:1770-6. [Crossref] [PubMed]
- Hall AC, Horwitz ER, Wilkins RJ. The cellular physiology of articular cartilage. Exp Physiol 1996;81:535-45. [Crossref] [PubMed]
- Rupprecht H, Hümmer HP, Stöss H, et al. Pathogenesis of chest wall abnormalities--electron microscopy studies and trace element analysis of rib cartilage. Z Kinderchir 1987;42:228-9. [Crossref] [PubMed]
- O'Dell BL, Newberne PM, Savage JE. Significance of dietary zinc for the growing chicken. J Nutr 1958;65:503-18. [Crossref] [PubMed]
- Hurley LS, Swenerton H. Congenital malformations resulting from zinc deficiency in rats. Proc Soc Exp Biol Med 1966;123:692-6. [Crossref] [PubMed]
- Kloth K, Klohs S, Bhullar J, et al. The Epidemiology behind Pectus Excavatum: Clinical Study and Review of the Literature. Eur J Pediatr Surg 2022;32:316-20.
- David VL. Current Concepts in the Etiology and Pathogenesis of Pectus Excavatum in Humans-A Systematic Review. J Clin Med 2022;11:1241. [Crossref] [PubMed]
- Coulson W. Deformities of the chest. Lond Med Gaz 1820;4:69-73.
- Creswick HA, Stacey MW, Kelly RE Jr, et al. Family study of the inheritance of pectus excavatum. J Pediatr Surg 2006;41:1699-703. [Crossref] [PubMed]
- Horth L, Stacey MW, Proud VK, et al. Advancing our understanding of the inheritance and transmission of pectus excavatum. J Pediatr Genet 2012;1:161-73. [Crossref] [PubMed]
- Gurnett CA, Alaee F, Bowcock A, et al. Genetic linkage localizes an adolescent idiopathic scoliosis and pectus excavatum gene to chromosome 18 q. Spine (Phila Pa 1976) 2009;34:E94-100. [Crossref] [PubMed]
- Wu S, Sun X, Zhu W, et al. Evidence for GAL3ST4 mutation as the potential cause of pectus excavatum. Cell Res 2012;22:1712-5. [Crossref] [PubMed]
- Karner CM, Long F, Solnica-Krezel L, et al. Gpr126/Adgrg6 deletion in cartilage models idiopathic scoliosis and pectus excavatum in mice. Hum Mol Genet 2015;24:4365-73. [Crossref] [PubMed]
- Heinegård D, Oldberg A. Structure and biology of cartilage and bone matrix noncollagenous macromolecules. FASEB J 1989;3:2042-51. [Crossref] [PubMed]
- Tong X, Li G, Feng Y. TINAG mutation as a genetic cause of pectus excavatum. Med Hypotheses 2020;137:109557. [Crossref] [PubMed]
- Loeys BL, Dietz HC, Braverman AC, et al. The revised Ghent nosology for the Marfan syndrome. J Med Genet 2010;47:476-85. [Crossref] [PubMed]
- Dietz H. FBN1-Related Marfan Syndrome. In: Adam MP, Mirzaa GM, Pagon RA, et al. editors. GeneReviews®. Seattle: University of Washington; 2001.
- Carmignac V, Thevenon J, Adès L, et al. In-frame mutations in exon 1 of SKI cause dominant Shprintzen-Goldberg syndrome. Am J Hum Genet 2012;91:950-7. [Crossref] [PubMed]
- Loeys BL, Schwarze U, Holm T, et al. Aneurysm syndromes caused by mutations in the TGF-beta receptor. N Engl J Med 2006;355:788-98. [Crossref] [PubMed]
- van de Laar IM, Oldenburg RA, Pals G, et al. Mutations in SMAD3 cause a syndromic form of aortic aneurysms and dissections with early-onset osteoarthritis. Nat Genet 2011;43:121-6. [Crossref] [PubMed]
- Lindsay ME, Schepers D, Bolar NA, et al. Loss-of-function mutations in TGFB2 cause a syndromic presentation of thoracic aortic aneurysm. Nat Genet 2012;44:922-7. [Crossref] [PubMed]
- Cannaerts E, Kempers M, Maugeri A, et al. Novel pathogenic SMAD2 variants in five families with arterial aneurysm and dissection: further delineation of the phenotype. J Med Genet 2019;56:220-7. [Crossref] [PubMed]
- Bertoli-Avella AM, Gillis E, Morisaki H, et al. Mutations in a TGF-β ligand, TGFB3, cause syndromic aortic aneurysms and dissections. J Am Coll Cardiol 2015;65:1324-36. [Crossref] [PubMed]
- Malfait F, Francomano C, Byers P, et al. The 2017 international classification of the Ehlers-Danlos syndromes. Am J Med Genet C Semin Med Genet 2017;175:8-26. [Crossref] [PubMed]
- Kotzot D, Schwabegger AH. Etiology of chest wall deformities--a genetic review for the treating physician. J Pediatr Surg 2009;44:2004-11. [Crossref] [PubMed]
- Kelly RE Jr. Pectus excavatum: historical background, clinical picture, preoperative evaluation and criteria for operation. Semin Pediatr Surg 2008;17:181-93. [Crossref] [PubMed]
- Coln E, Carrasco J, Coln D. Demonstrating relief of cardiac compression with the Nuss minimally invasive repair for pectus excavatum. J Pediatr Surg 2006;41:683-6; discussion 683-6. [Crossref] [PubMed]
- Crossland DS, Auldist AW, Davis AM. Malignant pectus excavatum. Heart 2006;92:1511. [Crossref] [PubMed]
- Fokin AA. Cleft sternum and sternal foramen. Chest Surg Clin N Am 2000;10:261-76.
- Hong JY, Suh SW, Park HJ, et al. Correlations of adolescent idiopathic scoliosis and pectus excavatum. J Pediatr Orthop 2011;31:870-4. [Crossref] [PubMed]
- Cobben JM, Oostra RJ, van Dijk FS. Pectus excavatum and carinatum. Eur J Med Genet 2014;57:414-7. [Crossref] [PubMed]
- van Es LJM, van Royen BJ, Oomen MWN. Clinical significance of concomitant pectus deformity and adolescent idiopathic scoliosis: systematic review with best evidence synthesis. N Am Spine Soc J 2022;11:100140. [Crossref] [PubMed]
- Billar RJ, Manoubi W, Kant SG, et al. Association between pectus excavatum and congenital genetic disorders: A systematic review and practical guide for the treating physician. J Pediatr Surg 2021;56:2239-52. [Crossref] [PubMed]
- Redlinger RE Jr, Kelly RE, Nuss D, et al. Regional chest wall motion dysfunction in patients with pectus excavatum demonstrated via optoelectronic plethysmography. J Pediatr Surg 2011;46:1172-6. [Crossref] [PubMed]
- Redlinger RE Jr, Wootton A, Kelly RE, et al. Optoelectronic plethysmography demonstrates abrogation of regional chest wall motion dysfunction in patients with pectus excavatum after Nuss repair. J Pediatr Surg 2012;47:160-4. [Crossref] [PubMed]
- Neviere R, Benhamed L, Duva Pentiah A, et al. Pectus excavatum repair improves respiratory pump efficacy and cardiovascular function at exercise. J Thorac Cardiovasc Surg 2013;145:605-6. [Crossref] [PubMed]
- Jaroszewski DE, Velazco CS, Pulivarthi VSKK, et al. Cardiopulmonary Function in Thoracic Wall Deformities: What Do We Really Know? Eur J Pediatr Surg 2018;28:327-46. [Crossref] [PubMed]
- Castellani C, Windhaber J, Schober PH, et al. Exercise performance testing in patients with pectus excavatum before and after Nuss procedure. Pediatr Surg Int 2010;26:659-63. [Crossref] [PubMed]
- Bawazir OA, Montgomery M, Harder J, et al. Midterm evaluation of cardiopulmonary effects of closed repair for pectus excavatum. J Pediatr Surg 2005;40:863-7. [Crossref] [PubMed]
- Borowitz D, Cerny F, Zallen G, et al. Pulmonary function and exercise response in patients with pectus excavatum after Nuss repair. J Pediatr Surg 2003;38:544-7. [Crossref] [PubMed]
- Lawson ML, Mellins RB, Tabangin M, et al. Impact of pectus excavatum on pulmonary function before and after repair with the Nuss procedure. J Pediatr Surg 2005;40:174-80. [Crossref] [PubMed]
- Kelly RE Jr, Mellins RB, Shamberger RC, et al. Multicenter study of pectus excavatum, final report: complications, static/exercise pulmonary function, and anatomic outcomes. J Am Coll Surg 2013;217:1080-9. [Crossref] [PubMed]
- Kelly RE, Goretsky MJ, Obermeyer R, et al. Twenty-one years of experience with minimally invasive repair of pectus excavatum by the Nuss procedure in 1215 patients. Ann Surg 2010;252:1072-81. [Crossref] [PubMed]
- O'Keefe J, Byrne R, Montgomery M, et al. Longer term effects of closed repair of pectus excavatum on cardiopulmonary status. J Pediatr Surg 2013;48:1049-54. [Crossref] [PubMed]
- Maagaard M, Tang M, Ringgaard S, et al. Normalized cardiopulmonary exercise function in patients with pectus excavatum three years after operation. Ann Thorac Surg 2013;96:272-8. [Crossref] [PubMed]
- Malek MH, Berger DE, Marelich WD, et al. Pulmonary function following surgical repair of pectus excavatum: a meta-analysis. Eur J Cardiothorac Surg 2006;30:637-43. [Crossref] [PubMed]
- Johnson JN, Hartman TK, Pianosi PT, et al. Cardiorespiratory function after operation for pectus excavatum. J Pediatr 2008;153:359-64. [Crossref] [PubMed]
- Jaroszewski DE, Ewais MM, Chao CJ, et al. Success of Minimally Invasive Pectus Excavatum Procedures (Modified Nuss) in Adult Patients (≥30 Years). Ann Thorac Surg 2016;102:993-1003. [Crossref] [PubMed]
- Neviere R, Montaigne D, Benhamed L, et al. Cardiopulmonary response following surgical repair of pectus excavatum in adult patients. Eur J Cardiothorac Surg 2011;40:e77-82. [Crossref] [PubMed]
- Chao CJ, Jaroszewski D, Gotway M, et al. Effects of Pectus Excavatum Repair on Right and Left Ventricular Strain. Ann Thorac Surg 2018;105:294-301. [Crossref] [PubMed]
- Chao CJ, Jaroszewski DE, Kumar PN, et al. Surgical repair of pectus excavatum relieves right heart chamber compression and improves cardiac output in adult patients--an intraoperative transesophageal echocardiographic study. Am J Surg 2015;210:1118-24; discussion 1124-5. [Crossref] [PubMed]
- Töpper A, Polleichtner S, Zagrosek A, et al. Impact of surgical correction of pectus excavatum on cardiac function: insights on the right ventricle. A cardiovascular magnetic resonance study†. Interact Cardiovasc Thorac Surg 2016;22:38-46. [Crossref] [PubMed]
- Jaroszewski DE, Farina JM, Gotway MB, et al. Cardiopulmonary Outcomes After the Nuss Procedure in Pectus Excavatum. J Am Heart Assoc 2022;11:e022149. [Crossref] [PubMed]
- Krueger T, Chassot PG, Christodoulou M, et al. Cardiac function assessed by transesophageal echocardiography during pectus excavatum repair. Ann Thorac Surg 2010;89:240-3. [Crossref] [PubMed]
- Kim HK, Shim JH, Choi KS, et al. The quality of life after bar removal in patients after the nuss procedure for pectus excavatum. World J Surg 2011;35:1656-61. [Crossref] [PubMed]
- Roberts J, Hayashi A, Anderson JO, et al. Quality of life of patients who have undergone the Nuss procedure for pectus excavatum: Preliminary findings. J Pediatr Surg 2003;38:779-83. [Crossref] [PubMed]
- Lawson ML, Cash TF, Akers R, et al. A pilot study of the impact of surgical repair on disease-specific quality of life among patients with pectus excavatum. J Pediatr Surg 2003;38:916-8. [Crossref] [PubMed]
- Lam MW, Klassen AF, Montgomery CJ, et al. Quality-of-life outcomes after surgical correction of pectus excavatum: a comparison of the Ravitch and Nuss procedures. J Pediatr Surg 2008;43:819-25. [Crossref] [PubMed]
- Sigalet DL, Montgomery M, Harder J. Cardiopulmonary effects of closed repair of pectus excavatum. J Pediatr Surg 2003;38:380-5; discussion 380-5. [Crossref] [PubMed]
- Das BB, Recto MR, Yeh T. Improvement of cardiopulmonary function after minimally invasive surgical repair of pectus excavatum (Nuss procedure) in children. Ann Pediatr Cardiol 2019;12:77-82. [Crossref] [PubMed]
- Kelly RE Jr, Quinn A, Varela P, et al. Dysmorphology of chest wall deformities: frequency distribution of subtypes of typical pectus excavatum and rare subtypes. Arch Bronconeumol 2013;49:196-200. [Crossref] [PubMed]
- Park HJ, Lee IS, Kim KT. Extreme eccentric canal type pectus excavatum: morphological study and repair techniques. Eur J Cardiothorac Surg 2008;34:150-4. [Crossref] [PubMed]
- Park HJ, Lee SY, Lee CS, et al. The Nuss procedure for pectus excavatum: evolution of techniques and early results on 322 patients. Ann Thorac Surg 2004;77:289-95. [Crossref] [PubMed]
- Daemen JHT, de Loos ER, Geraedts TCM, et al. Visual diagnosis of pectus excavatum: An inter-observer and intra-observer agreement analysis. J Pediatr Surg 2022;57:526-31. [Crossref] [PubMed]
- St Peter SD, Juang D, Garey CL, et al. A novel measure for pectus excavatum: the correction index. J Pediatr Surg 2011;46:2270-3. [Crossref] [PubMed]
- Haller JA Jr, Kramer SS, Lietman SA. Use of CT scans in selection of patients for pectus excavatum surgery: a preliminary report. J Pediatr Surg 1987;22:904-6. [Crossref] [PubMed]
- Rodríguez-Granillo GA, Martínez-Ferro M, Capuñay C, et al. Preoperative multimodality imaging of pectus excavatum: State of the art review and call for standardization. Eur J Radiol 2019;117:140-8. [Crossref] [PubMed]
- Khanna G, Jaju A, Don S, et al. Comparison of Haller index values calculated with chest radiographs versus CT for pectus excavatum evaluation. Pediatr Radiol 2010;40:1763-7. [Crossref] [PubMed]
- Daemen JHT, Loonen TGJ, Lozekoot PWJ, et al. Optical imaging versus CT and plain radiography to quantify pectus severity: a systematic review and meta-analysis. J Thorac Dis 2020;12:1475-87. [Crossref] [PubMed]
- Trò R, Martini S, Stagnaro N, et al. A new tool for assessing Pectus Excavatum by a semi-automatic image processing pipeline calculating the classical severity indexes and a new marker: the Volumetric Correction Index. BMC Med Imaging 2022;22:30. [Crossref] [PubMed]
- Sujka JA, St Peter SD. Quantification of pectus excavatum: Anatomic indices. Semin Pediatr Surg 2018;27:122-6. [Crossref] [PubMed]
- Poston PM, Patel SS, Rajput M, et al. The correction index: setting the standard for recommending operative repair of pectus excavatum. Ann Thorac Surg 2014;97:1176-9; discussion 1179-80. [Crossref] [PubMed]
- Janssen N, Daemen JHT, van Polen EJ, et al. Pectus Excavatum: Consensus and Controversies in Clinical Practice. Ann Thorac Surg 2023;116:191-9. [Crossref] [PubMed]
- Coorens NA, Daemen JHT, Slump CH, et al. The Automatic Quantification of Morphological Features of Pectus Excavatum Based on Three-Dimensional Images. Semin Thorac Cardiovasc Surg 2022;34:772-81. [Crossref] [PubMed]
- Daemen JHT, Heuts S, Rezazadah Ardabili A, et al. Development of Prediction Models for Cardiac Compression in Pectus Excavatum Based on Three-Dimensional Surface Images. Semin Thorac Cardiovasc Surg 2023;35:202-12. [Crossref] [PubMed]
- Desmarais TJ, Keller MS. Pectus carinatum. Curr Opin Pediatr 2013;25:375-81. [Crossref] [PubMed]
- Haje DP, Haje SA, Simioni MA. Prevalência das deformidades pectus carinatum e pectus excavatum em escolares do Distrito Federal. Brasília Med 2002;39:10-5.
- Blanco FC, Elliott ST, Sandler AD. Management of congenital chest wall deformities. Semin Plast Surg 2011;25:107-16. [Crossref] [PubMed]
- Robicsek F, Watts LT. Pectus carinatum. Thorac Surg Clin 2010;20:563-74. [Crossref] [PubMed]
- Martinez-Ferro M, Bellia-Munzon G, Schewitz IA, et al. Pectus carinatum: When less is more. Afr J Thorac Crit Care Med 2019; [Crossref]
- Kang DY, Jung J, Chung S, et al. Factors affecting patient compliance with compressive brace therapy for pectus carinatum. Interact Cardiovasc Thorac Surg 2014;19:900-3. [Crossref] [PubMed]
- Geraedts TCM, Daemen JHT, Vissers YLJ, et al. Minimally invasive repair of pectus carinatum by the Abramson method: A systematic review. J Pediatr Surg 2022;57:325-32. [Crossref] [PubMed]
- Knudsen MV, Grosen K, Pilegaard HK, et al. Surgical correction of pectus carinatum improves perceived body image, mental health and self-esteem. J Pediatr Surg 2015;50:1472-6. [Crossref] [PubMed]
- Bostanci K, Ozalper MH, Eldem B, et al. Quality of life of patients who have undergone the minimally invasive repair of pectus carinatum. Eur J Cardiothorac Surg 2013;43:122-6. [Crossref] [PubMed]
- Torre M, Palo F. Pectus Carinatum. In: Mattioli G, Petralia P, Torre M. editors. Pediatric Thoracic Surgery. Cham: Springer International Publishing; 2021:97-102.
- Martinez-Ferro M, Bellia Munzon G, Fraire C, et al. Non-surgical treatment of pectus carinatum with the FMF(®) Dynamic Compressor System. J Vis Surg 2016;2:57. [Crossref] [PubMed]