VE/VCO2 slope threshold optimization for preoperative evaluation in lung cancer surgery: identifying true high- and low-risk groups
Highlight box
Key findings
• Risk stratification based on three risk groups from preoperative VE/VCO2-slope (the slope of the increase in minute ventilation in relation to carbon dioxide elimination) can be used to identify patients at particularly high or low risk of major pulmonary complications after lung cancer surgery.
What is known and what is new?
• Traditionally, when using one threshold, cardiopulmonary exercise testing (CPET) is known for its strength in identifying patients with a high functional reserve who are at low risk of sustaining a serious complication. Previous studies have seldom reached clinically meaningful positive predictive values, which has been described as one of the major limitations in preoperative risk evaluation using CPET.
• In this study, we found a high specificity threshold (VE/VCO2 slope >41) with a positive predictive value of 47%.
What is the implication, and what should change now?
• Patients presenting with a VE/VCO2 slope above 41 during preoperative CPET should be considered at particularly high risk of complications and may require additional perioperative care.
Introduction
Background
Cardiopulmonary exercise testing (CPET) is the golden standard for functional evaluation before major pulmonary resection (1,2). In addition to exercise capacity (peak oxygen uptake, peak VO2), CPET enables measurement of ventilatory parameters such as the slope of the increase in minute ventilation in relation to carbon dioxide elimination (VE/VCO2 slope) (3). Several studies have shown that the VE/VCO2 slope may be a stronger marker for postoperative complications and mortality compared to peak VO2 (4-6). Most of these studies use a cut-off of 35 to identify high-risk patients (4,7). However, that threshold for adverse outcome is generated from historical data in heart failure patients (8,9). More recent studies have used receiver operating curve (ROC) analysis to more stringently identify the best single threshold, taking into account both sensitivity and specificity (5). However, using a single threshold for risk stratification is challenging, and previous research has in general shown acceptable negative predictive values (NPVs), but low positive predictive values (PPVs) (10).
Rationale and knowledge gap
International guidelines on lung cancer surgery recommend stratifying patients into three risk groups, using two thresholds for peak VO2: 10 and 20 mL/kg/min, respectively (2). This approach, compared to using only one threshold as has been the case for the VE/VCO2 slope, identifies a true high-risk and a true low-risk group with more certainty. It is reasonable, but not yet studied, that three rather than two strata would be beneficial in the evaluation of postoperative risk predictions when using the VE/VCO2 slope.
Objective
Therefore, the purpose of this study was to identify optimal thresholds for the VE/VCO2 slope to risk stratify postoperative pulmonary complications or death after major lung resection. Our hypothesis was that risk stratification into three risk groups based on two thresholds corresponding to either 90% sensitivity or 90% specificity for the main outcome, would generate better discrimination of the risk of complications compared to using a single threshold from either a ROC analysis or the traditional threshold value of 35. We present this article in accordance with the STROBE reporting checklist (available at https://jtd.amegroups.com/article/view/10.21037/jtd-23-1292/rc) (11).
Methods
Participants and setting
This was a single-center retrospective cohort study including all patients with lung cancer who underwent lobectomy or pneumonectomy and a preoperative CPET during the years 2008–2020 at Linköping University Hospital, Linköping, Sweden. Ethical permission was granted by the Swedish Ethical Review Authority (Dnr 2020-03375, 2020-05284, 2021-00543). The study was conducted in accordance with the Declaration of Helsinki (as revised in 2013).
Patient and public involvement
Patients were not involved in the design, recruitment or conduct of the study. Informed consent was waived for this retrospective, de-identified analysis.
Cardiopulmonary exercise test
A maximal cycle ergometer CPET was performed, including 5 minutes of warm-up at 10–50 watts (W), followed by a continuous incremental ramp protocol of 10–20 W/min (eBike Basic, GE Medical Systems, GmbH, Freiburg, Germany). Loads were individualized for each patient including the warm-up and incremental part, aiming to reach exhaustion after 8 to 12 minutes of exercise. Systolic blood pressure, ECG (Marquette CASE 8000, GE Medical Systems, Milwaukee, WI, USA), rating of perceived chest pain, exertion (Borg Rating of Perceived Exertion scale) and dyspnea (Borg CR-10 scale) was monitored (12).
Gas exchange and ventilatory variables were analyzed on a breath-by-breath basis (Jaeger Oxycon Pro or Vyntus CPX, Viasys Healthcare, Hoechberg, Germany) with a system calibrated prior to each test. Oxygen uptake (VO2), carbon dioxide elimination (VCO2) and minute ventilation (VE) were presented numerically as 10-s means, excluding breaths with the highest and lowest values. The VE/VCO2 slope was measured manually by correcting the proposed regression line within software (Sentry Suite 3.10, CareFusion GmbH, Heidelberg, Germany), and was determined as the slope of the VE/VCO2 curve, confined to the linear part up until the respiratory compensation point (13). Peak VO2 was presented indexed by body mass (mL/kg/min) and defined as the average of the two highest consecutive 10-second mean VO2 intervals at or close to the end of the exercise.
Pulmonary function testing
Preoperative pulmonary function testing is presented as crude values as well as percentages of predicted values (pp) (14,15) and includes: forced expiratory volume during the first second (FEV1), forced vital capacity (FVC), total lung capacity, residual volume and carbon monoxide lung diffusion capacity corrected for hemoglobin (DLCOc).
Outcome registration and definitions
The primary outcome used in this study was major pulmonary complication (MPC) or death within 30 days from surgery, where MPC was defined as any of pneumonia, pulmonary embolus, empyema, delayed extubation (not able to extubate in the operation room directly after surgery), reintubation, re-operation, acute respiratory distress syndrome, respiratory insufficiency or pulmonary edema. The definitions of complications and patient comorbidities harmonize with recent international recommendations (16).
Patient categorization
Using the preoperative value of the VE/VCO2 slope, patients were first categorized using two different single threshold approaches; the traditional threshold of 35 and the highest Youden value from the ROC analysis. Secondly, patients were categorized into three risk groups using two thresholds. These two thresholds were determined in a ROC analysis, where the VE/VCO2 slope values generating either a 90% sensitivity (lower threshold) or a 90% specificity (upper threshold) for the main outcome were chosen.
Three separate databases were cross-linked with data from the CPET database by use of the unique Swedish social security number. To retrieve data on in-hospital complications, comorbidities, operation code and surgical technique [open approach or minimally invasive thoracic surgery (MITS)], the Swedish National Quality Register for General Thoracic Surgery (17) was used. Second, data was then cross-linked with The Swedish National Patient Register (18), containing all in-patient and out-patient hospital diagnoses of each Swedish citizen. Finally, to determine survival status and date of death, the Swedish Cause of Death Register was used (18).
Statistics
Statistical analyses were performed with SPSS 23.0.0.2 (SPSS Inc., Chicago, IL, USA). Database management and cross-linking were performed using R Studio v1.1.456 (R Studio Inc, Vienna, Austria). Proportions were compared with the Chi2-test and mean values using the independent t-test. All tests were two-sided and the significance level was set to P<0.05.
The different thresholds for the VE/VCO2 slope were evaluated by their corresponding level of sensitivity, specificity, PPV, NPV, positive likelihood ratio (LR+) and negative likelihood ratio (LR−) for the main outcome. The area under the curve (AUC) from the ROC analysis was determined and presented with a 95% confidence interval (CI).
Logistic regression was used to determine the odds ratio (OR) with the 95% CI for the primary outcome, based on the different methods of categorizing the preoperative VE/VCO2 slope. Analyses were performed unadjusted as well as adjusted for age, sex, lobectomy/pneumonectomy, surgical technique (open approach or MITS), chronic obstructive pulmonary disease (COPD), and percent predicted total lung capacity.
We performed two sensitivity analyses. First, we only included patients with moderate levels of peak VO2 defined as 10–20 mL/kg/min, since the VE/VCO2 slope has previously mainly been used for risk stratification in this group of patients (19). Second, we included only patients undergoing lobectomy or bilobectomy, thus excluding patients undergoing pneumonectomy.
Results
A total of 158 patients [75 men (47.5%), mean age 70.1±7.6 years] with a pathological-anatomical diagnosis of lung cancer were included. All had undergone a maximal effort CPET prior to lobectomy (n=138, including 10 bilobectomies) or pneumonectomy (n=20), and had available data on peak VO2 and the VE/VCO2 slope. The mean value (and range) for peak VO2, VE/VCO2 slope and ppFEV1 was 18±4 mL/kg/min (range, 11–30 mL/kg/min), 34±6 (range, 19–58) and 76%±19% (range, 30–124%), respectively. Open approach and MITS techniques were used in 143 (90.5%) vs. 15 (9.5%) of patients, respectively. No difference was found in frequency of primary outcome based on these two surgical techniques (20% vs. 16%, P=0.70). No patient was treated with robotic-assisted thoracoscopic surgery. The basic characteristics of the study cohort are presented in Table 1.
Table 1
Characteristics | All patients (N=158) |
---|---|
Basic characteristics, mean [SD] | |
Age, years | 70.1 [7.6] |
Height, cm | 169.3 [9.2] |
Weight, kg | 75.5 [15.9] |
Body mass index, kg/m2 | 26.3 [4.8] |
Spirometry, mean [SD] | |
FEV1, L/min | 2.1 [0.6] |
ppFEV1, % | 76 [19] |
VC, L | 3.4 [0.9] |
ppFVC, % | 72 [21] |
FEV1/VC | 0.6 [0.1] |
DLCOc, mmol/min/kPa† | 5.6 [1.6] |
ppDLCOc, %† | 78 [19] |
TLC, L‡ | 6.2 [1.3] |
ppTLC, %‡ | 98 [15] |
RV, L† | 2.7 [0.8] |
ppRV, %† | 115 [34] |
Comorbidity, N [%] | |
Coronary artery disease | 21 [13] |
Previous cardiac surgery | 13 [8] |
Previous cerebrovascular insult | 13 [8] |
Current treatment for heart failure | 10 [6] |
Current treatment for hypertension | 59 [37] |
Current treatment for arrhythmia | 14 [9] |
Diabetes mellitus | 21 [13] |
Chronic kidney disease | 9 [6] |
Chronic obstructive pulmonary disease | 61 [39] |
†, data missing in 22 patients (14%). ‡, data missing in 20 patients (13%). SD, standard deviation; FEV1, forced expiratory volume in 1 second; pp, percent of predicted; VC, vital capacity; FVC, forced vital capacity; DLCOc, diffusing capacity of the lungs for carbon monoxide, corrected for hemoglobin; TLC, total lung capacity; RV, residual volume.
Within 30 days after surgery, 26 subjects (16.5%) had experienced MPC or death, and there had been three deaths. Registered complications were pneumonia (n=9), pulmonary embolus (n=1), empyema (n=4), delayed extubation (n=5), re-operation (n=12), and respiratory insufficiency (n=3). The causes of death in the three patients were: pneumonia and subsequently respiratory failure, sudden circulatory collapse (no autopsy was performed but clinically massive pulmonary embolus was suspected), and the third patient suffered cardiac herniation with subsequent right heart failure following left sided pneumonectomy. Complications were more common in males (26.7% vs. 7.2% in females, P=0.001). No difference was found in the frequency of complications for patients selected for lobectomy compared to pneumonectomy (16.7% vs. 15.0%, P=0.85). Patients selected for pneumonectomy had higher mean peak VO2 [20±4 (range, 13–28) vs. 17±4 (range, 11–29), P=0.002], with similar mean VE/VCO2 slope values [32±5 (range, 21–47) vs. 34±6 (range, 19–58), P=0.183].
One cut-off generating two risk groups
A threshold of 31 was derived when using the highest Youden value (0.31) for the ROC analysis. The implications of using a threshold of 31 or 35 for identifying postoperative complications are listed in Table 2. The frequency of complications for the low-risk group vs. the high-risk group when using the Youden-derived threshold of 31 was 6% vs. 22% (relative risk 3.66, P=0.006); compared to 12% vs. 26% (relative risk 2.16, P=0.026), when using the traditional threshold of 35. ORs for low and high-risk groups are presented in Table 3.
Table 2
Traditional threshold (cut-off ≥35) |
Highest Youden value (cut-off ≥31) |
High sensitivity threshold (cut-off <30) |
High specificity threshold (cut-off >41) |
|
---|---|---|---|---|
Sensitivity | 0.54 | 0.88 | 0.92 | 0.35 |
Specificity | 0.69 | 0.39 | 0.32 | 0.92 |
PPV | 0.25 | 0.22 | 0.21 | 0.47 |
NPV | 0.88 | 0.95 | 0.95 | 0.88 |
LR+ | 1.74 | 1.44 | 1.35 | 4.38 |
LR− | 0.67 | 0.31 | 0.25 | 0.71 |
VE/VCO2, the increase in minute ventilation in relation to carbon dioxide elimination; PPV, positive predictive value; NPV, negative predictive value; LR+, positive likelihood ratio; LR−, negative likelihood ratio.
Table 3
Thresholds | VE/VCO2 slope groups | Risk groups | N [%] | Unadjusted analysis | Adjusted analysis† | |||||
---|---|---|---|---|---|---|---|---|---|---|
OR | 95% CI | P | OR | 95% CI | P | |||||
Traditional | <35 | Low risk‡ | 103 [65] | |||||||
≥35 | High risk | 55 [35] | 2.59 | 1.10–6.09 | 0.029 | 2.13 | 0.78–5.88 | 0.14 | ||
Derived from highest Youden | <31 | Low risk‡ | 61 [39] | |||||||
≥31 | High risk | 97 [61] | 4.98 | 1.42–17.44 | 0.012 | 4.44 | 1.13–17.24 | 0.033 | ||
Two thresholds§ | <30 | Low risk‡ | 44 [28] | |||||||
30–41 | Intermediate risk | 95 [60] | 3.94 | 0.86–18.04 | 0.078 | 4.00 | 0.79–20.20 | 0.09 | ||
>41 | High risk | 19 [12] | 18.90 | 3.52–101.43 | 0.001 | 19.05 | 2.66–136.53 | 0.003 |
†, analyses adjusted for age, sex, lobectomy/pneumonectomy, surgical technique (open approach or MITS), chronic obstructive pulmonary disease, and percent predicted total lung capacity. ‡, low-risk group is used as the referent. §, thresholds are chosen from the VE/VCO2 slope values closest to generate 90% sensitivity or 90% specificity for detecting major pulmonary complications or death after major lung resection. MITS, minimally invasive thoracic surgery; OR, odds ratio; VE/VCO2, the increase in minute ventilation in relation to carbon dioxide elimination; CI, confidence interval.
Two cut-offs generating three risk groups
The two thresholds derived from the VE/VCO2 slope values closest to a corresponding 90% sensitivity (lower threshold) and 90% specificity (higher threshold) were 30 and 41, respectively. These thresholds generated three risk groups: low risk (VE/VCO2 slope <30 (44 patients, 28%); intermediate risk (VE/VCO2 slope 30–41, 95 patients, 60%) and high risk (VE/VCO2 slope >41, 19 patients, 12%). The frequency of complications differed between the low-, intermediate-, and high-risk groups: 5%, 16%, 47%, P<0.001. The prognostic values for these two thresholds are listed in Table 2 and the corresponding ORs in Table 3. Presentations of AUC values from ROC analyses for different thresholds of the VE/VCO2 slope are presented in Table 4 and in Figure 1.
Table 4
Thresholds | AUC | 95% CI | Standard error | P |
---|---|---|---|---|
Traditional† | 0.61 | 0.49–0.74 | 0.06 | 0.07 |
Derived from highest Youden‡ | 0.64 | 0.54–0.74 | 0.05 | 0.009 |
Two thresholds§ | 0.70 | 0.59–0.81 | 0.06 | 0.0003 |
†, threshold for the VE/VCO2 slope ≥35, ‡, threshold for the VE/VCO2 slope ≥31, §, thresholds for the VE/VCO2 slope <30 and >41. ROC, receiver operating curve; VE/VCO2, the increase in minute ventilation in relation to carbon dioxide elimination; AUC, area under the curve; CI, confidence interval.
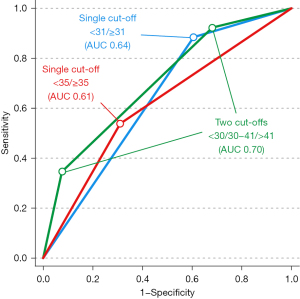
Sensitivity analysis
In a first sensitivity analysis, we only included the 114 patients with intermediate peak VO2 values (i.e., 10–20 mL/kg/min). Using the two threshold method (low-risk group as the referent), the ORs for MPC or death were: VE/VCO2 slope 30–41: OR 2.34, 95% CI: 0.49–11.27 (P=0.29); VE/VCO2 slope >41: OR 11.50, 95% CI: 2.07–63.90 (P=0.005).
In a second sensitivity analysis, we excluded 20 patients undergoing pneumonectomy (thus including 138 lobectomy patients). Using the two threshold method (low-risk group as the referent), the ORs for MPC or death were: VE/VCO2 slope 30–41, OR 4.88, 95% CI: 1.05–22.70 (P=0.044); VE/VCO2 slope >41: OR 18.00, 95% CI: 3.31–97.96 (P=0.001).
Discussion
Key findings
Traditionally, when using one threshold, CPET is known for its strength in identifying patients with a high functional reserve who are at low risk of sustaining a serious complication (10). In this study, we found that using three risk groups based on preoperative CPET identified a high sensitivity threshold (VE/VCO2 slope <30) which generated an NPV of 95% but importantly, also a high specificity threshold (VE/VCO2 slope >41) with a PPV of 47%. This means that 95% of patients classified as low risk did not experience any complications and 47% of patients classified as high risk did suffer a primary outcome complication. Also, using two thresholds compared to one threshold increased the overall model quality based on the AUC analysis.
Strengths and limitations
The strengths of this study include the fairly large and homogenous sample of patients and the stringent classification into two and three risk groups, using a ROC analysis.
This study has some limitations. First, the retrospective approach in this study excluded the possibility of prospectively recording study data. However, we used two Swedish registries of known high quality to define the occurrence of complications, and as only major complications or death were included as outcomes, the risk of under-reporting in the registries should be very low. Second, this was a single-center study with 30 days follow-up time. Therefore, the thresholds generated from this study as well as their long-term implications need to be validated in future cohorts. Third, we were unable to include patients with a very high risk of complications (peak VO2 <10 mL/kg/min or ppFEV <30%), as these patients did not undergo surgery at our center. Therefore, the thresholds proposed in this study are only valid when first excluding this very frail patient group. This is important since patients with severe COPD can present with normal VE/VCO2 slopes due to their ventilatory limitation and/or altered set point for partial pressure of carbon dioxide (PaCO2) (20). Fourthly, although we argue that using two thresholds is advantageous from a clinical perspective, the AUC value from the ROC analysis was moderate (AUC 0.70). This is not surprising and indicates that more factors than only the VE/VCO2-slope are of importance for the risk of post operative complications.
Comparison with similar research
Ventilatory efficiency and the VE/VCO2 slope
Ideally, there is a perfect match between lung perfusion and lung ventilation. When a mismatch occurs (e.g. due to dead space ventilation), gas exchange is impaired, requiring greater ventilation for a given output of CO2. This ‘ventilatory inefficiency’ is reflected in an increase in the VE/VCO2 slope measured during CPET (3). The VE/VCO2 slope has been found to be of value for assessing both the presence and severity of heart or lung disease (21,22), as well as for preoperative risk evaluation (4). The method currently recommended for determining the VE/VCO2 slope evaluates the ventilation in relation to the CO2 output below the ventilatory compensation point (13).
VE/VCO2 slope determination was first used by cardiologists evaluating patients with heart failure (3). Therefore, most studies using CPET for preoperative risk stratification refer to thresholds of VE/VCO2 slope generated from historical data in heart failure patients in the 1990s or early 2000s (8,9), most often using a cut-off of 35 to identify high-risk patients (4,7). Also, as noted by others (23), thresholds suggested from earlier iterations of guiding documents should be reevaluated recurrently since treatment techniques and indications for surgery may change over time.
Therefore, the current study fills an important gap in the literature by reevaluating clinically meaningful thresholds of the VE/VCO2 slope in the specific context of preoperative risk assessment in lung cancer surgery.
Explanations of findings
Traditional analysis using one threshold
As expected, using the traditional VE/VCO2 slope threshold at 35 (derived from heart failure populations as outlined above) did correspond to a significant difference in the frequency of complications between groups. However, a slight improvement in the quality of the overall model was found (AUC 0.61 to 0.64) when using a threshold of 31 (defined by ROC analysis) to identify high- vs. low-risk groups. Both of these cut-offs generated clinically useful values in terms of NPVs, but none reached clinically meaningful PPVs, and this has been described as one of the major limitations in preoperative risk evaluation using CPET (10).
The benefit of two thresholds
Using a single threshold entails a binary approach toward risk assessment, which is problematic in the real, more complex practice of preoperative CPET (24,25). Therefore, some authors have recommended adopting a dynamic range of values as a superior method of distinguishing the various stages of surgical risk (26).
The principle of embracing the “gray zone” in analyzing medical tests was first proposed in the evaluation of clinical biomarkers (27), but has also been used in perioperative medicine when assessing the diagnostic accuracy of pulse pressure variations for the prediction of fluid responsiveness (28). The idea is that one threshold is chosen to exclude the diagnosis with near-certainty (i.e., privilege specificity) while the second threshold is chosen to include the diagnosis with near-certainty (i.e., privilege sensitivity). This approach results in less loss of information compared to choosing a single cut-off, therefore providing an advantage in interpretation over a binary outcome (27).
Recently the “gray zone” has also been acknowledged in preoperative CPET studies where a metanalysis in esophagus cancer patients failed to generate good prognostic values for postoperative outcomes using single cut-off values for CPET measures (25). Instead, a gray zone approach has been used in elective colorectal surgery which suggested two thresholds for the VE/VCO2 slope at >40.1 and <32.7 (26). These thresholds have also been linked to a stepwise increase in mortality in major abdominal surgery (29). These threshold values are similar to the findings in our study, suggesting external validity of our results, especially for the threshold that privileges specificity, i.e., a VE/VCO2 slope of 41 in our material. Thus, a patient presenting with a VE/VCO2 slope above approximately 40 during preoperative CPET should be considered at particularly high risk of complications and may require additional peri- and post-operative care.
The sensitivity analysis showed that the results are also valid for the patient group defined as at moderate risk for postoperative complications or death, patients with a peak VO2 at 10–20 mL/kg/min. This is the patient group that has most often been risk-stratified by the VE/VCO2 slope, but previously only by a single threshold (19). Our second sensitivity analysis showed that the prognostic capacity of the VE/VCO2 slope was increased when only including lobectomies. This is reasonable since pneumonectomy is a more invasive procedure, which may make other risk factors less influential.
Implications and actions needed
After having identified patients at low, intermediate or high risk for pulmonary complications or death, how can the perioperative physician translate these results to clinical decision-making, ultimately decreasing the risk for the individual patient? First, although the frequency of the primary outcome was 47% in the high-risk group identified, this patient group still had a low risk of short term mortality and should not be excluded from surgery only based on this finding. Instead, if a previously unknown pathology is identified, treatment can be initiated to treat the underlying condition. Second, prehabilitation can be initiated which has been shown to reduce postoperative pulmonary and severe complications as well as length of stay in patients undergoing surgery for non-small cell lung cancer (30). However, previous data are discordant regarding if prehabilitation can increase ventilatory efficiency (i.e., lowering the VE/VCO2 slope) in patients awaiting pulmonary resection (31,32). High VE/VCO2 slope can be an indication of manifest severe comorbidity such as pulmonary artery hypertension or right heart failure and these patients should preferably be investigated with echocardiography preoperatively. Third, high-risk patients that proceed to operation should be evaluated with caution to identify complications before severe organ failure occurs, a situation that has been called the “failure of rescue” (33). Interestingly, one study has shown that in patients identified as having an intermediary risk, the risk of complications was dependent on whether they were treated on a high dependency unit or a standard postoperative ward (34). This could in turn speak in favor of having different postoperative care or readiness for complications depending on preoperative risk assessment, where CPET may play an important role.
Conclusions
The risk of major pulmonary complications or death following major lung cancer surgery was better defined using three risk groups based on preoperative VE/VCO2 slope than when using traditional risk stratification into two risk groups. These results could be translated into improved perioperative management, as this could facilitate the identification of patients where the risk of major complications is very high or very low.
Acknowledgments
Funding: This study was funded by unrestricted grants from
Footnote
Reporting Checklist: The authors have completed the STROBE reporting checklist. Available at https://jtd.amegroups.com/article/view/10.21037/jtd-23-1292/rc
Data Sharing Statement: Available at https://jtd.amegroups.com/article/view/10.21037/jtd-23-1292/dss
Peer Review File: Available at https://jtd.amegroups.com/article/view/10.21037/jtd-23-1292/prf
Conflicts of Interest: All authors have completed the ICMJE uniform disclosure form (available at https://jtd.amegroups.com/article/view/10.21037/jtd-23-1292/coif). The authors have no conflicts of interest to declare.
Ethical Statement: The authors are accountable for all aspects of the work in ensuring that questions related to the accuracy or integrity of any part of the work are appropriately investigated and resolved. Ethical permission was granted by the Swedish Ethical Review Authority (Dnr 2020-03375, 2020-05284, 2021-00543). The study was conducted in accordance with the Declaration of Helsinki (as revised in 2013). Informed consent was waived for this retrospective, de-identified analysis.
Open Access Statement: This is an Open Access article distributed in accordance with the Creative Commons Attribution-NonCommercial-NoDerivs 4.0 International License (CC BY-NC-ND 4.0), which permits the non-commercial replication and distribution of the article with the strict proviso that no changes or edits are made and the original work is properly cited (including links to both the formal publication through the relevant DOI and the license). See: https://creativecommons.org/licenses/by-nc-nd/4.0/.
References
- Brunelli A, Kim AW, Berger KI, et al. Physiologic evaluation of the patient with lung cancer being considered for resectional surgery: Diagnosis and management of lung cancer, 3rd ed: American College of Chest Physicians evidence-based clinical practice guidelines. Chest 2013;143:e166S-90S.
- Brunelli A, Charloux A, Bolliger CT, et al. ERS/ESTS clinical guidelines on fitness for radical therapy in lung cancer patients (surgery and chemo-radiotherapy). Eur Respir J 2009;34:17-41. [Crossref] [PubMed]
- Sun XG, Hansen JE, Garatachea N, et al. Ventilatory efficiency during exercise in healthy subjects. Am J Respir Crit Care Med 2002;166:1443-8. [Crossref] [PubMed]
- Brunelli A, Belardinelli R, Pompili C, et al. Minute ventilation-to-carbon dioxide output (VE/VCO2) slope is the strongest predictor of respiratory complications and death after pulmonary resection. Ann Thorac Surg 2012;93:1802-6. [Crossref] [PubMed]
- Miyazaki T, Callister MEJ, Franks K, et al. Minute ventilation-to-carbon dioxide slope is associated with postoperative survival after anatomical lung resection. Lung Cancer 2018;125:218-22. [Crossref] [PubMed]
- Kristenson K, Hylander J, Boros M, et al. Ventilatory efficiency in combination with peak oxygen uptake improves risk stratification in patients undergoing lobectomy. JTCVS Open 2022;11:317-26. [Crossref] [PubMed]
- Shafiek H, Valera JL, Togores B, et al. Risk of postoperative complications in chronic obstructive lung diseases patients considered fit for lung cancer surgery: beyond oxygen consumption. Eur J Cardiothorac Surg 2016;50:772-9. [Crossref] [PubMed]
- Chua TP, Ponikowski P, Harrington D, et al. Clinical correlates and prognostic significance of the ventilatory response to exercise in chronic heart failure. J Am Coll Cardiol 1997;29:1585-90. [Crossref] [PubMed]
- Corrà U, Mezzani A, Bosimini E, et al. Ventilatory response to exercise improves risk stratification in patients with chronic heart failure and intermediate functional capacity. Am Heart J 2002;143:418-26. [Crossref] [PubMed]
- Stubbs DJ, Grimes LA, Ercole A. Performance of cardiopulmonary exercise testing for the prediction of post-operative complications in non cardiopulmonary surgery: A systematic review. PLoS One 2020;15:e0226480. [Crossref] [PubMed]
- von Elm E, Altman DG, Egger M, et al. The Strengthening the Reporting of Observational Studies in Epidemiology (STROBE) statement: guidelines for reporting observational studies. Lancet 2007;370:1453-7. [Crossref] [PubMed]
- Borg GA. Psychophysical bases of perceived exertion. Med Sci Sports Exerc 1982;14:377-81.
- Mezzani A. Cardiopulmonary Exercise Testing: Basics of Methodology and Measurements. Ann Am Thorac Soc 2017;14:S3-S11. [Crossref] [PubMed]
- Hedenström H, Malmberg P, Agarwal K. Reference values for lung function tests in females. Regression equations with smoking variables. Bull Eur Physiopathol Respir 1985;21:551-7.
- Hedenström H, Malmberg P, Fridriksson HV. Reference values for lung function tests in men: regression equations with smoking variables. Ups J Med Sci 1986;91:299-310. [Crossref] [PubMed]
- Fernandez FG, Falcoz PE, Kozower BD, et al. The Society of Thoracic Surgeons and the European Society of Thoracic Surgeons general thoracic surgery databases: joint standardization of variable definitions and terminology. Ann Thorac Surg 2015;99:368-76. [Crossref] [PubMed]
- The Swedish National Quality Register for General Thoracic Surgery [Internet]. 2023 [cited 2023-04-14]. Available online: http://www.ucr.uu.se/thor
- Swedish National Board of Health and Welfare. Quality and Contents of the Swedish Hospital Discharge Register. The Centre for Epidemiology. 2008. Available online: https://www.socialstyrelsen.se/en/statistics-and-data/registers/national-patient-register/
- Salati M, Brunelli A. Risk Stratification in Lung Resection. Curr Surg Rep 2016;4:37. [Crossref] [PubMed]
- Neder JA, Berton DC, Arbex FF, et al. Physiological and clinical relevance of exercise ventilatory efficiency in COPD. Eur Respir J 2017;49:1602036. [Crossref] [PubMed]
- Wasserman K, Zhang YY, Riley MS. Ventilation during exercise in chronic heart failure. Basic Res Cardiol 1996;91:1-11. [Crossref] [PubMed]
- Medinger AE, Khouri S, Rohatgi PK. Sarcoidosis: the value of exercise testing. Chest 2001;120:93-101. [Crossref] [PubMed]
- Levett DZH, Jack S, Swart M, et al. Perioperative cardiopulmonary exercise testing (CPET): consensus clinical guidelines on indications, organization, conduct, and physiological interpretation. Br J Anaesth 2018;120:484-500. [Crossref] [PubMed]
- Older P. Anaerobic threshold, is it a magic number to determine fitness for surgery? Perioper Med (Lond) 2013;2:2. [Crossref] [PubMed]
- Sivakumar J, Forshaw MJ, Lam S, et al. Identifying the limitations of cardiopulmonary exercise testing prior to esophagectomy using a pooled analysis of patient-level data. Dis Esophagus 2022;35:doac005. [Crossref] [PubMed]
- Rose GA, Davies RG, Davison GW, et al. The cardiopulmonary exercise test grey zone; optimising fitness stratification by application of critical difference. Br J Anaesth 2018;120:1187-94. [Crossref] [PubMed]
- Ray P, Le Manach Y, Riou B, et al. Statistical evaluation of a biomarker. Anesthesiology 2010;112:1023-40. [Crossref] [PubMed]
- Cannesson M, Le Manach Y, Hofer CK, et al. Assessing the diagnostic accuracy of pulse pressure variations for the prediction of fluid responsiveness: a "gray zone" approach. Anesthesiology 2011;115:231-41. [Crossref] [PubMed]
- Wilson RJT. Shades of grey: embracing uncertainty in the exercise room. Br J Anaesth 2018;120:1145-6. [Crossref] [PubMed]
- Voorn MJJ, Franssen RFW, Hoogeboom TJ, et al. Evidence base for exercise prehabilitation suggests favourable outcomes for patients undergoing surgery for non-small cell lung cancer despite being of low therapeutic quality: a systematic review and meta-analysis. Eur J Surg Oncol 2023;49:879-94. [Crossref] [PubMed]
- Perrotta F, Cennamo A, Cerqua FS, et al. Effects of a high-intensity pulmonary rehabilitation program on the minute ventilation/carbon dioxide output slope during exercise in a cohort of patients with COPD undergoing lung resection for non-small cell lung cancer. J Bras Pneumol 2019;45:e20180132. [Crossref] [PubMed]
- Divisi D, Di Francesco C, Di Leonardo G, et al. Preoperative pulmonary rehabilitation in patients with lung cancer and chronic obstructive pulmonary disease. Eur J Cardiothorac Surg 2013;43:293-6. [Crossref] [PubMed]
- Ghaferi AA, Birkmeyer JD, Dimick JB. Variation in hospital mortality associated with inpatient surgery. N Engl J Med 2009;361:1368-75. [Crossref] [PubMed]
- Swart M, Carlisle JB, Goddard J. Using predicted 30 day mortality to plan postoperative colorectal surgery care: a cohort study. Br J Anaesth 2017;118:100-4. [Crossref] [PubMed]