Advancements in preoperative imaging of pectus excavatum: a comprehensive review
Introduction
Congenital pectus deformities are a set of structural anterior chest wall abnormalities. The most common pectus deformity is pectus excavatum, which is characterized by a sunken chest wall and represents 90% of all cases (1). The disease has been associated with cardiopulmonary impairment and related symptoms such as exercise intolerance, and psychological complaints due to body image disturbances (2-4).
A sunken chest wall was first described by Bauhinus in 1594 and more detailed reports followed in the nineteenth century (1,5). These reports included anatomical descriptions of the deformation, examination of symptoms, and assessments on pathogenesis and familial predisposition (5). The first attempt to correct a pectus deformity was performed by Meyer in 1911 through rib removal surgery (6,7). Ever since, numerous techniques regarding treatment (spanning from less invasive to more invasive methods) as well as (preoperative) evaluation of pectus deformities have been recommended.
The main purposes of preoperative evaluation include diagnosis, severity classification, functional assessment, and surgical planning of chest wall deformities. Over the years, preoperative evaluation of pectus deformities has evolved from simple clinical inspection and descriptive analyses with caliper measurements of the chest to more advanced quantitative measurements and imaged documentation.
Since the discovery of X-rays in 1895 and development of the first computed tomography (CT) scanner in 1971, internal structures of the human body have been imaged extensively. These imaging methods have also led to significant evolvement in clinical evaluation of pectus excavatum, accelerating the development of numerous indices and methods to assess chest wall deformities. As such, Welch described in 1958 an index derived from a lateral chest radiograph to distinguish mild, moderate, and severe degrees of pectus excavatum from normal chest wall profiles (8,9). In 1987, the Haller index was established, describing the ratio between the maximum transverse diameter and minimum anterior-posterior distance of the chest (10).
Nowadays, several methods for preoperative assessment are routinely applied in clinical practice, each with associated advantages and disadvantages and specific applications. While preoperative imaging is mainly used to visualize the morphology of the deformation, it can also be used to quantify its effects on intrathoracic organs. Since imaging techniques are continuously advancing, new insights and opportunities are created in the clinical assessment of pectus deformities. This narrative review aims to provide current insights in conventional methods and innovative developing techniques for preoperative imaging of pectus excavatum; the most common and widely studied chest wall deformity.
Conventional preoperative imaging methods
Strategies for preoperative evaluation of pectus deformities may vary between medical centers, e.g., due to national regulations, individual preferences, a paucity of evidence and a lack of guidelines. The current paper focusses on the most commonly applied preoperative imaging methods, including thoracic CT, chest radiography, cardiac magnetic resonance imaging (MRI), echocardiography and medical photography (Table 1).
Table 1
Technique | Imaging modality | Applications | Advantages | Limitations | Current developments |
---|---|---|---|---|---|
Conventional | Medical photography | Identification and documentation of chest wall deformations | Radiation free | No information on internal chest deformations | N/A |
Fast | |||||
Chest X-ray | Calculation of pectus indices | Reduced costs and radiation exposure compared to computed tomography | Unsuitable for (disproportionally) asymmetric chest deformations | N/A | |
Computed tomography | Calculation of pectus indices Identifying cardiac compression/displacement, chest asymmetry and sternal torsion |
3D reconstruction of thoracic wall | Ionizing radiation | Automatic quantification of pectus indices Radiation reduction Surgical planning |
|
Magnetic resonance imaging | Calculation of pectus indices Identifying cardiac compression/displacement Evaluate cardiac function |
Radiation free Real-time imaging |
Availability of resources Expenses |
Automatic quantification of pectus indices Exercise magnetic resonance imaging |
|
Echocardiography | Cardiac evaluation (particularly structural changes and anomalies) | Radiation free | Limited accuracy in evaluation of cardiac function | N/A | |
No (current) applications in surgical planning | |||||
Emerging | 3D imaging | Identification and documentation of chest wall deformations | Radiation free | Limited accuracy (e.g., in patients with breasts or excess body fat) | Automatic quantification of pectus indices |
Calculation of externally derived pectus indices (which can be correlated to internal equivalents) | Fast | Surgical planning |
N/A, not applicable.
CT
CT has been a frequently applied modality for assessment of pectus excavatum (Figure 1). The calculation of pectus indices (e.g., the Haller index and correction index) and identifying cardiac compression and displacement, chest asymmetry and sternal torsion are the main applications of CT. Current developments in preoperative evaluation through CT imaging focus on automatic quantification of pectus indices, minimizing radiation dose and surgical planning (11-29).
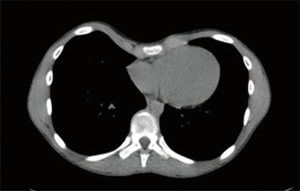
Over the years, various indices to quantify and characterize chest wall deformities have been developed and are in general manually extracted from CT images (10,30-32). To diminish time-consuming analyses and bypass intra- and interobserver variability of manual measurements, some studies focus on (semi-)automatic evaluation of pectus deformities. Between 2008 and 2010, Kim et al. proposed four indices (the eccentricity index, flatness index, circularity index, and rotation index) that quantify the depression and asymmetry of pectus excavatum and designed a fully automatic pipeline to calculate these indices using several image processing techniques, such as a segmentation algorithm and a curve fitting (active contour) model (11,12). A few years later, they extended their method and proposed two other indices (the depression ratio and asymmetry ratio) to provide a linear output for the severity of symmetric, asymmetric and complex chest wall deformities (13).
In contrast to an index-based evaluation of pectus excavatum severity, Lai et al. [2020] proposed a non-index-based approach, aiming to represent the full chest shape and exploit the volumetric information in chest CT images (14). They developed an automatic classification model based on deep learning that differentiates each CT slice as normal, mild, or severe pectus excavatum. Subsequently, a majority rule-based voting strategy is applied across the entire CT volume to draw a final diagnostic conclusion on the pectus severity.
Although these algorithms have been proposed in the past 15 years to automatically extract novel indices that evaluate pectus severity from CT images, none aimed to develop automated methods to calculate conventional and clinically used pectus indices such as the Haller index and correction index. Only recently, in 2022, Silva et al. applied a UNet++ architecture that was evaluated on a database of 269 CT scans to automatically calculate the Haller index, correction index and asymmetry index (15).
The greatest concern regarding the use of a CT scan for evaluating pectus deformities, particularly in pediatric and adolescent patients, involves the relatively high dose of ionizing radiation. In an effort to reduce the CT-associated radiation dose for pectus excavatum assessment, a so-called very low-dose protocol has been suggested, consisting of five to seven slices centered on the area of maximal sternal depression (16). This method reduces the radiation dose from 1–2 mSv in full chest CT to approximately 0.35 mSv, while still allowing the calculation of the Haller index and displaying local anatomy of interest.
Two other recent studies focused on the development of a CT imaging protocol to minimize radiation exposure. Gosztyla et al. [2022] proposed a mini-thoracic CT for the calculation of the Haller index (17). This mini-CT involves the placement of a radio-opaque marker at the point of most severe depression, after which a column of 3 cm above and 3 cm below the marker is scanned using 5 mm slices. Compared to a general low dose chest-CT of 10 mm slices from the sternal inlet to the costal margin, the mini-CT reduced the effective dose (mSv) by 63% (0.88 versus 0.32 mSv). The effective dose can be brought down even further to less than 0.15 mSv by implementing an ultralow-dose CT protocol (18,19). Gallo-Bernal et al. [2022] compared an ultralow-dose CT protocol (70–80 kV; fixed tube current of 50 mAs; slice thickness 2.5 mm) to a standard-dose CT protocol (100–120 kV; variable tube current, smart mA; slice thickness 2.5 mm) by evaluating the effect on image quality as well as the capability to detect non-osseous thoracic pathology (18). A scanning range from the thoracic inlet to the level of the adrenal glands was used and scans were reconstructed using adaptive statistical iterative reconstruction, which supports preservation of diagnostic image quality in low-dose CT acquisition. The signal-to-noise and contrast-to-noise ratios were significantly higher in standard CT images and 3 out of 104 ultralow-dose scans were considered insufficient to diagnose thoracic pathology beyond pectus excavatum.
Besides using CT for pectus evaluation, CT is considered a well-suited imaging modality for surgical planning of pectus excavatum repair. Surgeons may devise surgical strategies based upon CT-derived three-dimensional (3D) reconstruction of the thoracic wall (20). Other emerging techniques to aid in the decision process are 3D printing (additive manufacturing) of thoracic models and bar templates, or virtual simulations of the Nuss procedure (21-29). Preoperative planning using CT scans allows to create personalized pectus bars preoperatively for more effective corrections of the deformity and to eliminate intraoperative revisions of the bar shape. However, whether these technologies improve surgical accuracy and reduce surgical time and costs compared to conventional techniques remains to be elucidated.
Chest radiography
Chest radiography is an alternative imaging method to visualize and assess the degree of chest wall deformity (Figure 2). Aside from a depression of the sternum, characteristic findings of pectus excavatum on chest radiographs include a leftward displacement of the cardiac silhouette, obscured right cardiac border, narrowing of the mediastinum, and slanted anterior ribs (33). Although chest radiography is unsuitable to evaluate chest asymmetry or sternal torsion, various pectus indices to assess the deformity can be determined from plain radiographs (e.g., Welch index, vertebral index, body manubrium xyphoid index, Haller index, correction index) (8,10,30,32,34,35).
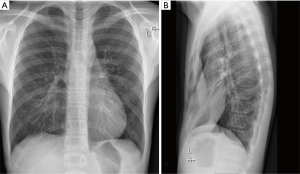
Both the Haller index and the correction index are originally CT-derived indices, but it has been shown that these indices can be measured reliably and accurately based on chest radiographic images (36-39). By replacing a full chest CT scan with a two-way chest radiograph, the estimated reduction in radiation exposure is in the order of 100-fold (1.1–4.1 versus 0.0198 mSv), while associated costs are decreased by approximately 90% (37,38). To further refine the measurement accuracy of the Haller index and correction index on radiographic images, potential confounders were investigated by McHugh et al. [2016]. The accuracy of both the Haller index and correction index measurements were not influenced by image quality, scoliosis and an eccentric type of pectus asymmetry. Nevertheless, caution is advocated when using chest radiography alone in cases of an unbalanced type of asymmetry (i.e., a disproportionate depression on one side of the chest compared to the contralateral side, with a difference in angles of >10 degrees), since this method was found to decrease measurement accuracy of the correction index when compared to CT measurements (40).
In addition to preoperative application of two-view chest radiography, single anterior-posterior radiographs of the chest are often obtained directly postoperatively to assess the position of implanted pectus bars and to detect complications such as a pneumothorax.
MRI
Traditionally, preoperative imaging in patients with pectus excavatum has been centered around radiographic imaging. However, over the past two decades, MRI has emerged as an alternative imaging method in this context (Figure 3). Similar to CT, an MRI allows for the (semi-automatic) calculation of traditional pectus indices (41), with the added advantage of imaging without exposure to ionizing radiation and the possibility to evaluate dynamic cardiac function (42-49).
Conventional methods to evaluate myocardial function on MRI are through the assessment of wall motion abnormalities or ejection fraction. However, imaging of ventricular myocardial strain (i.e., fractional deformation of the myocardial wall throughout the cardiac cycle) has been shown to detect myocardial dysfunction at an earlier stage compared to conventional measures (50-52).
In case of pectus excavatum, assessment of cardiac function is mainly focused on the right ventricle since the associated sternal depression usually deforms the right ventricular wall (53). To improve cardiac MRI assessment in patients with pectus excavatum, Rodriguez-Granillo et al. [2022] evaluated systolic function of both the right and left ventricles using longitudinal cardiac strain and conventional ejection fraction analyses (50). The study demonstrated that right ventricular systolic dysfunction can be identified using conventional analyses, while subtle left ventricular systolic dysfunction could only be detected using longitudinal strain analysis and was overlooked by conventional assessment (50). Though, it must be remembered that these results are based on analyses of cardiac function of patients at rest and in supine position. Exercise cardiac MRI is a developing technique which may offer a more comprehensive assessment of cardiac function during exercise (54). Still, this technique requires further advancements and its clinical utility in the preoperative assessment of pectus excavatum has yet to be evaluated.
Evaluating chest wall deformities is generally based on static axial cross-sectional images of the chest because standard imaging techniques require breath-holding procedures. Although it has been demonstrated that thoracic indices, such as the Haller index and correction index, vary during breathing cycle, the phase at which the indices should be determined has not been standardized. The added value of MRI compared to other imaging methods is the possibility for real-time imaging. In a recent study (55), dynamics of the chest wall in 56 patients with pectus excavatum have been observed using real-time MRI. The results showed that between maximum inspiration and maximum expiration, the Haller index passed through the clinical threshold of 3.25 in only 4 subjects (7%), while the correction index passed its threshold of 2% in 15 subjects (27%). Although measurement variance was not addressed in this study, dynamic real-time MRI in the assessment of pectus excavatum may provide clear visualization and a better understanding of the compression by the sternum on the heart and lungs and thus helps in clinical decision-making. In case of conventional static imaging of the chest wall, the authors recommend calculating thoracic indices in the end-expiratory phase of quiet breathing, since the respective indices are not significantly dependent on the degree of expiration and the intraoperative situation is resembled more closely by the end-expiratory breathing phase (55).
Echocardiography
Echocardiographic imaging (see Figure 4) may be useful for cardiac evaluation, as it can reveal structural and functional changes of the right ventricle (e.g., compression of the right atrium and ventricle; systolic and diastolic disfunction) and heart valves (e.g., tricuspid- and mitral valve prolapse) as well as the presence of (congenital) anomalies (e.g., septal defects, aortic root dilation and pericardial effusion) (56,57). A dilated aortic root and mitral valve prolapse can for example indicate Marfan’s syndrome. Transthoracic imaging is often challenging in patients with pectus excavatum due to the deformation of the thoracic and cardiac geometry. In some cases a subcostal view may provide adequate images, but transesophageal imaging can largely overcome the limited view during imaging (57,58). However, this technique is practically more inconvenient and often requires sedation of the patient.
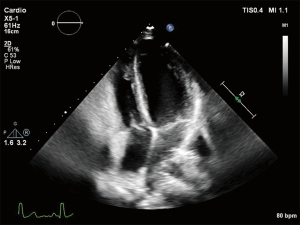
Although echocardiography is performed to identify potential cardiac abnormalities, echocardiographic approaches are of limited accuracy in evaluating cardiac function in patients with pectus excavatum. Qualitative assessment (e.g., tricuspid annular plane systolic excursion) is based on geometrical assumptions which not necessarily hold true in this anatomically distinct population (59). These limitations may explain why there is only limited data on the application of echography in the preoperative assessment of pectus excavatum. Nevertheless, a study from 2020 based on a relatively large cohort (269 patients) analyzed findings from stress echocardiography and explored the relationship with the severity of chest wall malformation based on the Haller index and correction index. Although the mechanisms involved are not fully elucidated, right ventricular diastolic as well as systolic function appeared to worsen during exercise and both were demonstrated to be associated with the Haller index and correction index (60).
Medical photography
Medical photography is a relatively simple, inexpensive, and non-invasive method to image and document anatomical malformations of the chest (Figure 5). Prior to surgical correction of pectus excavatum, it is useful to identify the presence of asymmetry, costal flaring and sternal torsion and the extent of depression (61). Medical photography of pectus excavatum is often combined with a caliper measurement to provide a quantitative measure of the excavation depth (62,63). A reliable protocol for photographic documentation of pectus excavatum consists of three types of recordings: a series of five photographs from different angles (left lateral, left oblique, anterior, right oblique, right lateral) with additional lighting to accentuate the shape of the deformity, a photograph of a caliper measuring the depth of the excavation, and a 3D grid-projection photograph (i.e., raster stereography) (62). By comparing preoperative and postoperative photographs, the result of the surgical correction can be properly assessed and monitored over time.
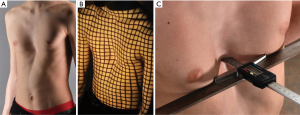
Emerging preoperative imaging: 3D optical surface imaging
An emerging imaging technique to preoperatively evaluate pectus deformities is 3D optical surface imaging, which maps the external contour (i.e., skin surface) of the thoracic wall (Figure 6). This optical scanning technique offers the acquisition of images as fast as within a few seconds, without ionizing radiation. Such scanning devices can be based on several different technologies to capture and reconstruct image data, each having distinct advantages and limitations with a broad range of costs. Most scanning devices used for chest wall imaging are based on structured light projection technology in which the distortion of a pattern of light due to the thoracic geometry allows for the measurement of protrusions and depression in the examined surface. A 3D surface scan can be represented by point clouds or a polygonal mesh that can be analyzed manually or automatically to produce quantitative measurements. Several studies have investigated the application of optical scanning in the preoperative evaluation of pectus deformities and demonstrated that a modified pectus index based on the skin surface (i.e., an external-derived Haller or correction index) correlates with the conventional Haller and correction index (64-72). In addition, cut-off values for externally derived indices to help determine surgical candidacy have been established. The optimal cut-off value for the external Haller index was calculated at ≥1.83 (corresponding to the conventional Haller index ≥3.25) and the external correction index at ≥15.2% (corresponding to the conventional correction index ≥28.0%) (73). Along with the calculation of equivalents to conventional pectus indices, optical imaging may be used to quantify other morphological features of pectus excavatum (74) and can predict the presence of cardiac compression without the use of CT imaging (75). Combined with automatic algorithms and predictive models, 3D surface scans may aid in preoperative decision-making and visualization of expected aesthetic outcomes after surgery (76).
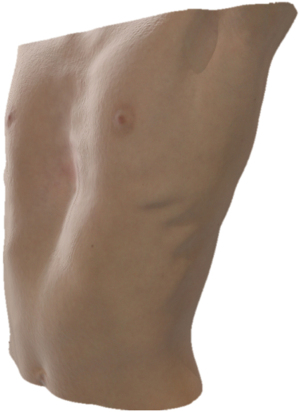
Nevertheless, these developments are still in their infancy and require further evaluation and validation. Prior to widespread use of 3D optical imaging, additional issues should be addressed regarding accuracy of measurements in patients with breasts or excess body fat, standardization of imaging systems and analyses, cost and availability of 3D imaging systems in medical centers.
Conclusions
Preoperative imaging of pectus excavatum comprises a variety of imaging modalities which have been evolved extensively over the years. Current advancements in preoperative imaging focus on radiation reduction, automatic quantification of pectus indices, and surgical planning. MRI offers valuable advantages, as it permits the (semi-automatic) calculation of traditional pectus indices, as well as the assessment of cardiac function within a single, radiation-free examination. Furthermore, the capacity for real-time imaging can provide insight into the dynamic nature of chest wall deformities. Chest radiography and echocardiography are alternative procedures, but future technological developments are mainly focused on other imaging techniques. The latest emerging imaging technique is three-dimensional optical surface imaging, which may be applied as a non-invasive method to calculate equivalents of conventional pectus indices and other features of pectus excavatum. As a currently developing technology, 3D imaging holds promising potential for future advancements in the field.
Acknowledgments
Funding: None.
Footnote
Provenance and Peer Review: This article was commissioned by the editorial office, Journal of Thoracic Disease, for the series “Minimally Invasive Treatment of Pectus Deformities”. The article has undergone external peer review.
Peer Review File: Available at https://jtd.amegroups.com/article/view/10.21037/jtd-23-662/prf
Conflicts of Interest: All authors have completed the ICMJE uniform disclosure form (available at https://jtd.amegroups.com/article/view/10.21037/jtd-23-662/coif). The series “Minimally Invasive Treatment of Pectus Deformities” was commissioned by the editorial office without any funding or sponsorship. E.R.d.L. and J.H.T.D. served as unpaid Guest Editors of the series. The authors have no other conflicts of interest to declare.
Ethical Statement: The authors are accountable for all aspects of the work in ensuring that questions related to the accuracy or integrity of any part of the work are appropriately investigated and resolved. All clinical procedures described in this study were performed in accordance with the ethical standards of the institutional and/or national research committee(s) and with the Helsinki Declaration (as revised in 2013). Written informed consent was obtained from the patients for the publication of this article and accompanying images.
Open Access Statement: This is an Open Access article distributed in accordance with the Creative Commons Attribution-NonCommercial-NoDerivs 4.0 International License (CC BY-NC-ND 4.0), which permits the non-commercial replication and distribution of the article with the strict proviso that no changes or edits are made and the original work is properly cited (including links to both the formal publication through the relevant DOI and the license). See: https://creativecommons.org/licenses/by-nc-nd/4.0/.
References
- Brochhausen C, Turial S, Müller FK, et al. Pectus excavatum: history, hypotheses and treatment options. Interact Cardiovasc Thorac Surg 2012;14:801-6. [Crossref] [PubMed]
- Maagaard M, Heiberg J. Improved cardiac function and exercise capacity following correction of pectus excavatum: a review of current literature. Ann Cardiothorac Surg 2016;5:485-92. [Crossref] [PubMed]
- Kolvekar SK, Panagiotopoulos N. Pectus Excavatum. In: Kolvekar S, Pilegaard H, editors. Chest Wall Deformities and Corrective Procedures. Springer Cham 2016. p. 17-20.
- Steinmann C, Krille S, Mueller A, et al. Pectus excavatum and pectus carinatum patients suffer from lower quality of life and impaired body image: a control group comparison of psychological characteristics prior to surgical correction. Eur J Cardiothorac Surg 2011;40:1138-45. [Crossref] [PubMed]
- Ochsner A, DeBakey M. Chōnē-chondrosternon: report of a case and review of the literature. J Thorac Surg 1939;8:469-511.
- Sanger PW, Robicsek F, Daugherty HK. The repair of recurrent pectus excavatum. J Thorac Cardiovasc Surg 1968;56:141-3.
- Meyer L. Zur chirurgischen Behandlung der angeborenen Trichterburst. Verh Berliner Med 1911;42:364-73.
- Welch KJ. Satisfactory surgical correction of pectus excavatum deformity in childhood: a limited opportunity. J Thorac Surg 1958;36:697-713.
- Shamberger RC, Welch KJ. Surgical repair of pectus excavatum. J Pediatr Surg 1988;23:615-22. [Crossref] [PubMed]
- Haller JA Jr, Kramer SS, Lietman SA. Use of CT scans in selection of patients for pectus excavatum surgery: a preliminary report. J Pediatr Surg 1987;22:904-6. [Crossref] [PubMed]
- Kim HC, Park HJ, Ham SY, et al. Development of automatized new indices for radiological assessment of chest-wall deformity and its quantitative evaluation. Med Biol Eng Comput 2008;46:815-23. [Crossref] [PubMed]
- Kim HC, Park HJ, Nam KW, et al. Fully automatic initialization method for quantitative assessment of chest-wall deformity in funnel chest patients. Med Biol Eng Comput 2010;48:589-95. [Crossref] [PubMed]
- Kim HC, Choi H, Jin SO, et al. New computerized indices for quantitative evaluation of depression and asymmetry in patients with chest wall deformities. Artif Organs 2013;37:712-8. [Crossref] [PubMed]
- Lai L, Cai S, Huang L, et al. Computer-aided diagnosis of pectus excavatum using CT images and deep learning methods. Sci Rep 2020;10:20294. [Crossref] [PubMed]
- Silva B, Pessanha I, Correia-Pinto J, et al. Automatic Assessment of Pectus Excavatum Severity From CT Images Using Deep Learning. IEEE J Biomed Health Inform 2022;26:324-33. [Crossref] [PubMed]
- Rattan AS, Laor T, Ryckman FC, et al. Pectus excavatum imaging: enough but not too much. Pediatr Radiol 2010;40:168-72. [Crossref] [PubMed]
- Gosztyla CE, Petrosyan M, Kane T, et al. Mini thoracic CT adequately determines Haller index and decreases radiation exposure in children with pectus excavatum. J Pediatr Surg 2022;57:1076-8. [Crossref] [PubMed]
- Gallo-Bernal S, Kim J, Savage C, et al. Detection of Incidental Nonosseous Thoracic Pathology on State-of-the-Art Ultralow-Dose Protocol Computed Tomography in Pediatric Patients With Pectus Excavatum. J Comput Assist Tomogr 2022;46:492-8. [Crossref] [PubMed]
- Messerli-Odermatt O, Serrallach B, Gubser M, et al. Chest X-ray Dose Equivalent Low-dose CT with Tin Filtration: Potential Role for the Assessment of Pectus Excavatum. Acad Radiol 2020;27:644-50. [Crossref] [PubMed]
- Calloway EH, Chhotani AN, Lee YZ, et al. Three-dimensional computed tomography for evaluation and management of children with complex chest wall anomalies: useful information or just pretty pictures? J Pediatr Surg 2011;46:640-7. [Crossref] [PubMed]
- Leonardi B, Carlucci A, Noro A, et al. Three-Dimensional Printed Models for Preoperative Planning and Surgical Treatment of Chest Wall Disease: A Systematic Review. Technologies 2021;9:97.
- Wang L, Guo T, Zhang H, et al. Three-dimensional printing flexible models: a novel technique for Nuss procedure planning of pectus excavatum repair. Ann Transl Med 2020;8:110. [Crossref] [PubMed]
- Huang YJ, Lin KH, Chen YY, et al. Feasibility and Clinical Effectiveness of Three-Dimensional Printed Model-Assisted Nuss Procedure. Ann Thorac Surg 2019;107:1089-96. [Crossref] [PubMed]
- Ho CWG, Tan BK, Ong BH, et al. Customized Two-Dimensional Computed Tomography-Guided Preoperative Pectus Bar Shaping. Ann Plast Surg 2021;87:676-80. [Crossref] [PubMed]
- Matsuo N, Matsumoto K, Taura Y, et al. Initial experience with a 3D printed model for preoperative simulation of the Nuss procedure for pectus excavatum. J Thorac Dis 2018;10:E120-4. [Crossref] [PubMed]
- Bellia-Munzon G, Martinez J, Toselli L, et al. From bench to bedside: 3D reconstruction and printing as a valuable tool for the chest wall surgeon. J Pediatr Surg 2020;55:2703-9. [Crossref] [PubMed]
- Tse KM, Tan LB, Lee SJ, et al. Feasibility of using computer simulation to predict the postoperative outcome of the minimally invasive Nuss procedure: Simulation prediction vs. postoperative clinical observation. J Plast Reconstr Aesthet Surg 2018;71:1496-506. [Crossref] [PubMed]
- Rechowicz KJ, McKenzie FD. Development and validation methodology of the Nuss procedure surgical planner. Simulation 2013;89:1474-88.
- Obeid MF, Kelly R, McKenzie FD. Development and Validation of a Hybrid Nuss Procedure Surgical Simulator and Trainer. IEEE Trans Biomed Eng 2021;68:2520-8. [Crossref] [PubMed]
- St Peter SD, Juang D, Garey CL, et al. A novel measure for pectus excavatum: the correction index. J Pediatr Surg 2011;46:2270-3. [Crossref] [PubMed]
- Bellía-Munzón G, Sanjurjo D, Toselli L, et al. Novel index to estimate the cephalocaudal extent of the excavation in pectus excavatum: The Titanic index. J Pediatr Surg 2023;58:605-7. [Crossref] [PubMed]
- Martinez-Ferro M. Indexes for pectus deformities. In: Kolvekar S, Pilegaard H, editors. Chest Wall Deformities and Corrective Procedures. Springer Cham 2016. p. 35-60.
- Sarwar ZU, DeFlorio R, O'Connor SC. Pectus excavatum: current imaging techniques and opportunities for dose reduction. Semin Ultrasound CT MR 2014;35:374-81. [Crossref] [PubMed]
- Haje SA, Harcke HT, Bowen JR. Growth disturbance of the sternum and pectus deformities: imaging studies and clinical correlation. Pediatr Radiol 1999;29:334-41. [Crossref] [PubMed]
- Derveaux L, Clarysse I, Ivanoff I, et al. Preoperative and postoperative abnormalities in chest x-ray indices and in lung function in pectus deformities. Chest 1989;95:850-6. [Crossref] [PubMed]
- Mueller C, Saint-Vil D, Bouchard S. Chest x-ray as a primary modality for preoperative imaging of pectus excavatum. J Pediatr Surg 2008;43:71-3. [Crossref] [PubMed]
- Khanna G, Jaju A, Don S, et al. Comparison of Haller index values calculated with chest radiographs versus CT for pectus excavatum evaluation. Pediatr Radiol 2010;40:1763-7. [Crossref] [PubMed]
- Poston PM, Patel SS, Rajput M, et al. Defining the role of chest radiography in determining candidacy for pectus excavatum repair. Innovations (Phila) 2014;9:117-21; discussion 121. [Crossref] [PubMed]
- Poston PM, McHugh MA, Rossi NO, et al. The case for using the correction index obtained from chest radiography for evaluation of pectus excavatum. J Pediatr Surg 2015;50:1940-4. [Crossref] [PubMed]
- McHugh MA, Poston PM, Rossi NO, et al. Assessment of potential confounders when imaging pectus excavatum with chest radiography alone. J Pediatr Surg 2016;51:1485-9. [Crossref] [PubMed]
- Trò R, Martini S, Stagnaro N, et al. A new tool for assessing Pectus Excavatum by a semi-automatic image processing pipeline calculating the classical severity indexes and a new marker: the Volumetric Correction Index. BMC Med Imaging 2022;22:30. [Crossref] [PubMed]
- Lollert A, Funk J, Tietze N, et al. Morphologic assessment of thoracic deformities for the preoperative evaluation of pectus excavatum by magnetic resonance imaging. Eur Radiol 2015;25:785-91. [Crossref] [PubMed]
- Birkemeier KL, Podberesky DJ, Salisbury S, et al. Limited, fast magnetic resonance imaging as an alternative for preoperative evaluation of pectus excavatum: a feasibility study. J Thorac Imaging 2012;27:393-7. [Crossref] [PubMed]
- Lai K, Colen TM, Dicken BJ. Integrating cardiac MRI into pre-operative planning for patients with pectus excavatum and right ventricular dysfunction. J Pediatr Surg Case Rep 2019;42:63-5.
- Viña NA, Carrascosa P, Mogensen VC, et al. Evaluation of pectus excavatum indexes during standard cardiac magnetic resonance: Potential for single preoperative tool. Clin Imaging 2019;53:138-42. [Crossref] [PubMed]
- Lo Piccolo R, Bongini U, Basile M, et al. Chest fast MRI: an imaging alternative on pre-operative evaluation of Pectus Excavatum. J Pediatr Surg 2012;47:485-9. [Crossref] [PubMed]
- Stagnaro N, Trocchio G, Torre M, et al. Cardiovascular MRI assessment of pectus excavatum in pediatric patients and postoperative simulation using vacuum bell. J Pediatr Surg 2021;56:1600-5. [Crossref] [PubMed]
- Humphries CM, Anderson JL, Flores JH, et al. Cardiac magnetic resonance imaging for perioperative evaluation of sternal eversion for pectus excavatum. Eur J Cardiothorac Surg 2013;43:1110-3. [Crossref] [PubMed]
- Dore M, Triana Junco P, Bret M, et al. Advantages of Cardiac Magnetic Resonance Imaging for Severe Pectus Excavatum Assessment in Children. Eur J Pediatr Surg 2018;28:34-8. [Crossref] [PubMed]
- Rodriguez-Granillo GA, Toselli L, Farina J, et al. Usefulness of strain cardiac magnetic resonance for the exposure of mild left ventricular systolic abnormalities in pectus excavatum. J Pediatr Surg 2022;57:319-24. [Crossref] [PubMed]
- Scatteia A, Baritussio A, Bucciarelli-Ducci C. Strain imaging using cardiac magnetic resonance. Heart Fail Rev 2017;22:465-76. [Crossref] [PubMed]
- Valente F, Gutierrez L, Rodríguez-Eyras L, et al. Cardiac magnetic resonance longitudinal strain analysis in acute ST-segment elevation myocardial infarction: A comparison with speckle-tracking echocardiography. Int J Cardiol Heart Vasc 2020;29:100560. [Crossref] [PubMed]
- Oezcan S, Attenhofer Jost CH, Pfyffer M, et al. Pectus excavatum: echocardiography and cardiac MRI reveal frequent pericardial effusion and right-sided heart anomalies. Eur Heart J Cardiovasc Imaging 2012;13:673-9. [Crossref] [PubMed]
- Craven TP, Tsao CW, La Gerche A, et al. Exercise cardiovascular magnetic resonance: development, current utility and future applications. J Cardiovasc Magn Reson 2020;22:65. [Crossref] [PubMed]
- Gräfe D, Lacher M, Martynov I, et al. Pectus excavatum in motion: dynamic evaluation using real-time MRI. Eur Radiol 2023;33:2128-35. [Crossref] [PubMed]
- Ganescu O, LaRusso K, St-Louis E, et al. The utility of echocardiography and pulmonary function testing in the preoperative evaluation of pectus excavatum. J Pediatr Surg 2022;57:1561-6. [Crossref] [PubMed]
- Silbiger JJ, Parikh A. Pectus excavatum: echocardiographic, pathophysiologic, and surgical insights. Echocardiography 2016;33:1239-44. [Crossref] [PubMed]
- Laín A, Giralt G, Giné C, et al. Transesophageal echocardiography during pectus excavatum correction in children: What happens to the heart? J Pediatr Surg 2021;56:988-94. [Crossref] [PubMed]
- Töpper A, Polleichtner S, Zagrosek A, et al. Impact of surgical correction of pectus excavatum on cardiac function: insights on the right ventricle. A cardiovascular magnetic resonance study. Interact Cardiovasc Thorac Surg 2016;22:38-46. [Crossref] [PubMed]
- Raggio IM, Martínez-Ferro M, Bellía-Munzón G, et al. Diastolic and Systolic Cardiac Dysfunction in Pectus Excavatum: Relationship to Exercise and Malformation Severity. Radiol Cardiothorac Imaging 2020;2:e200011. [Crossref] [PubMed]
- Kelly RE Jr. Pectus excavatum: historical background, clinical picture, preoperative evaluation and criteria for operation. Semin Pediatr Surg 2008;17:181-93. [Crossref] [PubMed]
- van Dijk H, Höppener PF, Siebenga J, et al. Medical photography: a reliable and objective method for documenting the results of reconstructive surgery of pectus excavatum. J Vis Commun Med 2011;34:14-21. [Crossref] [PubMed]
- Ewert F, Syed J, Wagner S, et al. Does an external chest wall measurement correlate with a CT-based measurement in patients with chest wall deformities? J Pediatr Surg 2017;52:1583-90. [Crossref] [PubMed]
- Poncet P, Kravarusic D, Richart T, et al. Clinical impact of optical imaging with 3-D reconstruction of torso topography in common anterior chest wall anomalies. J Pediatr Surg 2007;42:898-903. [Crossref] [PubMed]
- Bliss DP Jr, Vaughan NA, Walk RM, et al. Non-Radiographic Severity Measurement of Pectus Excavatum. J Surg Res 2019;233:376-80. [Crossref] [PubMed]
- Taylor JS, Madhavan S, Szafer D, et al. Three-Dimensional Optical Imaging for Pectus Excavatum Assessment. Ann Thorac Surg 2019;108:1065-71. [Crossref] [PubMed]
- Scalabre A, Maniouloux F, Vermersch S, et al. Utility of radiation-free imaging for initial evaluation of pectus excavatum. Interact Cardiovasc Thorac Surg 2019;29:503-9. [Crossref] [PubMed]
- Hebal F, Port E, Hunter CJ, et al. A novel technique to measure severity of pediatric pectus excavatum using white light scanning. J Pediatr Surg 2019;54:656-62. [Crossref] [PubMed]
- Fuentes S, Pradillos-Serna JM, Berlioz M, et al. Validating 3D indexes in the non-surgical pectus excavatum patient. J Pediatr Surg 2021;56:230-4. [Crossref] [PubMed]
- Wang H, Liu W, Zhang DY, et al. Surface topography index: a novel deformity severity assessment index for pectus excavatum. Transl Pediatr 2021;10:2044-51. [Crossref] [PubMed]
- Glinkowski W, Sitnik R, Witkowski M, et al. Method of pectus excavatum measurement based on structured light technique. J Biomed Opt 2009;14:044041. [Crossref] [PubMed]
- Belgacem A, Tricard J, Dutoit A, et al. Efficiency of non-operative management for pectus deformities in children using an X-ray-free protocol. Interdiscip Cardiovasc Thorac Surg 2023;36:ivad093. [Crossref] [PubMed]
- Daemen JHT, Coorens NA, Hulsewé KWE, et al. Three-dimensional Surface Imaging for Clinical Decision Making in Pectus Excavatum. Semin Thorac Cardiovasc Surg 2022;34:1364-73. [Crossref] [PubMed]
- Coorens NA, Daemen JHT, Slump CH, et al. The Automatic Quantification of Morphological Features of Pectus Excavatum Based on Three-Dimensional Images. Semin Thorac Cardiovasc Surg 2022;34:772-81. [Crossref] [PubMed]
- Daemen JHT, Heuts S, Rezazadah Ardabili A, et al. Development of Prediction Models for Cardiac Compression in Pectus Excavatum Based on Three-Dimensional Surface Images. Semin Thorac Cardiovasc Surg 2023;35:202-12. [Crossref] [PubMed]
- Coorens NA, Daemen JHT, Slump CH, et al. Predicting Aesthetic Outcome of the Nuss Procedure in Patients with Pectus Excavatum. Semin Thorac Cardiovasc Surg 2023;35:627-37. [Crossref] [PubMed]