Optimal antithrombotic strategy following valve-in-valve transcatheter aortic and mitral valve replacement
Introduction
Antithrombotic therapy is administered in patients with bioprosthetic valves in order to prevent thromboembolic events and leaflet degeneration. Several randomized controlled trials (RCT) suggest that the optimal antithrombotic therapy for transcatheter aortic valve replacement (TAVR) of a native aortic valve is single antiplatelet therapy (SAPT) (1-3), provided that anticoagulation or dual antiplatelet (DAPT) therapy is not required for other reasons. Although the American College of Cardiology/American Heart Association (ACC/AHA) guidelines recommend the use of aspirin (4), it remains to be clarified whether aspirin or P2Y12 inhibitors, namely clopidogrel, are superior in these patients. For patients who require oral anticoagulation (OAC), a single anticoagulant agent appears to suffice (4,5).
Much less is known however when TAVR is performed in the aortic or mitral position in order to treat an existing, degenerated bioprosthesis [valve-in-valve-TAVR (ViV-TAVR) and ViV-transcatheter mitral valve replacement (ViV-TMVR) respectively]. The presence of extra bioprosthetic material as well as typically the smaller bioprosthesis size and higher gradients may predispose to higher rates of thromboembolic events and leaflet degeneration (6-10). Although the optimal antithrombotic strategy has not been adequately studied, these patients may benefit from DAPT or OAC. The aim of this review is to summarize technical considerations and discuss outcomes in relation to antithrombotic therapy after ViV-TAVR and ViV-TMVR.
ViV-TAVR leaflet thrombosis and related outcomes
Much of the information regarding outcomes of ViV-TAVR comes from retrospective databases (11-15), dedicated registries and/or subanalyses of native TAVR RCTs such as the Valve-in-Valve International Data (VIVID), the Transcatheter Valve Therapy (TVT), and the Placement of Aortic Transcatheter Valves (PARTNER) 2 ViV (6-8).
ViV-TAVR has been associated with increased residual mean valvular gradients ranging from ~15 to 20 mmHg (6-8), and with an increased incidence of hemodynamic valvular deterioration (defined as increase in mean valvular gradients >10 mmHg) (16,17) (Table 1). In comparison, mean valvular gradients following native TAVR range from ~8 to 12 mmHg depending on TAVR platform and size (6,22). It is important to note that patients with small native annuli who receive small prosthesis at the time of index surgical aortic valve replacement (SAVR) are predisposed to prosthesis-patient mismatch (PPM; defined as effective orifice area <0.65 cm2/m2 when severe) (23). Therefore, upfront surgical root enlargement or redo SAVR could avoid worsening hemodynamics associated with ViV-TAVR.
Table 1
Type of risk factors | TAVR | SAVR | ViV-TAVR |
---|---|---|---|
Technical/anatomical (reference) | THV size ≤23 mm (16,17)a | PPM (18)a | Porcine tissue valve (9)b |
ViV procedure (16,17,19)a,b | Porcine tissue valve (18)a | Decreased ratio of true ID/BSA (9)b | |
Severe PPM (17)a | Decreased ratio true ID/label size (9)b | ||
Mean AV gradient (17)a | Additional areas of stasis (neo-sinuses) (20)a,b | ||
Use of balloon-expandable valve (21)b | Increased residual valvular gradientsa,b | ||
Increased amount of excess tissuea,b | |||
Clinical (reference) | Increased BMI (16,17,21)a,b | HTN (18)a | Increased BSA (9)b |
Severe COPD (17)a | Creatinine >2.1 mg/dL at follow-up (18)a | Previous stroke (9)b | |
No anticoagulation at discharge (16)a | |||
Factor Xa inhibitor only at discharge (17)a | |||
Antiplatelet therapy alone at discharge (21)b |
a, valve degeneration; b, clinical valvular thrombosis. TAVR, transcatheter aortic valve replacement; SAVR, surgical aortic valve replacement; ViV, valve-in-valve; THV, transcatheter heart valve; PPM, patient-prosthesis mismatch; ID, inner diameter; BSA, body surface area; AV, aortic valve; BMI, body mass index; HTN, hypertension; COPD, chronic obstructive pulmonary disease.
Leaflet thrombosis has been described as one of the major underlying mechanisms of hemodynamic valve deterioration (16). Due to the nature of a ViV procedure, neo-sinuses between the leaflets of the new transcatheter heart valve (THV) and the stent frame of the surgical bioprosthesis are formed leading to additional areas of stasis (20). The location of the neo-sinuses is shown in Figure 1 (20). Clinical valvular thrombosis, shown in Figure 2 in a symptomatic patient, is a serious complication after ViV-TAVR (9,10,21). It is defined as valve dysfunction (residual mean valvular gradient >20 mmHg, aortic valve area <1.2 cm2, or new-onset valvular regurgitation more than mild) presenting secondary to thrombosis. Thrombosis is diagnosed based on imaging including transesophageal echocardiography (TEE) and four-dimensional computed tomography (4D-CT), often as a mobile mass attached to the leaflet characteristic of a thrombus in the absence of infection and based on its response to OAC (9). Analysis of the VIVID registry showed an incidence of clinical valvular thrombosis of 7.6% (9), which is markedly higher compared to an incidence of 0.61% in native TAVR (19). Moreover, failing surgical stented porcine bioprosthesis showed a higher risk of clinical valvular thrombosis compared to other valve types which was consistent with previous reports (9,21,24). Monitoring of valvular gradients is crucial to detect any gradual or sudden increases which could be indicative of leaflet thrombosis (9). The frequency of clinical valvular thrombosis in ViV-TAVR patients using direct oral anticoagulants (DOACs) or vitamin K antagonists (VKAs) was ~1% compared to 11% in patients not on an OAC (9). Figure 3 shows a representative case of clinical valvular thrombosis after ViV-TAVR and its resolution with the use of a VKA (9).
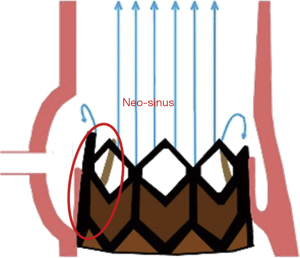
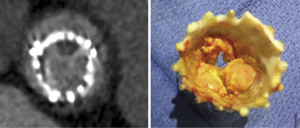
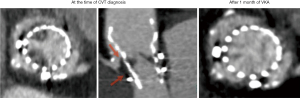
In addition to technical/anatomical risk factors, clinical characteristics have also been associated with the incidence of clinical valvular thrombosis and valve degeneration following bioprosthetic aortic valve (BAV) replacement (Table 1). An increased body mass index (BMI) has been shown to be a risk factor for both (16,17,21). Additionally, the presence of severe chronic obstructive pulmonary disease (COPD), hypertension, and elevated creatinine at follow-up have been associated with an increased rate of valvular degeneration (17,18). In a study comparing patients who developed clinical valvular thrombosis vs. patients who did not after ViV-TAVR, an increased body surface area (BSA) and an increased rate of stroke in the past medical history was shown in the thrombosis group (9).
In addition, TAVR has been associated with higher rates of subclinical valvular thrombosis (25-27). This is characterized by the occurrence of hypoattenuated leaflet thickening (HALT), graded based on the extent to which the leaflets are affected (Grade 0: 0%; Grade 1: ≤25%; Grade 2: 26% to 50%; Grade 3: 51% to 75%; Grade 4: >75%) and/or reduced leaflet motion (RLM), classified based on the extent of leaflet mobility [Grade 0: unrestricted; Grade 1: minimally restricted (<30%); Grade 2: mildly restricted (30% to 50%); Grade 3: moderately restricted (51% to 70%); Grade 4 (>70%)] as assessed by 4D cardiac computed tomography (25). According to the PARTNER 3 CT substudy, the rate of HALT after TAVR (13%) was significantly higher compared to SAVR (5%) within 30 days, with no difference at the one year follow up (27). Furthermore, the ATLANTIS-4D-CT substudy reported a numerically increased rate of ischemic events, a composite of death, myocardial infarction, stroke, and peripheral embolism, in patients with (10.7%) compared to patients without (7.1%) grade 3 or 4 HALT and/or RLM within 90 days (25). Even though the consequences of and the necessity to treat subclinical valvular thrombosis remain a topic of debate, the use of DOACs has been shown to potentially reduce subclinical valvular thrombosis compared to antiplatelet therapy alone after TAVR (25,26).
Patients with a clinical valvular thrombosis after ViV-TAVR have an increased rate of major stroke of 4.8% compared to patients without valvular thrombosis with a rate of 1.1% within 30 days following the procedure (9). Despite the higher risk of valve thrombosis, the overall rates of postprocedural stroke after ViV-TAVR do not appear to be higher compared to native TAVR. Stroke within 30 days and one year after ViV-TAVR ranged from 1.7% to 2.5% and 3.2% to 4.5%, respectively (6-8). In comparison, the rates of stroke following native TAVR within 30 days and one year in patients with similar Society of Thoracic Surgeons (STS) risk profiles from the PARTNER 2 trial were 5.5% and 8.0%, respectively (22). Figure 4 summarizes the rate of stroke within 30 days and one year following native TAVR in high- and intermediate-risk patients (28-31). In the TVT registry rates of stroke after native TAVR were 3.0% and 5.0%, compared to 1.7% and 3.2% following ViV-TAVR within 30 days and one year, respectively (7). A recent update from the PARTNER 2 ViV registry reported a five-year follow-up with a stroke rate of 10.5% (6). In contrast, the rate of stroke within five years in patients who underwent native TAVR was 15.3% (32).
Overall mortality within 30 days and one year was 2.7% and 11.8% in the PARTNER 2 ViV and continued access registry (6), 2.9% and 11.7% in the TVT registry (7), and 7.6% and 16.8% in the VIVID registry (8). In contrast, overall mortality within 30 days and one year following native TAVR ranged from 3.9% to 4.8% and 12.3% to 21.2%, respectively (7,32). Figure 4 shows a summary of all-cause and cardiovascular mortality within 30 days and one year following native TAVR in high- and intermediate-risk patients. In the VIVID registry, predictors of early mortality (≤30 days) were small surgical BAV (label inner diameter ≤21 mm), and predictors of late mortality (≤1 year) were baseline surgical bioprosthesis stenosis, emergent or salvage TAVR, creatinine >2 mg/dL or dialysis, nonfemoral access, anemia, and low platelet count (7,8). Studies reporting long-term outcomes after ViV-TAVR are limited. In the five-year follow-up from the PARTNER 2 ViV registry overall mortality was 50.6% with independent predictors including male sex, STS score, and small implanted THV (SAPIEN XT size 23 vs. 26 mm) (6). Following native TAVR, overall mortality after five years was 46.0% (32). Risk factors associated with decreased long-term survival after ViV-TAVR included smaller surgical BAVs (true inner diameter ≤20 mm), non-transfemoral access, age, lower baseline left ventricular ejection fraction (LVEF), chronic kidney disease (CKD), and diabetes mellitus (33).
Anticoagulation considerations
The higher rates of clinical valvular thrombosis after ViV-TAVR suggest that a more aggressive antithrombotic regimen may be useful in these patients. An analysis of the VIVID registry compared patients who developed clinical valvular thrombosis vs. those who did not after ViV-TAVR (9). The rate of clinical valvular thrombosis in ViV-TAVR patients using DOACs or VKAs was ~1% compared to 11% in patients not on an OAC (9). In the no thrombosis group, OAC was used in 35.2% [VKA in 29.7%; non-vitamin K antagonist oral anticoagulant (NOAC) in 5.5%] and antiplatelet therapy (SAPT or DAPT) was used in 63.4% of patients (9). In contrast, only 4.3% (VKA in all) of patients received OAC and 95.7% of patients received antiplatelet therapy (SAPT or DAPT) in the thrombosis group (9). The incidence of postprocedural stroke was significantly decreased in the no thrombosis group with 1.1% compared to the thrombosis group with 4.8% (9). Another study comparing ViV-TAVR and native TAVR regarding the risk of coronary obstruction, reported the use of either antiplatelet therapy or OAC (34). In the ViV-TAVR group, 76.0% of patients received SAPT (aspirin in 52.7%; P2Y12 inhibitor in 23.3%) and only 6.2% of patients received OAC (34). Similarly, in the native TAVR group, SAPT was used in 64.4% (aspirin in 45.0%; P2Y12 inhibitor in 19.4%) and OAC was used in 5.4% of patients (34).
However, data remains limited and current clinical guidelines only include antithrombotic recommendations for native TAVR without a specific protocol for ViV-TAVR (4,5). Several RCTs have demonstrated that OAC is associated with worse clinical outcomes compared to SAPT or DAPT in patients undergoing native TAVR without other indications for OAC (35-37). When OAC is required, DOACs may be superior to VKAs (38,39). Current guidelines for native TAVR recommend SAPT and OAC therapy for patients without and with other indications for OAC, respectively (4,5). Both the ACC/AHA and the European Society for Cardiology/European Association for Cardiothoracic Surgery (ESC/EACTS) guidelines are shown in detail in Table 2 (4,5). The applicability of these guidelines to ViV-TAVR is unclear and there are important factors that need to be considered when choosing the optimal antithrombotic therapy. So far, this procedure is only performed in high-risk patients who are usually at an advanced age and often have multiple comorbidities (40). Also, the risk of valvular thrombosis and its implications on BAV durability and patient survival are critical factors impacting the ideal antithrombotic treatment (9).
Table 2
Guidelines & year (reference) | Recommendations | Class/level of evidence |
---|---|---|
ACC/AHA 2020 (4) | Aspirin 75 to 100 mg daily is reasonable in the absence of other indications for OAC | IIa/B |
For patients who are at low risk of bleeding, DAPT with aspirin 75 to 100 mg and clopidogrel 75 mg may be reasonable for 3 to 6 months | IIb/B | |
For patients who are at low risk of bleeding, OAC with a VKA to achieve an INR of 2.5 may be reasonable for at least 3 months | IIb/B | |
ESC/EACTS 2021 (5) | Lifelong SAPT is recommended in patients with no baseline indication for OAC | I/A |
OAC is recommended lifelong for patients who have other indications for OAC | I/B |
ACC, American College of Cardiology; AHA, American Heart Association; ESC, European Society for Cardiology; EACTS, European Association for Cardiothoracic Surgery; OAC, oral anticoagulation; DAPT, dual antiplatelet therapy; VKA, vitamin K antagonist; INR, international normalized ratio; SAPT, single antiplatelet therapy.
ViV-TMVR leaflet thrombosis and related outcomes
Similar to ViV-TAVR, most of the current data on ViV-TMVR is derived from retrospective series and analyses of large registries such as VIVID, TVT, and the international TMVR (41-46). The ongoing MITRAL trial is the first prospective randomized clinical trial investigating the safety and feasibility of ViV-TMVR (47).
ViV-TMVR is associated with substantial residual mean valvular gradients ranging from 5.6 to 7.3 mmHg (44-46). Analysis of the TMVR registry showed an incidence of clinical valvular thrombosis of 6.6% vs. 1.6% within one year in patients with vs. without OAC therapy, respectively (44). Even though the study also included valve-in-ring (ViR)- and valve-in-mitral annular calcification (ViMAC)-TMVR, 90.9% of the thrombosis occurred in ViV-TMVR patients (44). Of note, of the patients who suffered from clinical valvular thrombosis 90% had a porcine and 10% had a pericardial surgical bioprosthetic mitral valve (44). An example of clinical valvular thrombosis following ViV-TMVR is shown in Figure 5 (48).

The rate of postprocedural stroke after ViV-TMVR ranged from 1.1% to 2.3% within 30 days which did not markedly increase at one year reaching 3.3% (44-46). In the ongoing MITRAL trial, the rates of postprocedural stroke within 30 days and one year after ViV-TMVR was 3.3% and 6.7%, respectively, with no additional cerebrovascular events reported at two years (47). In contrast, 3.3% of patients who underwent a redo SMVR suffered a stroke (49). Moreover, the rates of postprocedural stroke within 30 days and one year after native TAVR were 5.5% and 8.0%, respectively, in the PARTNER 2 trial and 3.0% and 5.0%, respectively, in the TVT registry (7,22).
Overall mortality at 30 days and one year was 6.2% and 14% in the TMVR registry (44), 6.5% and 13.8% in the VIVID registry (45), and 5.4% and 16.7% in the TVT registry (46). In the MITRAL trial overall mortality was 3.3% within 30 days and one year and 6.7% at two-year follow-up (47). In comparison, overall mortality within 30 days and one year after redo SMVR was 3.4% and 11.9%, respectively, and after native TAVR it ranged from 3.9% to 4.8% and 12.3% to 21.2%, respectively (7,32,49). Functional status and quality of life increased substantially and remained stable until the end of the follow-up period (47); 85.2% of patients were New York Heart Association (NYHA) class I or II at two years compared to 80% of patients in NYHA class III or IV at baseline (47). Data regarding long-term outcomes after ViV-TMVR are still very limited. Currently the analysis of the VIVID registry presents the longest follow-up with four years (45). Overall mortality was 37.5% with independent predictors including age, NYHA class IV, baseline pulmonary artery systolic pressure, chronic lung disease, and CKD (45).
Anticoagulation considerations
A low rate of clinical valvular thrombosis at one year (0.5%) was reported in a study of the TVT registry where most patients (80.8%) were discharged on OAC (46). These results suggest the potential value of postprocedural OAC also after ViV-TMVR. However, evidence regarding antithrombotic therapy after dedicated TMVR is limited and clinical trials investigating DOACs in transcatheter treatment of structural heart disease are mainly limited to TAVR. With a comparable risk of postprocedural stroke in ViV-TMVR compared to ViV-TAVR and a seemingly increased risk of valvular thrombosis with biological prosthesis in mitral vs. aortic position (50,51), results from clinical trials regarding TAVR should be considered. Moreover, a large proportion of patients undergoing ViV-TMVR suffer from atrial fibrillation (43,44). Therefore, DOACs, especially apixaban, might be useful in patients undergoing this procedure. In addition, the recently conducted RIVER trial investigated the use of rivaroxaban compared to warfarin in patients with a bioprosthetic mitral valve and atrial fibrillation (52). The results showed non-inferiority of rivaroxaban in respect to the time to occurrence of major cardiovascular events, death, and major bleeding at twelve months (52). Based on the early termination of the GALILEO trial, low-dose rivaroxaban together with aspirin is not recommended in patients with BAVs (4). However, at the dose of 20 mg used in the RIVER trial and in the setting of atrial fibrillation it might be useful after mitral valve replacement.
Current guidelines for antithrombotic therapy after mitral valve replacement are only based on surgical mitral valve replacement without specific protocols for TMVR and specific recommendations for ViV-TMVR are lacking (4,5). The ACC/AHA guidelines recommend SAPT and the ESC/EACTS recommend OAC therapy for patients without and both guidelines recommend OAC therapy for patients with other indications for OAC treatment (4,5). The detailed recommendations of both guidelines for surgical mitral valve replacement are shown in Table 3 (4,5). The extent to which these guidelines can be used in the setting of ViV-TMVR and how they need to be potentially modified has not been clarified. As for ViV-TAVR, the patient population undergoing this procedure consists mainly of older patients who suffer from multiple comorbidities (53). Additionally, valvular hemodynamics and the risk of thrombosis have important implications on the ideal antithrombotic treatment strategy (54).
Table 3
Guidelines & year (Ref) | Recommendations | Class/level of evidence |
---|---|---|
ACC/AHA 2020 (4) | Aspirin 75 to 100 mg daily is reasonable in the absence of other indications for OAC | IIa/B |
For patients at low risk of bleeding, OAC with a VKA to achieve an INR of 2.5 is reasonable for at least 3 months and for as long as 6 months | IIa/B | |
ESC/EACTS 2021 (5) | In patients with no baseline indication for OAC, low-dose aspirin (75–100 mg/day) or OAC using a VKA should be considered for the first 3 months | IIa/B |
OAC is recommended for patients who have other indications for OAC | I/C | |
NOACs should be considered over VKA after 3 months in patients with AF | IIa/B |
ACC, American College of Cardiology; AHA, American Heart Association; ESC, European Society for Cardiology; EACTS, European Association for Cardiothoracic Surgery; OAC, oral anticoagulation; VKA, vitamin K antagonist; INR, international normalized ratio; NOAC, non-vitamin K antagonist oral anticoagulant; AF, atrial fibrillation.
Anticoagulation and bleeding complications
While a more aggressive antithrombotic strategy may mitigate the risk of clinical valvular thrombosis and stroke following ViV-TAVR and ViV-TMVR, it also increases the risk of bleeding. This is well known from the use of OAC and additional clopidogrel among patients that undergo native TAVR. Among patients that underwent native TAVR in the large GALILEO trial, the rate of major, life-threatening, or disabling bleeding within one year among patients with no other indication for OAC was 5.8% with OAC (rivaroxaban) vs. 3.9% with SAPT (aspirin). Aspirin or clopidogrel was used in each group, respectively, as a second agent for the first three months after TAVR (37). In fact, the primary outcome of death or a first thromboembolic event was higher in the OAC group and the trial was prematurely terminated. Similar results were noted in the second stratum of the ATLANTIS trial (35). Together, these results indicate that routine OAC should not be administered in all patients after native TAVR that have no other indication for OAC. Similarly, the addition of clopidogrel among patients who receive OAC resulted in more frequent serious bleeding in the POPULAR TAVI trial (3). Finally, subanalyses of large trials on native TAVR that were not powered for bleeding complication indicate similar findings (32).
Among patients who undergo ViV-TAVR, there are no dedicated RCTs that were powered to compare bleeding rates with additional antithrombotic agents. However, the risk of bleeding with additional antithrombotic agents can be expected to increase. The key point is whether this counteracts the risk of thrombosis and prosthesis degeneration. In the analysis of the VIVID registry, patients who developed clinical valvular thrombosis had lower rates of major bleeding vs. those who did not develop thrombosis but were more frequently on OAC (0% vs. 3.3% of patients, respectively) (9). Of note, the specific antithrombotic medication used in the patients who had a bleeding complication was not reported.
It is important to note that an advantage of DOACs is that blood level monitoring is not required. However, if patients develop thrombosis on DOACs, switching to a VKA with international normalized ratio (INR) monitoring might be needed. The risk of bleeding may not be significantly different when using DOAC vs. VKA, according to the ATLANTIS trial (35) but other data suggest higher rates of bleeding in non-TAVR settings. Finally, the risk of bleeding may not be different when DOAC vs. DAPT is used according to the ADAPT-TAVR trial (36).
Conclusions
Whereas clinical thrombosis can occur more frequently after ViV-TAVR or ViV-TMVR compared to replacement of native valves, the optimal antithrombotic treatment strategy following these procedures remains an area of debate. Retrospective registry analyses suggest that OAC can reduce the risk of valvular thrombosis. This benefit though has to be balanced with the risk of bleeding. Clinical trials including patients undergoing ViV-TAVR and ViV-TMVR are needed to determine the applicability of these results to these procedures.
Acknowledgments
Funding: None.
Footnote
Peer Review File: Available at https://jtd.amegroups.com/article/view/10.21037/jtd-23-1313/prf
Conflicts of Interest: All authors have completed the ICMJE uniform disclosure form (available at https://jtd.amegroups.com/article/view/10.21037/jtd-23-1313/coif). I.G. reports the following disclosures: consultant (honoraria) – Zimmer Biomet, Atricure, Neosurgery, Neptune Medical, Abbvie, Johnson & Johnson, Boston Scientific, Edwards Lifesciences, Medtronic, Help-TheraX, 3ive, Encompass, Summus Medical, Abbott SJM. Advisory Boards: Edwards Surgical, Medtronic Surgical, Medtronic Structural Mitral & Tricuspid, Trisol Medical, Abbvie, Johnson & Johnson, Foldax Medical, Zimmer Biomet, Neosurgery, Abbvie, Boston Scientific, Summus Medical. Equity: Valcare Medical, Durvena, CardioMech, Vdyne, MitreMedical, MITRx. Institutional funding to Columbia University: Edwards Lifesciences, Medtronic, Abbott Vascular, Boston Scientific, JenaValve. The other authors have no conflicts of interest to declare.
Ethical Statement: The authors are accountable for all aspects of the work in ensuring that questions related to the accuracy or integrity of any part of the work are appropriately investigated and resolved.
Open Access Statement: This is an Open Access article distributed in accordance with the Creative Commons Attribution-NonCommercial-NoDerivs 4.0 International License (CC BY-NC-ND 4.0), which permits the non-commercial replication and distribution of the article with the strict proviso that no changes or edits are made and the original work is properly cited (including links to both the formal publication through the relevant DOI and the license). See: https://creativecommons.org/licenses/by-nc-nd/4.0/.
References
- Ussia GP, Scarabelli M, Mulè M, et al. Dual antiplatelet therapy versus aspirin alone in patients undergoing transcatheter aortic valve implantation. Am J Cardiol 2011;108:1772-6. [Crossref] [PubMed]
- Rodés-Cabau J, Masson JB, Welsh RC, et al. Aspirin Versus Aspirin Plus Clopidogrel as Antithrombotic Treatment Following Transcatheter Aortic Valve Replacement With a Balloon-Expandable Valve: The ARTE (Aspirin Versus Aspirin + Clopidogrel Following Transcatheter Aortic Valve Implantation) Randomized Clinical Trial. JACC Cardiovasc Interv 2017;10:1357-65. [Crossref] [PubMed]
- Brouwer J, Nijenhuis VJ, Delewi R, et al. Aspirin with or without Clopidogrel after Transcatheter Aortic-Valve Implantation. N Engl J Med 2020;383:1447-57. [Crossref] [PubMed]
- Otto CM, Nishimura RA, Bonow RO, et al. 2020 ACC/AHA Guideline for the Management of Patients With Valvular Heart Disease: Executive Summary: A Report of the American College of Cardiology/American Heart Association Joint Committee on Clinical Practice Guidelines. Circulation 2021;143:e35-71. [Crossref] [PubMed]
- Vahanian A, Beyersdorf F, Praz F, et al. 2021 ESC/EACTS Guidelines for the management of valvular heart disease. Eur Heart J 2022;43:561-632. [Crossref] [PubMed]
- Hahn RT, Webb J, Pibarot P, et al. 5-Year Follow-Up From the PARTNER 2 Aortic Valve-in-Valve Registry for Degenerated Aortic Surgical Bioprostheses. JACC Cardiovasc Interv 2022;15:698-708. [Crossref] [PubMed]
- Tuzcu EM, Kapadia SR, Vemulapalli S, et al. Transcatheter Aortic Valve Replacement of Failed Surgically Implanted Bioprostheses: The STS/ACC Registry. J Am Coll Cardiol 2018;72:370-82. [Crossref] [PubMed]
- Dvir D, Webb JG, Bleiziffer S, et al. Transcatheter aortic valve implantation in failed bioprosthetic surgical valves. JAMA 2014;312:162-70. [Crossref] [PubMed]
- Abdel-Wahab M, Simonato M, Latib A, et al. Clinical Valve Thrombosis After Transcatheter Aortic Valve-in-Valve Implantation. Circ Cardiovasc Interv 2018;11:e006730. [Crossref] [PubMed]
- Aktuerk D, Mirsadraee S, Quarto C, et al. Leaflet thrombosis after valve-in-valve transcatheter aortic valve implantation: a case series. Eur Heart J Case Rep 2020;4:1-6. [Crossref] [PubMed]
- Piazza N, Bleiziffer S, Brockmann G, et al. Transcatheter aortic valve implantation for failing surgical aortic bioprosthetic valve: from concept to clinical application and evaluation (part 2). JACC Cardiovasc Interv 2011;4:733-42. [Crossref] [PubMed]
- Linke A, Woitek F, Merx MW, et al. Valve-in-valve implantation of Medtronic CoreValve prosthesis in patients with failing bioprosthetic aortic valves. Circ Cardiovasc Interv 2012;5:689-97. [Crossref] [PubMed]
- Silaschi M, Wendler O, Seiffert M, et al. Transcatheter valve-in-valve implantation versus redo surgical aortic valve replacement in patients with failed aortic bioprostheses. Interact Cardiovasc Thorac Surg 2017;24:63-70. [Crossref] [PubMed]
- Matta A, Levai L, Roncalli J, et al. Comparison of in-hospital outcomes and long-term survival for valve-in-valve transcatheter aortic valve replacement versus the benchmark native valve transcatheter aortic valve replacement procedure. Front Cardiovasc Med 2023;10:1113012. [Crossref] [PubMed]
- Webb JG, Wood DA, Ye J, et al. Transcatheter valve-in-valve implantation for failed bioprosthetic heart valves. Circulation 2010;121:1848-57. [Crossref] [PubMed]
- Del Trigo M, Muñoz-Garcia AJ, Wijeysundera HC, et al. Incidence, Timing, and Predictors of Valve Hemodynamic Deterioration After Transcatheter Aortic Valve Replacement: Multicenter Registry. J Am Coll Cardiol 2016;67:644-55. [Crossref] [PubMed]
- Vemulapalli S, Holmes DR Jr, Dai D, et al. Valve hemodynamic deterioration and cardiovascular outcomes in TAVR: A report from the STS/ACC TVT Registry. Am Heart J 2018;195:1-13. [Crossref] [PubMed]
- Nitsche C, Kammerlander AA, Knechtelsdorfer K, et al. Determinants of Bioprosthetic Aortic Valve Degeneration. JACC Cardiovasc Imaging 2020;13:345-53. [Crossref] [PubMed]
- Latib A, Naganuma T, Abdel-Wahab M, et al. Treatment and clinical outcomes of transcatheter heart valve thrombosis. Circ Cardiovasc Interv 2015;8:e001779. [Crossref] [PubMed]
- Hatoum H, Gooden SCM, Sathananthan J, et al. Neosinus and Sinus Flow After Self-Expanding and Balloon-Expandable Transcatheter Aortic Valve Replacement. JACC Cardiovasc Interv 2021;14:2657-66. [Crossref] [PubMed]
- Jose J, Sulimov DS, El-Mawardy M, et al. Clinical Bioprosthetic Heart Valve Thrombosis After Transcatheter Aortic Valve Replacement: Incidence, Characteristics, and Treatment Outcomes. JACC Cardiovasc Interv 2017;10:686-97. [Crossref] [PubMed]
- Reardon MJ, Van Mieghem NM, Popma JJ, et al. Surgical or Transcatheter Aortic-Valve Replacement in Intermediate-Risk Patients. N Engl J Med 2017;376:1321-31. [Crossref] [PubMed]
- Fallon JM, DeSimone JP, Brennan JM, et al. The Incidence and Consequence of Prosthesis-Patient Mismatch After Surgical Aortic Valve Replacement. Ann Thorac Surg 2018;106:14-22. [Crossref] [PubMed]
- Gandolfo C, Turrisi M, Follis F, et al. Acute Obstructive Thrombosis of Sapien 3 Valve After Valve-in-Valve Transcatheter Aortic Valve Replacement for Degenerated Mosaic 21 Valve. JACC Cardiovasc Interv 2018;11:215-7. [Crossref] [PubMed]
- Montalescot G, Redheuil A, Vincent F, et al. Apixaban and Valve Thrombosis After Transcatheter Aortic Valve Replacement: The ATLANTIS-4D-CT Randomized Clinical Trial Substudy. JACC Cardiovasc Interv 2022;15:1794-804. [Crossref] [PubMed]
- De Backer O, Dangas GD, Jilaihawi H, et al. Reduced Leaflet Motion after Transcatheter Aortic-Valve Replacement. N Engl J Med 2020;382:130-9. [Crossref] [PubMed]
- Makkar RR, Blanke P, Leipsic J, et al. Subclinical Leaflet Thrombosis in Transcatheter and Surgical Bioprosthetic Valves: PARTNER 3 Cardiac Computed Tomography Substudy. J Am Coll Cardiol 2020;75:3003-15. [Crossref] [PubMed]
- Smith CR, Leon MB, Mack MJ, et al. Transcatheter versus surgical aortic-valve replacement in high-risk patients. N Engl J Med 2011;364:2187-98. [Crossref] [PubMed]
- Leon MB, Smith CR, Mack MJ, et al. Transcatheter or Surgical Aortic-Valve Replacement in Intermediate-Risk Patients. N Engl J Med 2016;374:1609-20. [Crossref] [PubMed]
- Popma JJ, Adams DH, Reardon MJ, et al. Transcatheter aortic valve replacement using a self-expanding bioprosthesis in patients with severe aortic stenosis at extreme risk for surgery. J Am Coll Cardiol 2014;63:1972-81. [Crossref] [PubMed]
- Adams DH, Popma JJ, Reardon MJ, et al. Transcatheter aortic-valve replacement with a self-expanding prosthesis. N Engl J Med 2014;370:1790-8. [Crossref] [PubMed]
- Makkar RR, Thourani VH, Mack MJ, et al. Five-Year Outcomes of Transcatheter or Surgical Aortic-Valve Replacement. N Engl J Med 2020;382:799-809. [Crossref] [PubMed]
- Bleiziffer S, Simonato M, Webb JG, et al. Long-term outcomes after transcatheter aortic valve implantation in failed bioprosthetic valves. Eur Heart J 2020;41:2731-42. [Crossref] [PubMed]
- Koren O, Patel V, Naami R, et al. New adverse coronary events in valve-in-valve TAVR and native TAVR-A 2-year matched cohort. Front Cardiovasc Med 2022;9:1004103. [Crossref] [PubMed]
- Collet JP, Van Belle E, Thiele H, et al. Apixaban vs. standard of care after transcatheter aortic valve implantation: the ATLANTIS trial. Eur Heart J 2022;43:2783-97. [Crossref] [PubMed]
- Park DW, Ahn JM, Kang DY, et al. Edoxaban Versus Dual Antiplatelet Therapy for Leaflet Thrombosis and Cerebral Thromboembolism After TAVR: The ADAPT-TAVR Randomized Clinical Trial. Circulation 2022;146:466-79. [Crossref] [PubMed]
- Dangas GD, Tijssen JGP, Wöhrle J, et al. A Controlled Trial of Rivaroxaban after Transcatheter Aortic-Valve Replacement. N Engl J Med 2020;382:120-9. [Crossref] [PubMed]
- Kawashima H, Watanabe Y, Hioki H, et al. Direct Oral Anticoagulants Versus Vitamin K Antagonists in Patients With Atrial Fibrillation After TAVR. JACC Cardiovasc Interv 2020;13:2587-97. [Crossref] [PubMed]
- Van Mieghem NM, Unverdorben M, Hengstenberg C, et al. Edoxaban versus Vitamin K Antagonist for Atrial Fibrillation after TAVR. N Engl J Med 2021;385:2150-60. [Crossref] [PubMed]
- Carroll JD, Mack MJ, Vemulapalli S, et al. STS-ACC TVT Registry of Transcatheter Aortic Valve Replacement. J Am Coll Cardiol 2020;76:2492-516. [Crossref] [PubMed]
- Cheung A, Webb JG, Barbanti M, et al. 5-year experience with transcatheter transapical mitral valve-in-valve implantation for bioprosthetic valve dysfunction. J Am Coll Cardiol 2013;61:1759-66. [Crossref] [PubMed]
- Eleid MF, Whisenant BK, Cabalka AK, et al. Early Outcomes of Percutaneous Transvenous Transseptal Transcatheter Valve Implantation in Failed Bioprosthetic Mitral Valves, Ring Annuloplasty, and Severe Mitral Annular Calcification. JACC Cardiovasc Interv 2017;10:1932-42. [Crossref] [PubMed]
- Urena M, Brochet E, Lecomte M, et al. Clinical and haemodynamic outcomes of balloon-expandable transcatheter mitral valve implantation: a 7-year experience. Eur Heart J 2018;39:2679-89. [Crossref] [PubMed]
- Yoon SH, Whisenant BK, Bleiziffer S, et al. Outcomes of transcatheter mitral valve replacement for degenerated bioprostheses, failed annuloplasty rings, and mitral annular calcification. Eur Heart J 2019;40:441-51. [Crossref] [PubMed]
- Simonato M, Whisenant B, Ribeiro HB, et al. Transcatheter Mitral Valve Replacement After Surgical Repair or Replacement: Comprehensive Midterm Evaluation of Valve-in-Valve and Valve-in-Ring Implantation From the VIVID Registry. Circulation 2021;143:104-16. [Crossref] [PubMed]
- Whisenant B, Kapadia SR, Eleid MF, et al. One-Year Outcomes of Mitral Valve-in-Valve Using the SAPIEN 3 Transcatheter Heart Valve. JAMA Cardiol 2020;5:1245-52. [Crossref] [PubMed]
- Eleid MF, Wang DD, Pursnani A, et al. 2-Year Outcomes of Transcatheter Mitral Valve Replacement in Patients With Annular Calcification, Rings, and Bioprostheses. J Am Coll Cardiol 2022;80:2171-83. [Crossref] [PubMed]
- Quick S, Speiser U, Strasser RH, et al. First bioprosthesis thrombosis after transcatheter mitral valve-in-valve implantation: diagnosis and treatment. J Am Coll Cardiol 2014;63:e49. [Crossref] [PubMed]
- Kamioka N, Babaliaros V, Morse MA, et al. Comparison of Clinical and Echocardiographic Outcomes After Surgical Redo Mitral Valve Replacement and Transcatheter Mitral Valve-in-Valve Therapy. JACC Cardiovasc Interv 2018;11:1131-8. [Crossref] [PubMed]
- Dangas GD, Weitz JI, Giustino G, et al. Prosthetic Heart Valve Thrombosis. J Am Coll Cardiol 2016;68:2670-89. [Crossref] [PubMed]
- Egbe AC, Pislaru SV, Pellikka PA, et al. Bioprosthetic Valve Thrombosis Versus Structural Failure: Clinical and Echocardiographic Predictors. J Am Coll Cardiol 2015;66:2285-94. [Crossref] [PubMed]
- Guimarães HP, Lopes RD, de Barros E, Silva PGM, et al. Rivaroxaban in Patients with Atrial Fibrillation and a Bioprosthetic Mitral Valve. N Engl J Med 2020;383:2117-26. [Crossref] [PubMed]
- Mack M, Carroll JD, Thourani V, et al. Transcatheter Mitral Valve Therapy in the United States: A Report from the STS/ACC TVT Registry. Ann Thorac Surg 2022;113:337-65. [Crossref] [PubMed]
- Capretti G, Urena M, Himbert D, et al. Valve Thrombosis After Transcatheter Mitral Valve Replacement. J Am Coll Cardiol 2016;68:1814-5. [Crossref] [PubMed]