Phase I/II study to evaluate consolidative hypofractionated radiation therapy for boosting the residual primary disease in combination with durvalumab after definitive chemoradiation therapy for stage III non-small cell lung cancer (NSCLC): study protocol for a prospective trial
Introduction
Background
Worldwide, lung cancer has been the most common cancer in the past few decades. In 2018, there were an estimated 2.1 million new cases, representing 11.6% of all new cancers (1). It is also the most common cause of death from cancer, with 1.8 million deaths (18.4% of the total). Non-small cell lung cancer (NSCLC) represents approximately 80% to 85% of all lung cancers and 30% of patients present with Stage III disease.
The management of stage III NSCLC is rapidly evolving (2,3). A meta-analysis of concurrent versus sequential chemoradiotherapy for unresectable stage III disease showed better outcomes with concurrent therapy, but even with concurrent chemoradiotherapy 5-year overall survival (OS) is approximately 15% (4). Recently, results from the phase III PACIFIC trial have been reported comparing consolidative durvalumab vs. placebo after definitive chemoradiation therapy (dCRT) in patients with stage III unresectable NSCLC (5,6). Median progression-free survival (PFS) and OS improvements were seen for patients who received consolidative durvalumab therapy (17.2 vs. 5.6 months) (47.5 vs. 29.1 months) respectively (6). Thus, further exploring other consolidative therapies with durvalumab in an attempt to further extend disease control and improve OS in patients with stage III NSCLC is warranted.
Radiation therapy (RT) has long been indicated to induce immune response as demonstrated by the abscopal effect, in which a systemic tumor response has been observed after local RT (7). Although previously thought to be an infrequent event, increasing cases of abscopal effects have been reported since immune checkpoint inhibitors were introduced along with recently increased use of stereotactic body radiotherapy (SBRT), which raised new interests in exploring immunoradiotherapy strategies (8). A wide variety of RT schemes have been used in combination with immunotherapy in preclinical studies, ranging from conventional/low dose fractionation of 1.8 to 5 Gy/fraction, to intermediate dose per fraction (6 Gy/fraction ×5 fractions, 8 Gy/fraction ×3 fractions, etc.), to high dose in single fraction (12–30 Gy per fraction) (9-12). RT in general was able to induce cell surface markers such as Fas, MHC I, ICAM-1 (11-18). Most of the animal model studies showed that the optimal increase in cell surface receptor expression resulted single dose of radiation greater than or equal to 8 Gy rather than conventional/low dose fractionation (11,12). Furthermore, it is thought that large single fraction regimens have a higher likelihood of altering the tumor microenvironment by facilitating infiltration of host immune cells such as macrophages, dendritic cells, or tumor antigen specific cytotoxic T lymphocytes (CTLs) (12). These results explained that in pre-clinical settings higher dose per fraction of RT such as the dose regimen used for SBRT was found to be more likely to be immunostimulating than conventional fractionated RT (13,14). However, radiation dose and fractionation schedules for optimal synergy between radiotherapy and immunotherapy are not well defined in clinical settings (15-18).
Rationale and knowledge gap
Single institutional protocols have suggested a benefit to a consolidative hypofractionated boost following dCRT (19). Unfortunately, no protocol to our knowledge has consolidative durvalumab with a consolidative hypofractionated radiotherapy boost. The synergistic effect of hypofractionated radiotherapy with durvalumab remains understudied. We thus propose to conduct a phase I/II clinical trial testing a consolidative RT using a regimen within this range with a concurrent programmed death-ligand 1 (PD-L1) antibody, durvalumab, in nonresectable stage III NSCLC, after definitive chemoradiation therapy (CRT). This is a trial designed as a follow-up study to the recently completed PACIFIC trial.
We hypothesize that combining consolidative hypofractionated radiation therapy (hfRT) regimen of 10 Gy ×2 fractions for boosting the residual primary lung cancer with adjuvant anti-PD-L1 therapy will further improve immunostimulation and provide better tumor control locoregionally and distantly than either modality alone.
Objectives
The primary objectives of the study are to evaluate the safety and efficacy of combing hfRT and durvalumab following dCRT. Safety is determined by assessing adverse events (AEs) throughout the entire study course while 12-month PFS according to RECIST 1.1 will be evaluated for treatment efficacy (20). Secondary endpoints include 18-month PFS, 12-month OS, rates of local control (LC), locoregional control (LRC), distant metastasis (DM), time to distant metastases (TTDM), and patient reported quality of life (QOL) during and following the treatment course. To explore the potential systemic effects from local hfRT boost with concurrent durvalumab, we also propose for exploratory aims to evaluate the effects of combined treatment on tumor microenvironment from up to four patients that will be consented for residual primary tumor biopsy two months after hfRT to assess exploratory markers which may include but is not limited to: immune cell gene expression profiles within the tumoral compartments, the presence of tumor necrosis factor (IFN)-γ, IFN-α, interleukin (IL)-2, IL-6, IL-8, IL-10, and IL-12 as well as expression of PD-L1 and the number and phenotype of immune cells such as T-cells, M1 and M2 subtypes of macrophage, and dendritic cells by immunohistochemistry methods. It is also proposed to evaluate the systemic effects of combined therapy by testing circulating tumor RNA/DNA, soluble PD-L1 and cytokine levels in peripheral blood specimen. Correlation of these biomarkers with response to durvalumab/hfRT treatment and/or the progression of cancer and/or toxicities will be performed.
Methods
This is a single center, phase I/II trial designed to evaluate safety and efficacy of consolidative hfRT with durvalumab following dCRT in subjects with unresectable stage III NSCLC.
Eligibility criteria
Subjects diagnosed with clinical stage III [American Joint Committee on Cancer (AJCC) 8th ed.] based on standard criteria including positron emission tomography/computed tomography (PET/CT) and brain magnetic resonance imaging (MRI) (Table S1) and pathologically confirmed NSCLC (including squamous cell carcinoma, adenocarcinoma, large-cell carcinoma, or NSCLC not otherwise specified) who are eligible or have undergone dCRT to 5,700–6,300 cGy (conventionally fractionated radiotherapy) with at least 2 cycles of platinum-based concurrent chemotherapy are assessed for eligibility (21) (see Figure 1). Additionally, eligibility criteria include: age >19 years, Eastern Cooperative Oncology Group (ECOG) performance status of 0 or 1 at time of enrollment, adequate normal organ and marrow function, and willingness to comply with the protocol. Following completion of dCRT (5–10 days following the end of dCRT as assessed by a CT chest/abdomen/pelvis with IV contrast or PET/CT), subjects with CR (no target for planning hfRT) or tumor size in lung parenchyma >5 cm in the largest axis (expecting high toxicities with hfRT) will not be eligible to enroll or continue in the study.
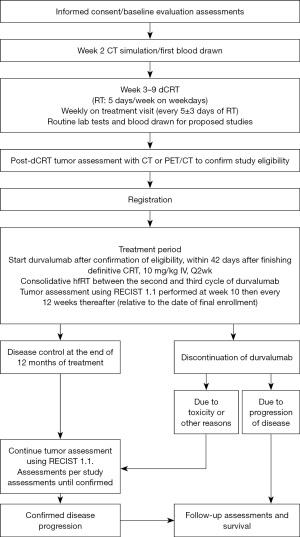
Interventions
dCRT
Subjects should receive a course of dCRT prior to the final study eligibility confirmation. Subjects should receive treatment 5 days per week, 1 fraction per day, 1.8–2 Gy per fraction to a total dose of 59.8 to 60 Gy. However, a total dose of 57 to 63 Gy is allowed for subjects to be enrolled to current trial. 3D conformal radiation therapy (3DCRT) or intensity modulated radiation therapy (IMRT) can be used per physician’s choice for RT planning but prefer tighter dose constraints (Table S2). Chemotherapy should be given concurrently with radiation therapy per standard care and institutional preference. More specifically, these subjects should receive two or more cycles (defined according to local practice) of platinum-based doublet chemotherapy (containing etoposide, vinblastine, vinorelbine, a taxane [paclitaxel or docetaxel], or pemetrexed) concurrently with RT. No adjuvant chemotherapy after dCRT is allowed. Dosimetric review of the dCRT plan is required to ensure that minimal dosimetric constraints are met (Table S3).
Durvalumab
The subject will start consolidative/adjuvant durvalumab within 42 days after the end of dCRT phase at 10 mg/kg body weight intravenously every two weeks to match the protocol of PACIFIC trial so that results would be comparable (5). Consolidative hfRT to the residual lung mass will be delivered between the second and third cycle of durvalumab if no significant toxicities (> Grade 2) occurred from immunotherapy.
hfRT
All subjects must undergo radiotherapy planning based on CT in the treatment position with the acquisition of a four-dimensional CT (4D-CT) for evaluation of respiratory motion (see Table S3). The target lesion will be outlined by an appropriately trained physician and designated the gross tumor volume (GTV). A PET/CT rather than a CT chest is preferably performed within 5–10 days after completion of dCRT to help guide GTV delineation by including only the solid tumor volume of standardized uptake value (SUV) >4 to avoid contouring atelectasis or treatment-related inflammation which usually has no/low glucose avidity. If PET/CT is not available, for example, due to insurance denial, CT chest imaging will be used for GTV contouring which will only include solid component of the tumor. In either situation, normal tissue constraints will be met at higher priority than tumor coverage during hfRT planning. Normal tissue constraints per Tables S4,S5 will not be loosened up even with dose escalation pending phase I results. A 4D-CT image guided GTV delineation to take tumor motion into consideration is mandated with the creation of an internal target volume (ITV) around the GTV, accounting for tumor motion. The PTV will include the ITV with the addition of a 0.5 cm uniform margin.
A safety analysis using a two-step dose escalation scheme will be conducted for consolidative hfRT. Initial hfRT dose will be 6.5 Gy ×2 fractions, increasing to 10 Gy ×2 fractions if maximum tolerated dose (MTD) is not reached. Up to six subjects will be accrued to a given dose level to confirm that the dose level does not exceed MTD. The MTD will be defined as the dose below which 2 or more of 6 subjects experience dose-limiting toxicity (DLT).
DLT includes the following:
- Any Grade 3+ non-hematologic toxicity, excluding Grade 3 nausea/vomiting/diarrhea/electrolyte abnormality that is reduced to < Grade 3 with maximal supportive care within 3 days of onset.
- Any Grade 4 AE of any duration.
- Any Grade 3 AE that does not improve to baseline or Grade 1 within 7 days of onset.
- Any Grade 3 or 4 neutropenia associated with sepsis or fever >38 ℃.
- Any Grade 4+ hematologic toxicity. An exception is Grade 4 neutropenia that improves to < Grade 4 within ≤7 days of onset.
- Grade 3 thrombocytopenia associated with clinically significant bleeding.
After determining which dose regimen to use for consolidative hfRT after the initial 3+3 dose escalation study, we will continue to enroll subjects to a goal of 32 subjects for final data analyses including those treated at the same dose level during dose escalation. Tables S4-S6 describes dose constraints.
Three-dimensional coplanar or non-coplanar beam arrangements will be custom designed for each case to deliver highly conformal prescription dose distributions. Non-opposing, noncoplanar beams are preferable. Typically, ≥10 beams of radiation will be used with roughly equal weighting. In practice, more beams are used for larger lesion sizes. When static beams are used, a minimum of 7 non-opposing beams should be used. For arc rotation techniques, a minimum of 340 degrees (cumulative for all beams) should be utilized. In order to obtain acceptable coverage, field aperture size and shape should correspond nearly identically to the projection of the PTV along a beam’s eye view (i.e., no additional “margin” for dose buildup at the edges of the blocks or MLC jaws beyond the PTV). The only exception should be when observing the minimum field dimension of 3.5 cm when treating small lesions (see above). As such, prescription lines covering the PTV will typically be the 67–90% line (where the maximum dose is 100%); however, higher isodoses (hotspots) must be manipulated to occur within the target and not in adjacent normal tissue. The treatment isocenter or setup point in stereotactic coordinates will be determined from system fiducials (and can be adjusted pre-treatment depending on the results from localization imaging studies) and translated to the treatment record.
Localization and treatment delivery
Isocenter or reference point port localization images should be obtained on the treatment unit immediately before treatment to ensure proper alignment of the geometric center (i.e., isocenter) of the simulated fields (22,23). These IGRT images can be obtained with planar kV imaging devices, an in-room helical CT device, tomotherapy helical CT, cone-beam CT equipment, of standard electronic portal imaging device (EPID) imaging. In all cases, the RTOG Image Guidance Guidelines must be followed. For treatment systems that use kV imaging but also allow EPID imaging using the treatment beam, orthogonal images verifying the isocenter also should be obtained. Consolidative hfRT is scheduled in between the second and third cycle of durvalumab, to be delivered every other day (QOD) with exception that, if the first dose is on a Friday, the second dose is allowed to be delivered on the following Monday.
Follow-up and toxicity assessment
Clinical follow-up with physical exam and laboratory assessment should be performed at 1, 3, 6, 9, and 12 months. Tumor assessment via CT or MRI evaluation must be performed at least every 12 weeks ±1 week for the first 48 weeks [relative to the date of registration (day 0)], and thereafter until confirmed objective disease progression/death (whichever comes first).
For toxicities out of thorax and out of RT field, for the purpose of this study, we will define AEs as durvalumab-related. For AEs in tissues in thorax, i.e., chest wall/rib, esophagus, airway, great vessels and heart, or tissues adjacent to thorax such as liver and stomach, RT dose distributions in each organ will be reviewed from the sum plan combining RT plans of dCRT and hfRT. If TD5/5 dose constraint (the radiation dose that would result in 5% risk of severe complications within 5 years after irradiation based on QUANTEC, is exceeded, we will define the serious AEs (SAEs) as RT-related; otherwise as durvalumab-related (24).
Definition of end points
PFS (assessed per RECIST 1.1) will be defined as the time from the date of registration (day 0) until the date of objective disease progression or death (by any cause in the absence of progression) regardless of whether the subject withdraws from the trial or receives another anti-cancer therapy prior to progression (20). Subjects who have not progressed or died at the time of analysis will be censored at the time of the latest date of assessment from their last evaluable RECIST 1.1 assessment. OS is defined as the time from the date of registration (day 0) until death due to any cause. Any subject not known to have died at the time of analysis will be censored based on the last recorded date on which the subject was known to be alive. TTDM will be defined as the time from the date of registration until the first date of distant metastasis or death in the absence of distant metastasis. Distant metastasis is defined as any new lesion that is outside of the radiation field according to RECIST 1.1 or proven by biopsy (20). Subjects who have not developed distant metastasis or died at the time of analysis will be censored at the time of the latest date of assessment from their last evaluable RECIST 1.1 assessment.
Statistical analysis
Descriptive statistics will be used for all variables. Continuous variables will be summarized by the number of observations, mean, standard deviation, median, minimum, and maximum. Categorical variables will be summarized by frequency counts and percentages for each category. Unless otherwise stated, percentages will be calculated in relation to the entire correlated analysis set.
Time to event variables will be summarized using Kaplan-Meier curves; this includes OS, time to onset of symptoms, PFS and TTDM. Chi-square or Fisher’s exact test (as appropriate) will be used to compare treatment response to steroids for subjects who develop acute radiation pneumonitis (aRP) during or shortly after dCRT but prior to starting of durvalumab to those having aRP within six months after hfRT. Imaging features will be compared using two-sample or paired t-tests (or an appropriate non-parametric test) as appropriate to the hypothesis being tested. Unless otherwise stated, a significance level of 0.05 will be assumed for all tests.
We will evaluate the 12-month PFS data for the potential benefit of adding consolidative hfRT to be compared with the historical data but incorporating an interim analysis after recruiting thirteen subjects. In our case, the historical data from PACIFIC trial will be used (5,6). An additional 20% PFS increase is expected when adding hfRT to durvalumab, as such, a 1-year PFS of 75.9% or higher is predicted for this study. The sample size was estimated with the assistance from our statistician to achieve 80% statistical power.
Stopping rule
An interim analysis to be conducted at 6 months after enrollment of the 12th subject treated with the final selected dose of hfRT. The trial will be terminated with progression or death of 7 or more subjects.
The trial will be stopped if, at any time, a total of two cases developed Grade 5 toxicity after consolidative RT from side effects caused by immunotherapy and/or consolidative RT if other causes including tumor progression can be ruled out. With Grade 5 toxicities only occurred in 4.4% of patients in the durvalumab arm in PACIFIC trial, having an increased mortality rate to above 6%, if happened in this trial, would most likely offset any potential survival benefit adding consolidative RT rendering continuing the trial be meaningless as well as raise concerns of safety.
Ethical statement
The study will be conducted in accordance with the Declaration of Helsinki (as revised in 2013), as well as in accordance with the guidelines laid down by the International Conference on Harmonisation for Good Clinical Practice (ICH GCP E6 guidelines) (25,26). The study was approved by the Institutional Review Board and Scientific Review Committee of the University of Nebraska (No. 04-021). Patient accrual started in June 2021 and is currently ongoing. Informed consent was and will be obtained from all individual participants.
Discussion
The presented phase I/II trial evaluates the safety and efficacy of consolidative hfRT with durvalumab following dCRT for stage III NSCLC. The synergistic relationship between hfRT and durvalumab is hypothesized to improve PFS (primary endpoint), OS, DM, TTDM, LC, and LRC without compromising subject safety or QOL.
In the recently published results from PACIFIC trial, efficacy of durvalumab as consolidative therapy after definitive dCRT for stage III nonresectable NSCLC has been demonstrated (5,6). From May 2014 through April 2016, a total of 713 patients underwent randomization, of whom 709 (99.4%) received at least one dose of durvalumab or placebo after chemoradiotherapy. Randomization occurred 1 to 42 days after the patients had received chemoradiotherapy in a 2:1 ratio to receive durvalumab at a dose of 10 mg per kilogram (mg/kg) of body weight intravenously or matching placebo every 2 weeks as consolidation therapy for up to 12 months. The 48-month OS rate was 49.6% in the durvalumab group, compared to 36.3% in the placebo group (two-sided P<0.05) (6). Durvalumab significantly prolonged OS, as compared with placebo (stratified hazard ratio for death, 0.71; 95% CI: 0.57 to 0.88; P<0.05). The OS benefit with durvalumab was observed across all the pre-specified subgroups. PFS was also significantly longer with durvalumab than with placebo. The 48-month progression-free survival rate was 35.3% compared to 19.5% (stratified hazard ratio for disease progression or death, 0.55; 95% CI: 0.44 to 0.67, P<0.05). The overall response rate was 30.0% in the durvalumab group, as compared with 17.8% in the placebo group (P<0.001) (5).
Multiple studies have suggested the synergistic relationship between hfRT/SBRT and immunotherapy (9,11-15). Schaue et al. showed that, when using a mouse melanoma model, treatment with single fractions of 7.5 Gy or moderately higher doses was associated with an increase in anti-tumor CTLs and a decrease in regulatory T cells (Tregs), thus being immunostimulatory, which is not seen in 5 Gy ×1 regimen (14). Surprisingly, when the single fractional dose increased to 15 Gy, RT not only increased the infiltration of anti-tumor CTLs, but also the Tregs which could potentially dampen the immunostimulation. However, when mice were irradiated with 15 Gy total dose but fractionated in 2, 3 or 5 fractions, for example, 7.5 Gy ×2 fractions, 5 Gy ×3 fractions, or 3 Gy ×5 fractions, the 2-fraction regimen (7.5 Gy ×2 fractions), induced the highest level of anti-tumor CTLs and the lowest level of Tregs in the treated area, achieving the best tumor control. The authors hypothesized that the ratio of anti-tumor CTLs to Tregs might be a critical factor reflecting the aggregate effect of a particular RT regimen on the immunostimulatory and immunosuppressive pathways, with the most immunostimulating regimen being most likely ranging from 7.5 to 15 Gy per fraction.
When combining RT with immune checkpoint blockade such as using anti-CTLA-4 antibody in a mouse model, the most immunostimulating RT fractional dose falls into this range as well as reported by Demaria et al. (9). In their study using a mouse breast model, tumor cells were injected subcutaneously in mice at a primary site which was irradiated and a secondary site that was distal and outside the RT field. Mice were randomized to RT alone, anti-CTLA-4 antibody 9H10 alone, or a combination of RT and 9H10 antibody. Three different fractionation regimens were tested: 20 Gy ×1 fraction, 8 Gy ×3 fractions, or 6 Gy ×5 fractions; 8 Gy ×3 fractions in combination with anti-CTLA4 was significantly more effective than 6 Gy ×5 fractions, in inhibiting tumor growth at both the primary and secondary sites; 20 Gy ×1, although showing primary site tumor control, was ineffective in inducing an immune-mediated response at the secondary site. As for combining RT with programmed cell death 1 (PD-1)/PD-L1 antibody studies, Zeng et al. also confirmed the efficacy of a RT regimen of 12 Gy ×1 fraction, when combined with anti-PD-1 antibody, produces long-term survival in a mouse glioma model which was not seen in either therapy alone (27). This dose regimen induced increased infiltration by cytotoxic T cells and decreased Tregs within the tumor microenvironment of gliomas. The same regimen of 12 Gy ×1 fraction has also shown synergistic inhibitory effects with anti-PD-L1 therapy in mammary carcinoma and colon adenocarcinoma mouse models. However, further dose escalation per fraction to above 18 Gy/fraction was reported to induce negative feedback pathways including the expression of 3’ repair endonuclease 1 (TREX1) with further stimulation of DNA damage thus in turn degrading cytoplasmic DNA in micronuclei and preventing cGAS-STING activation, activating tumor-promoting macrophage and Tregs, etc., which may dampen immunostimulation as reviewed by Demaria et al. 2021 and Zhang et al. 2022 (28,29). These results indicate that the most immunostimulating RT dose range (7.5–15 Gy per fraction) shown by Schaue et al. is very likely shared among different types of primary tumors (14,15).
One of the main concerns of adding consolidative RT with potential immunostimulatory effects after definitive dCRT is pulmonary toxicity, particularly with anti-PD-L1 therapy. A prospective study has been conducted at University of Kentucky on thirty-seven patients with stage IIB/III NSCLC who underwent CT or PET/CT and were screened approximately 1 month after completion of definitive dCRT (19). Limited residual disease (≤5 cm) within the site of the primary tumor received a stereotactic radiation therapy boost of either 10 Gy ×2 fractions or 6.5 Gy ×3 fractions to the primary tumor, to achieve a total biologically equivalent dose (BED) >100 Gy. This study did not include anti-PD-L1 therapy. The long-term toxicities included five patients (13.5%) who experienced Grade 3 pneumonitis, four developed acutely within three months after SBRT and one late onset. This is comparable to historical “moderate to severe” radiation pneumonitis rates of 8.0% to 15.3% per the authors. No Grade 4 or 5 pneumonitis occurred. Predictors for Grade 3 pneumonitis included age and mean lung dose during definitive dCRT. Other studies have also been proposed to combine SBRT boost with dCRT for locally advanced NSCLC patients but mostly in pre-immunotherapy era (30).
The relative importance of LC increases as distant metastatic free survival improves with the use of immunotherapy. Ablative radiotherapy as consolidative treatment may provide a beneficial avenue for improving LC in stage III NSCLC. The Pacific trial suggests that a component of locoregional failure remained a common pattern of failure of patients who recurred (5). However, in the trial discussed above with SBRT boost after dCRT in stage IIB/III NSCLC, with a median follow-up of 25.2 months, the crude LC rate for the entire group was 78% with 29% regional failure and 65% distant failure (19). Interestingly, when comparing the outcome of boost dose regimens, although 6.5 Gy ×3 fractions had a slightly lower BED (102.2 Gy including the definitive conventional fractionated RT dose) than 10 Gy ×2 fractions (110 Gy), both achieved similar rates of LC (22% vs. 21%, P=1.0) (19). However, the survival for the 20 Gy boost (10 Gy ×2 fractions) were numerically better. Median OS was 19.2 months for the 19.5 Gy boost vs. 26.4 months for the 20 Gy boost (P=0.05). Although not statistically significantly different, the PFS curves of 19.5 and 20 Gy boost treatment started to separate after 6 months with 20 Gy boost showing much better long-term disease control. Although no conclusions can be drawn from these data with the small number of patients in each group and a nonrandomized design, a possible stronger immunostimulatory effect from 10 Gy ×2 fractions than 6.5 Gy ×3 fractions could partly explain the difference.
The combination of hfRT with immunotherapy after dCRT is understudied, although there are some small prospective trials evaluating the combination of SBRT with immunotherapy. The University of Chicago recently completed a phase I study of SBRT followed by pembrolizumab (anti-PD-L1) in patients with multiple sites of metastatic solid tumors (31). Of the total 151 sites of metastases irradiated, 68 were in the lung, 24 in the liver, 28 in other abdomen/pelvis sites, 16 in the bone, and 15 near the spine. The abscopal response rate per RECIST criteria of any single non-irradiated target metastasis was an impressive 26.9%. This off-target effects, or abscopal effects, generated by combined SBRT and immunotherapy. Grade 3 toxicity was seen in 6 patients (pneumonitis n=3; colitis n=2; hepatic toxicity n=1). These promising results in a heterogenous group of tumors support further studies combining checkpoint inhibitors with SBRT.
More specifically, the short-term safety of combining thoracic SBRT and immunotherapy was recently explored at MD Anderson Cancer Center in a combined analysis of two out of three prospective trials (32). The first was the phase 1–2 trial testing SBRT with ipilimumab (anti-CTLA4). The second was another ongoing phase 1–2 trial testing SBRT with concurrent pembrolizumab (anti-PD-L1) in metastatic NSCLC. With RT doses of 50 Gy in 4 fractions or 60 Gy in 10 fractions with a median follow up of 6.9 months (range, 0.5–30.9 months), there were no Grade ≥4 toxicities among the 60 enrolled patients. Only four patients experienced Grade 3 pulmonary toxicities with no difference between the ipilimumab and pembrolizumab groups. These short-term pulmonary toxicity rates were on par with RTOG 0236 which was a phase II study with SBRT only for stage I NSCLC, in which 8 of 55 patients developed Grade 3 respiratory events (23).
In summary, preclinical and limited clinical data suggest the most efficient immunostimulating effects of fractional dose of RT being around 8–12 Gy with combined SBRT with concurrent immunotherapy overall safe in practice. We thus hypothesize that combining consolidative hfRT regimen of 10 Gy ×2 fractions for boosting the residual primary lung cancer with adjuvant anti-PD-L1 therapy will be safe and could further improve immunostimulation and provide better tumor control locoregionally and distantly than either modality alone.
Acknowledgments
Funding: The study is funded by
Footnote
Peer Review File: Available at https://jtd.amegroups.com/article/view/10.21037/jtd-23-304/prf
Conflicts of Interest: All authors have completed the ICMJE uniform disclosure form (available at https://jtd.amegroups.com/article/view/10.21037/jtd-23-304/coif). C.Z. received grand funding from AstraZeneca, Inc., clinical trial research funding from BioMiMetix, and travel support from GTMedical Technologies. C.Z. reports a US Provisional patent (63/164,215) issued which is unrelated to the current study. A.K.G. reports Grants to his institution from Veterans Health Administration Office of Research and Development, Merck, TAB Biosciences, NEKTAR Therapeutics, Mirati Therapeutics, IOVANCE Therapeutics, Apexigen; Royalties from Oxford University Press; Consulting fees from AstraZeneca, Flagship Biosciences, G1 Therapeutics, Jazz Pharmaceuticals, Cardinal Health, Mirati Therapeutics, Beigene Ltd., Sanofi Genzyme, Blueprint Medicines, Regeneron Pharmaceuticals; Honoraria for lectures from MedLearning Group and Plexus Communications; DSMB for YmAbs Therapeutics; Leadership roles in Academic and Community Cancer Research United (ACCRU) and A Breath of Hope for Lung Cancer (ABOHLC); Receipt of drugs to the institution from Takeda Pharmaceuticals and Chimerx. C.W. reports funding from Chi Zhang’s grant (PI Astra Zeneca Pharmaceuticals IIT Grant; UNMC project number MEDI4736). The other authors have no conflicts of interest to declare.
Ethical Statement: The authors are accountable for all aspects of the work in ensuring that questions related to the accuracy or integrity of any part of the work are appropriately investigated and resolved. The study will be conducted in accordance with the Declaration of Helsinki (as revised in 2013), as well as in accordance with the guidelines laid down by the International Conference on Harmonisation for Good Clinical Practice (ICH GCP E6 guidelines). The study was approved by the Institutional Review Board and Scientific Review Committee of the University of Nebraska (No. 04-021). Patient accrual started in June 2021 and is currently ongoing. Informed consent was and will be obtained from all individual participants.
Open Access Statement: This is an Open Access article distributed in accordance with the Creative Commons Attribution-NonCommercial-NoDerivs 4.0 International License (CC BY-NC-ND 4.0), which permits the non-commercial replication and distribution of the article with the strict proviso that no changes or edits are made and the original work is properly cited (including links to both the formal publication through the relevant DOI and the license). See: https://creativecommons.org/licenses/by-nc-nd/4.0/.
References
- Bray F, Ferlay J, Soerjomataram I, et al. Global cancer statistics 2018: GLOBOCAN estimates of incidence and mortality worldwide for 36 cancers in 185 countries. CA Cancer J Clin 2018;68:394-424. [Crossref] [PubMed]
- Robinson LA, Ruckdeschel JC, Wagner H Jr, et al. Treatment of non-small cell lung cancer-stage IIIA: ACCP evidence-based clinical practice guidelines (2nd edition). Chest 2007;132:243S-65S.
- Eberhardt WE, Pöttgen C, Gauler TC, et al. Phase III Study of Surgery Versus Definitive Concurrent Chemoradiotherapy Boost in Patients With Resectable Stage IIIA(N2) and Selected IIIB Non-Small-Cell Lung Cancer After Induction Chemotherapy and Concurrent Chemoradiotherapy (ESPATUE). J Clin Oncol 2015;33:4194-201. [Crossref] [PubMed]
- Aupérin A, Le Péchoux C, Rolland E, et al. Meta-analysis of concomitant versus sequential radiochemotherapy in locally advanced non-small-cell lung cancer. J Clin Oncol 2010;28:2181-90. [Crossref] [PubMed]
- Antonia SJ, Villegas A, Daniel D, et al. Durvalumab after Chemoradiotherapy in Stage III Non-Small-Cell Lung Cancer. N Engl J Med 2017;377:1919-29. [Crossref] [PubMed]
- Faivre-Finn C, Vicente D, Kurata T, et al. Four-Year Survival With Durvalumab After Chemoradiotherapy in Stage III NSCLC-an Update From the PACIFIC Trial. J Thorac Oncol 2021;16:860-7. [Crossref] [PubMed]
- MOLE RH. Whole body irradiation; radiobiology or medicine? Br J Radiol 1953;26:234-41. [Crossref] [PubMed]
- Abuodeh Y, Venkat P, Kim S. Systematic review of case reports on the abscopal effect. Curr Probl Cancer 2016;40:25-37. [Crossref] [PubMed]
- Demaria S, Formenti SC. Radiation as an immunological adjuvant: current evidence on dose and fractionation. Front Oncol 2012;2:153. [Crossref] [PubMed]
- Golden EB, Formenti SC. Is tumor (R)ejection by the immune system the "5th R" of radiobiology? Oncoimmunology 2014;3:e28133. [Crossref] [PubMed]
- Gandhi SJ, Minn AJ, Vonderheide RH, et al. Awakening the immune system with radiation: Optimal dose and fractionation. Cancer Lett 2015;368:185-90. [Crossref] [PubMed]
- Monjazeb AM, Schoenfeld JD. Radiation dose and checkpoint blockade immunotherapy: unanswered questions. Lancet Oncol 2016;17:e3-4. [Crossref] [PubMed]
- Dewan MZ, Galloway AE, Kawashima N, et al. Fractionated but not single-dose radiotherapy induces an immune-mediated abscopal effect when combined with anti-CTLA-4 antibody. Clin Cancer Res 2009;15:5379-88. [Crossref] [PubMed]
- Schaue D, Ratikan JA, Iwamoto KS, et al. Maximizing tumor immunity with fractionated radiation. Int J Radiat Oncol Biol Phys 2012;83:1306-10. [Crossref] [PubMed]
- Deng L, Liang H, Burnette B, et al. Irradiation and anti-PD-L1 treatment synergistically promote antitumor immunity in mice. J Clin Invest 2014;124:687-95. [Crossref] [PubMed]
- Dunn GP, Old LJ, Schreiber RD. The three Es of cancer immunoediting. Annu Rev Immunol 2004;22:329-60. [Crossref] [PubMed]
- Hegde AM, Cherry RC, Stroud CRG, et al. Outcomes of immunomodulatory radiation strategies in combination with nivolumab compared with single agent nivolumab in lung cancer patients. J Clin Oncol 2018;36:e21134.
- Park B, Yee C, Lee KM. The effect of radiation on the immune response to cancers. Int J Mol Sci 2014;15:927-43. [Crossref] [PubMed]
- Kumar S, Feddock J, Li X, et al. Update of a Prospective Study of Stereotactic Body Radiation Therapy for Post-Chemoradiation Residual Disease in Stage II/III Non-Small Cell Lung Cancer. Int J Radiat Oncol Biol Phys 2017;99:652-9. [Crossref] [PubMed]
- Eisenhauer EA, Therasse P, Bogaerts J, et al. New response evaluation criteria in solid tumours: revised RECIST guideline (version 1.1). Eur J Cancer 2009;45:228-47. [Crossref] [PubMed]
- Lim W, Ridge CA, Nicholson AG, et al. The 8(th) lung cancer TNM classification and clinical staging system: review of the changes and clinical implications. Quant Imaging Med Surg 2018;8:709-18. [Crossref] [PubMed]
- Potters L, Steinberg M, Rose C, et al. American Society for Therapeutic Radiology and Oncology and American College of Radiology practice guideline for the performance of stereotactic body radiation therapy. Int J Radiat Oncol Biol Phys 2004;60:1026-32. [Crossref] [PubMed]
- Timmerman R, Paulus R, Galvin J, et al. Stereotactic body radiation therapy for inoperable early stage lung cancer. JAMA 2010;303:1070-6. [Crossref] [PubMed]
- Marks LB, Ten Haken RK, Martel MK. Guest editor's introduction to QUANTEC: a users guide. Int J Radiat Oncol Biol Phys 2010;76:S1-S2. [Crossref] [PubMed]
- World Medical Association Declaration of Helsinki. ethical principles for medical research involving human subjects. J Am Coll Dent 2014;81:14-8.
- Dixon JR Jr. The International Conference on Harmonization Good Clinical Practice guideline. Qual Assur 1998;6:65-74. [Crossref] [PubMed]
- Zeng J, See AP, Phallen J, et al. Anti-PD-1 blockade and stereotactic radiation produce long-term survival in mice with intracranial gliomas. Int J Radiat Oncol Biol Phys 2013;86:343-9. [Crossref] [PubMed]
- Demaria S, Guha C, Schoenfeld J, et al. Radiation dose and fraction in immunotherapy: one-size regimen does not fit all settings, so how does one choose? J Immunother Cancer 2021;9:e002038. [Crossref] [PubMed]
- Zhang Z, Liu X, Chen D, et al. Radiotherapy combined with immunotherapy: the dawn of cancer treatment. Signal Transduct Target Ther 2022;7:258. [Crossref] [PubMed]
- Alcibar OL, Nadal E, Romero Palomar I, et al. Systematic review of stereotactic body radiotherapy in stage III non-small cell lung cancer. Transl Lung Cancer Res 2021;10:529-38. [Crossref] [PubMed]
- Luke JJ, Lemons JM, Karrison TG, et al. Safety and Clinical Activity of Pembrolizumab and Multisite Stereotactic Body Radiotherapy in Patients With Advanced Solid Tumors. J Clin Oncol 2018;36:1611-8. [Crossref] [PubMed]
- Verma V, Cushman TR, Selek U, et al. Safety of Combined Immunotherapy and Thoracic Radiation Therapy: Analysis of 3 Single-Institutional Phase I/II Trials. Int J Radiat Oncol Biol Phys 2018;101:1141-8. [Crossref] [PubMed]