Negative impact of ratio of the microvascular area to tumor area on the response to EGFR-TKI in non-small cell lung cancer with an EGFR mutation
Highlight box
Key findings
• High ratio of microvascular area to tumor area (RMV) in non-small cell lung cancer (NSCLC) harboring epidermal growth factor receptor (EGFR) mutations is a negative predictive factor for EGFR-tyrosine kinase inhibitor (EGFR-TKI), suggesting that the tumor microenvironment with abundant microvessels in EGFR mutation-positive NSCLC is associated with resistance to EGFR-TKI.
What is known and what is new?
• Tumor angiogenesis plays a crucial role in cancer progression and distant metastasis. In EGFR mutation-positive adenocarcinoma, a tumor microenvironment with abundant microvessels was associated with aggressive behavior.
• This study clarifies the impact of RMV on the efficacy of EGFR-TKIs in patients with NSCLCs with EGFR mutations.
What is the implication, and what should change now?
• The combination of EGFR-TKIs and angiogenesis inhibitors may be an appropriate treatment strategy.
Introduction
Background
Tumor angiogenesis plays a crucial role in cancer progression and distant metastasis. Compared to normal vasculature, tumor vasculature resulting from angiogenesis has abnormal structural and functional features, such as tortuosity, leakage, ramification, and poor pericyte support (1). These blood vessels characterized by high permeability lead to interstitial hypertension, which decreases drug delivery into the tumor and is likely to lead to resistance to treatment (2).
Rationale and knowledge gap
Microvascular density (MVD) based on pathological methods is generally recognized as an indicator of tumor angiogenesis in various cancers, including lung cancer. MVD is calculated as the ratio of microvessels number per area of cancer tissue and has been reported to be a prognostic factor in lung and other types of cancers (3-7). However, assessment of MVD, in which observers count the number of microvessels via immunohistochemistry staining of vascular endothelial cells, involves the subjectivity of observers, such as in determining the size and morphology of microvessels (8). Development of quantitative evaluation methods based on image analysis software has enabled automatic analysis of the microvascular area. These analyses are expected to allow for more objective and reproducible measurements for microvascular evaluations.
Epidermal growth factor receptor (EGFR) mutations are the most common driver mutations in non-small cell lung cancer (NSCLC) and are detected in approximately 40% of lung adenocarcinomas in Asian populations (9). EGFR-tyrosine kinase inhibitors (EGFR-TKIs) for patients with NSCLC with EGFR mutations provided therapeutic benefits compared to cytotoxic anticancer agents in several randomized phase III studies and are used as the standard treatment in the first-line setting (10-13). In EGFR mutation-positive adenocarcinoma, a tumor microenvironment with abundant microvessels was reported to be associated with aggressive behavior (14); however, the clinical impact of tumor microvessels on the efficacy of EGFR-TKIs in EGFR mutation-positive NSCLC is unclear.
Objective
In this study, we measured tumor microvessel areas (MVAs) using image analysis software and calculated the ratio of microvascular area to tumor area (RMV), which is the microvessel areas divided by the field view area. Additionally, we aimed to clarify the impact of RMV on the efficacy of EGFR-TKIs in patients with NSCLC with EGFR mutations. We present this article in accordance with the REMARK reporting checklist (available at https://jtd.amegroups.com/article/view/10.21037/jtd-23-1723/rc).
Methods
Study design and patients
This study included 40 consecutive post-operative recurrent NSCLC patients with EGFR mutations who were treated with EGFR-TKIs as first-line treatment at Kumamoto University Hospital from 1 January 2010 to 28 February 2021. We retrospectively collected the following data from the medical records: age, sex, smoking history, Eastern Cooperative Oncology Group (ECOG) performance status (PS), histology, pathological stage, EGFR mutation status, and the type of EGFR-TKI (gefitinib, erlotinib, afatinib, or osimertinib). EGFR mutations were examined using the Cobas® EGFR mutation test v2 (Roche Molecular Systems Inc., Pleasanton, CA, USA) or Oncomine Dx Target Test (Thermo Fisher, Waltham, MA, USA).
The study was conducted in accordance with the Declaration of Helsinki (as revised in 2013). The study was approved by the institutional ethics review board of Kumamoto University Hospital (IRB No. 2364) and individual consent for this retrospective analysis was waived.
Immunohistochemical analysis
Sections of formalin-fixed, paraffin-embedded tissues were depleted of paraffin and hydrated. They were incubated with 3% H2O2 for 5 min, washed with 0.05% Tween in Tris-buffered saline (TBST), exposed to blocking buffer [5% goat serum and 0.5% bovine serum albumin in phosphate-buffered saline (PBS)] in PBS for 10 min at 24 degrees Celsius, and incubated with mouse anti-CD34 antibodies (ab8536; clone QBEND-10; Abcam, Cambridge, UK) for 60 min. They were then washed with TBST, incubated with biotinylated secondary antibodies (Vector Laboratories, CA, USA) for 10 min at room temperature, and washed again, after which immune complexes were detected using an avidin-peroxidase complex (Vector Laboratories) and 3,3'-diaminobenzidine (Vector Laboratories). The sections were counterstained with Mayer’s hematoxylin, dehydrated using a graded series of ethanol, and mounted in Marinol (Muto Pure Chemicals, Tokyo, Japan).
Assessment of microvessels
Three images with the highest density of CD34-positive microvessels as the hotspot field of the tumor section were captured using a BX51 microscope (Olympus, Tokyo, Japan) at ×200 magnification. The number of CD34-positive microvessels was counted by two independent investigators (M.A. and K.S.). MVD was recorded as the average count of CD34-positive microvessels in three images. The MVAs in each image were automatically measured using StrataQuest (TissueGnostics, Vienna, Austria). The RMV in each image was calculated as the area occupied by CD34-positive microvessels divided by the total area of the field of view (area of 0.175 mm2 per field of view). The RMV in each case was recorded as the average RMV of three images. Representative images of the RMV measurements are shown in Figure 1.
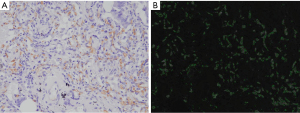
Clinical outcome parameter
Progression-free survival (PFS) was calculated from the initial date of EGFR-TKI therapy to the date of progressive disease or last follow-up. Overall survival (OS) was calculated from the initial date of EGFR-TKI therapy to the date of death or last follow-up. The best tumor response was evaluated based on the Response Evaluation Criteria in Solid Tumours guidelines version 1.1.
Statistical analysis
Primary endpoint of this study was to assess the correlation between MVD and RMV, to compare PFS and OS according to RMV, and to analyze whether RMV was independent predictive factors of PFS and OS. Chi-square test, Fisher’s exact test, or Mann-Whitney U test was used to investigate the association of clinical factors. The Spearman’s rank correlation coefficient was used to assess the correlation between MVD and RMV. Survival curves were generated using the Kaplan-Meier method and compared using the log-rank test. Univariate and multivariate analyses were performed using a Cox proportional hazard model to identify independent predictive factors of PFS and OS. Age, sex, smoking, PS, pathological stage, EGFR mutation type, and RMV were included in the multivariable model as covariates. All statistical analyses were performed using SPSS (version 23.0; IBM, Armonk, NY, USA). Statistical significance was set at P<0.05.
Results
Patient characteristics
The median age at diagnosis was 71.5 years. Of the patients, 22 (55%) were male, 36 (90%) had ECOG PS 0–1, 38 (95%) had adenocarcinoma, 19 (47.5%) had pathological stage I, 23 (57.5%) had vascular invasion, and 19 (47.5%) had pleural invasion (Table 1). Regarding the type of EGFR mutation, 24 patients (60%) had exon 19 deletions, and 15 patients (37.5%) had exon 21 L858R mutation. The EGFR-TKI drugs administered as first-line treatment for post-operative recurrence were as follows: gefitinib in 11 (27.5%), erlotinib in 7 (17.5%), afatinib in 11 (27.5%), and osimertinib in 11 (27.5%) patients.
Table 1
Characteristics | Subtypes | Value (n=40) |
---|---|---|
Age, years, median [range] | 71.5 [43–89] | |
Sex | Male | 22 (55.0) |
Female | 18 (45.0) | |
Smoking | Yes | 17 (42.5) |
No | 23 (57.5) | |
ECOG PS | 0 | 20 (50.0) |
1 | 16 (40.0) | |
2 | 3 (7.5) | |
3 | 0 | |
4 | 1 (2.5) | |
Histology | Ad | 38 (95.0) |
Non-Ad | 2 (5.0) | |
EGFR mutation type | Exon 19 deletions | 24 (60.0) |
Exon 21 mutations | ||
L858R | 15 (37.5) | |
L861Q | 1 (2.5) | |
Vascular invasion | Absence | 17 (42.5) |
Presence | 23 (57.5) | |
Pleural invasion | Absence | 21 (52.5) |
Presence | 19 (47.5) | |
Pathological stage | I | 19 (47.5) |
II | 12 (30.0) | |
III | 9 (22.5) | |
EGFR-TKI | Gefitinib | 11 (27.5) |
Erlotinib | 7 (17.5) | |
Afatinib | 11 (27.5) | |
Osimertinib | 11 (27.5) |
Data are presented as n (%) unless otherwise specified. ECOG PS, Eastern Cooperative Oncology Group performance status; Ad, adenocarcinoma; EGFR, epidermal growth factor receptor mutation; EGFR-TKI, epidermal growth factor receptor-tyrosine kinase inhibitor.
Assessment of correlation between MVD and RMV
The median MVD and RMV were 123/mm2 (range, 70–470/mm2) and 0.058 (range, 0.013–0.094), respectively. Scatter plots of the MVD and RMV data are shown in Figure 2. A significant moderate positive correlation was observed between MVD and RMV (r=0.47, P=0.020).
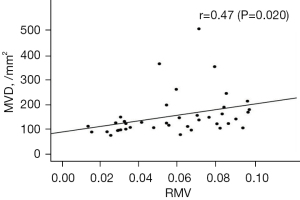
Comparison of clinicopathological features according to RMV
Using the median RMV as the cut-off value, we divided the patients into high (≥0.058) and low (<0.058) RMV groups. No significant difference in clinicopathological factors was observed between the two groups (Table 2).
Table 2
Variables | Subtypes | RMV | P | |
---|---|---|---|---|
High (N=20) | Low (N=20) | |||
Age, years, median [range] | 72.5 [46–89] | 71 [43–85] | 0.659 | |
Sex | Male | 13 [65] | 9 [45] | 0.341 |
Female | 7 [35] | 11 [55] | ||
Smoking | Yes | 11 [55] | 6 [30] | 0.2 |
No | 9 [45] | 14 [70] | ||
ECOG PS | 0–1 | 17 [85] | 20 [100] | 0.231 |
2–4 | 3 [15] | 0 [0] | ||
Histology | Ad | 19 [95] | 19 [95] | >0.99 |
Non-Ad | 1 [5] | 1 [5] | ||
Vascular invasion | Absence | 8 [40] | 15 [75] | 0.054 |
Presence | 12 [60] | 5 [25] | ||
Pleural invasion | Absence | 14 [70] | 7 [35] | 0.056 |
Presence | 6 [30] | 13 [65] | ||
Pathological stage | I–II | 16 [80] | 15 [75] | >0.99 |
III | 4 [20] | 5 [25] | ||
EGFR mutation type | Exon 19 deletions | 9 [45] | 15 [75] | 0.105 |
Exon 21 mutations | 11 [55] | 5 [25] | ||
EGFR-TKI | Gefitinib | 5 [25] | 6 [30] | 0.6 |
Erlotinib | 4 [20] | 3 [15] | ||
Afatinib | 4 [20] | 7 [35] | ||
Osimertinib | 7 [35] | 4 [20] |
Data are presented as n [%] unless otherwise specified. RMV, ratio of microvascular area to tumor area; ECOG PS, Eastern Cooperative Oncology Group performance status; Ad, adenocarcinoma; EGFR, epidermal growth factor receptor mutation; EGFR-TKI, epidermal growth factor receptor-tyrosine kinase inhibitor.
Tumor response, PFS, and OS analyses with EGFR-TKI according to RMV
Among the 30 patients with measurable lesions, four had complete response, seventeen had partial response, five had stable disease, one had progressive disease, and three were not evaluable (Table S1). The best objective response rate was 70% [95% confidence interval (95% CI): 52.0–83.5%], and no significant difference was observed between the high and low RMV groups.
The median follow-up time from the initiation of EGFR-TKI therapy in the first-line setting was 1,059.5 days (range, 120–2,807 days). The median PFS and OS were 610 days (range, 102–2,807 days) and 1,602 days (range, 146–2,933 days), respectively. The median PFS was significantly shorter in the high RMV group than in the low RMV group [296 days (95% CI: 217–374) vs. 918 days (95% CI: 279–1,556), P=0.002; Figure 3A]. However, no significant differences in OS were observed between the high and low RMV groups [1,586 days (95% CI: 422–2,749) vs. 1,839 days (95% CI: 1,361–2,316), P=0.355; Figure 3B].
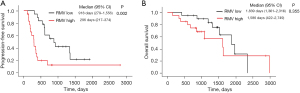
Univariate and multivariate analysis for PFS and OS
The univariate and multivariate analyses of PFS and OS are summarized in Tables 3,4. Univariate analysis showed that PFS was significantly associated with RMV [hazard ratio (HR): 3.25, 95% CI: 1.48–7.16, P=0.003]. Multivariate analysis revealed that only high RMV (HR: 3.21, 95% CI: 1.18–8.76, P=0.022) were significant independent negative predictive factors for PFS after first-line EGFR-TKI treatment.
Table 3
Variables | Subtypes | Univariate | Multivariate | |||
---|---|---|---|---|---|---|
HR (95% CI) | P | HR (95% CI) | P | |||
Age | <75 years | Ref | 0.630 | Ref | 0.830 | |
≥75 years | 0.82 (0.36–1.87) | 1.12 (0.40–3.16) | ||||
Sex | Male | Ref | 0.371 | Ref | 0.050 | |
Female | 1.42 (0.66–3.04) | 3.77 (1.00–14.23) | ||||
Smoking | No | Ref | 0.405 | Ref | 0.225 | |
Yes | 0.72 (0.33–1.56) | 0.39 (0.09–1.78) | ||||
PS | 0–1 | Ref | 0.963 | Ref | 0.633 | |
≥2 | 1.05 (0.14–7.82) | 0.58 (0.06–5.337) | ||||
Stage | I–II | Ref | 0.367 | Ref | 0.810 | |
III | 0.61 (0.21–1.78) | 0.50 (0.23–1.09) | ||||
EGFR mut | Exon 19 deletions | Ref | 0.370 | Ref | 0.851 | |
Exon 21 mutations | 1.43 (0.66–3.10) | 0.913 (0.35–2.36) | ||||
RMV | Low | Ref | 0.003 | Ref | 0.022 | |
High | 3.25 (1.48–7.16) | 3.21 (1.18–8.76) |
PFS, progression-free survival; HR, hazard ratio; CI, confidence interval; PS, performance status; EGFR, epidermal growth factor receptor; mut, mutation; RMV, ratio of microvascular area to tumor area.
Table 4
Variables | Subtypes | Univariate | Multivariate | |||
---|---|---|---|---|---|---|
HR (95% CI) | P | HR (95% CI) | P | |||
Age | <75 years | Ref | 0.969 | Ref | 0.926 | |
≥75 years | 0.98 (0.36–2.67) | 1.07 (0.27–4.24) | ||||
Sex | Male | Ref | 0.271 | Ref | 0.161 | |
Female | 1.78 (0.63–5.00) | 3.11 (0.64–15.12) | ||||
Smoking | No | Ref | 0.998 | Ref | 0.602 | |
Yes | 1.00 (0.38–2.67) | 0.65 (0.13–3.29) | ||||
PS | 0–1 | Ref | 0.009 | Ref | 0.027 | |
≥2 | 2.93 (1.03–6.60) | 12.59 (1.34–118.30) | ||||
Stage | I–II | Ref | 0.801 | Ref | 0.687 | |
III | 1.16 (0.37–3.60) | 0.74 (0.17–3.20) | ||||
EGFR mut | Exon 19 deletions | Ref | 0.761 | Ref | 0.331 | |
Exon 21 mutations | 0.85 (0.30–2.44) | 0.53 (0.14–1.92) | ||||
RMV | Low | Ref | 0.359 | Ref | 0.697 | |
High | 1.58 (0.60–4.16) | 1.33 (0.32–5.47) |
OS, overall survival; HR, hazard ratio; CI, confidence interval; PS, performance status; EGFR, epidermal growth factor receptor; mut, mutation; RMV, ratio of microvascular area to tumor area.
Univariate analysis showed that OS was significantly associated with ECOG PS (HR: 2.93, 95% CI: 1.03–6.60, P=0.009), but no significant differences were found in RMV (HR: 1.58; 95% CI: 0.60–4.16, P=0.359). Multivariate analysis revealed that ECOG PS ≥2 (HR: 12.59, 95% CI: 1.34–118.30, P=0.027) was a significant independent negative predictive factor for OS.
Discussion
To our knowledge, this is the first study to assess the association between RMV and antitumor efficacy of EGFR-TKIs in patients with NSCLC harboring EGFR mutations. This study showed a significant positive correlation between MVD and RMV, suggesting that RMV and MVD might be indicators of angiogenesis. Moreover, we found that a high RMV in patients with EGFR mutation-positive NSCLC was a negative predictive factor for PFS in EGFR-TKI treatment; thus, a novel treatment strategy with EGFR-TKIs, such as a combination of EGFR-TKI and angiogenesis inhibitors, might be necessary for EGFR mutation-positive NSCLC with abundant tumor microvessels.
Numerous studies using MVD based on immunohistochemistry have shown that MVD is correlated with lung cancer development and prognosis (3,15,16). In contrast, MVA has been reported to be a prognostic factor in breast cancer and renal cell carcinoma (17-19). Moreover, Sullivan et al. described that the evaluation of MVA by automated image analysis was correlated with pathologist-diagnosed MVD (17), indicating that MVA is an alternative angiogenetic marker of MVD. However, MVA is measured as the total area of blood vessels per field of view and is dependent on magnification of the microscope and whole tumor size, which makes it difficult to consistently evaluate the overall assessment of angiogenesis status in whole cancer tissue. In the current study, RMV was defined as the ratio of microvascular area to field of view, likely to be estimated independently of tumor size, and was found to be positively correlated with MVD. Thus, the measurement of RMV using image analysis software could be an alternative indicator of tumor angiogenesis as well as MVD.
We found that the PFS of EGFR-TKI in the higher RMV group was significantly shorter than that in the lower RMV group. Tumor angiogenesis assessed by MVD has been reported to be associated with resistance to cytotoxic chemotherapy in several cancers, including breast, cervical, and ovarian cancers (20,21). One cause of drug resistance is the vascular endothelial growth factor (VEGF). VEGF plays an important role in tumor angiogenesis and leads to leakage from microvessels in tumor tissues and elevation of interstitial fluid pressure, which decreases drug delivery and results in drug resistance (22-25). Previous reports have shown MVD to be significantly correlated with plasma and tumor VEGF levels in NSCLC and esophageal and gastric cancers (26,27). Thus, EGFR mutation-positive NSCLC with higher RMV is suggested to be a microenvironment with abundant VEGF, which might reduce the efficacy of EGFR-TKIs due to inhibition of drug delivery.
Angiogenesis inhibitors, such as bevacizumab and ramucirumab, have demonstrated clinical efficacy and have been used for patients with NSCLC in routine practice. Recently, randomized phase III studies comparing the combination therapy of EGFR-TKI and angiogenesis inhibitors to EGFR-TKI monotherapy in EGFR mutation-positive NSCLC showed that the former resulted in longer PFS (28,29). In ovarian and colorectal cancers, high MVD assessed by immunohistochemistry has been reported to be a potential predictive factor of bevacizumab (30,31). Furthermore, Zhao et al. reported a positive correlation between MVD and tumor shrinkage rate in patients with NSCLC treated with platinum doublets and bevacizumab (4). We found that EGFR mutation-positive NSCLC with a high RMV was resistant to EGFR-TKI monotherapy compared to that with a low RMV. Based on these findings, EGFR mutation-positive NSCLC with abundant tumor microvessels might be preferable in combination with EGFR-TKI and angiogenesis inhibitors.
Despite these findings, our study had several limitations. First, this was a retrospective study performed in a single institution; therefore, this study included small number of Japanese patients and various selection biases might be existed. Second, this study used surgical specimens from patients with post-operative recurrence. The tumor microenvironment, including angiogenesis status, might have changed between the surgical and post-operative recurrent specimens, and these differences in microvasculature might have affected the results of our study. Third, four types of EGFR-TKIs (gefitinib, erlotinib, afatinib and osimertinib) were included in this study. In the LUX-lung 7 and FLAURA trials, afatinib and osimertinib significantly prolonged PFS compared to the first-generation EGFR-TKIs (gefitinib and erlotinib) in EGFR-mutant NSCLC (13,32). Although there was no significant difference in the type of EGFR-TKI between low and high RMV groups, the type of EGFR-TKI might have affected the results of this study. Fourth, image analysis software of RMV evaluation we employed was only StrataQuest in this study although previous studies on RMV have been used various image analysis software to evaluate RMV. It is necessary to verify the reliability and validity for RMV evaluation of each software. Fifth, MVD and RMV were previously reported as a negative prognostic marker for NSCLC (3,33). Although high RMV was significantly associated with shorter PFS of EGFR-TKI for EGFR-mutant NSCLC in this study, further study involving large cohort is needed to determine whether RMV is truly predictive factor of EGFR-TKI in patients with EGFR mutation-positive NSCLC. Sixth, median value of RMV that we provisionally employed was cut off level in order to analyze the predictive factor of EGFR-TKI. Therefore, a large prospective study using advanced and post-operative recurrent NSCLC specimens is required to clarify the optimal cut-off value of RMV for the predictive value of response to EGFR-TKIs.
Conclusions
High RMV in NSCLC harboring EGFR mutations is a negative predictive factor for EGFR-TKI, suggesting that the tumor microenvironment with abundant microvessels in EGFR mutation-positive NSCLC is associated with resistance to EGFR-TKI. In these populations, the combination of EGFR-TKIs and angiogenesis inhibitors may be an appropriate treatment strategy.
Acknowledgments
We are grateful to Ms. Takahashi, who is a technical assistant at the Department of Respiratory Medicine at Kumamoto University Hospital for her support.
Funding: This work was supported by
Footnote
Reporting Checklist: The authors have completed the REMARK reporting checklist. Available at https://jtd.amegroups.com/article/view/10.21037/jtd-23-1723/rc
Data Sharing Statement: Available at https://jtd.amegroups.com/article/view/10.21037/jtd-23-1723/dss
Peer Review File: Available at https://jtd.amegroups.com/article/view/10.21037/jtd-23-1723/prf
Conflicts of Interest: All authors have completed the ICMJE uniform disclosure form (available at https://jtd.amegroups.com/article/view/10.21037/jtd-23-1723/coif). The authors have no conflicts of interest to declare.
Ethical Statement: The authors are accountable for all aspects of the work in ensuring that questions related to the accuracy or integrity of any part of the work are appropriately investigated and resolved. The study was conducted in accordance with the Declaration of Helsinki (as revised in 2013). The study was approved by the institutional ethics review board of Kumamoto University Hospital (IRB No. 2364) and individual consent for this retrospective analysis was waived.
Open Access Statement: This is an Open Access article distributed in accordance with the Creative Commons Attribution-NonCommercial-NoDerivs 4.0 International License (CC BY-NC-ND 4.0), which permits the non-commercial replication and distribution of the article with the strict proviso that no changes or edits are made and the original work is properly cited (including links to both the formal publication through the relevant DOI and the license). See: https://creativecommons.org/licenses/by-nc-nd/4.0/.
References
- Carmeliet P, Jain RK. Angiogenesis in cancer and other diseases. Nature 2000;407:249-57. [Crossref] [PubMed]
- Heldin CH, Rubin K, Pietras K, et al. High interstitial fluid pressure - an obstacle in cancer therapy. Nat Rev Cancer 2004;4:806-13. [Crossref] [PubMed]
- Zhang T, Nie J, Liu X, et al. Correlation Analysis Among the Level of IL-35, Microvessel Density, Lymphatic Vessel Density, and Prognosis in Non-Small Cell Lung Cancer. Clin Transl Sci 2021;14:389-94. [Crossref] [PubMed]
- Zhao YY, Xue C, Jiang W, et al. Predictive value of intratumoral microvascular density in patients with advanced non-small cell lung cancer receiving chemotherapy plus bevacizumab. J Thorac Oncol 2012;7:71-5. [Crossref] [PubMed]
- Li Y, Wei X, Zhang S, et al. Prognosis of invasive breast cancer after adjuvant therapy evaluated with VEGF microvessel density and microvascular imaging. Tumour Biol 2015;36:8755-60. [Crossref] [PubMed]
- Balac I, Jurisic V, Laban A, et al. The CD34-microvascular density in colorectal cancer patients. J BUON 2012;17:97-101.
- Chen B, Fang WK, Wu ZY, et al. The prognostic implications of microvascular density and lymphatic vessel density in esophageal squamous cell carcinoma: Comparative analysis between the traditional whole sections and the tissue microarray. Acta Histochem 2014;116:646-53. [Crossref] [PubMed]
- Vermeulen PB, Libura M, Libura J, et al. Influence of investigator experience and microscopic field size on microvessel density in node-negative breast carcinoma. Breast Cancer Res Treat 1997;42:165-72. [Crossref] [PubMed]
- Yatabe Y, Kerr KM, Utomo A, et al. EGFR mutation testing practices within the Asia Pacific region: results of a multicenter diagnostic survey. J Thorac Oncol 2015;10:438-45. [Crossref] [PubMed]
- Mok TS, Wu YL, Thongprasert S, et al. Gefitinib or carboplatin-paclitaxel in pulmonary adenocarcinoma. N Engl J Med 2009;361:947-57. [Crossref] [PubMed]
- Mitsudomi T, Morita S, Yatabe Y, et al. Gefitinib versus cisplatin plus docetaxel in patients with non-small-cell lung cancer harbouring mutations of the epidermal growth factor receptor (WJTOG3405): an open label, randomised phase 3 trial. Lancet Oncol 2010;11:121-8. [Crossref] [PubMed]
- Wu YL, Zhou C, Hu CP, et al. Afatinib versus cisplatin plus gemcitabine for first-line treatment of Asian patients with advanced non-small-cell lung cancer harbouring EGFR mutations (LUX-Lung 6): an open-label, randomised phase 3 trial. Lancet Oncol 2014;15:213-22. [Crossref] [PubMed]
- Soria JC, Ohe Y, Vansteenkiste J, et al. Osimertinib in Untreated EGFR-Mutated Advanced Non-Small-Cell Lung Cancer. N Engl J Med 2018;378:113-25. [Crossref] [PubMed]
- Saruwatari K, Ikemura S, Sekihara K, et al. Aggressive tumor microenvironment of solid predominant lung adenocarcinoma subtype harboring with epidermal growth factor receptor mutations. Lung Cancer 2016;91:7-14. [Crossref] [PubMed]
- Bing Z, Jian-ru Y, Yao-quan J, et al. Evaluation of angiogenesis in non-small cell lung carcinoma by CD34 immunohistochemistry. Cell Biochem Biophys 2014;70:327-31. [Crossref] [PubMed]
- Meert AP, Paesmans M, Martin B, et al. The role of microvessel density on the survival of patients with lung cancer: a systematic review of the literature with meta-analysis. Br J Cancer 2002;87:694-701. [Crossref] [PubMed]
- Sullivan CA, Ghosh S, Ocal IT, et al. Microvessel area using automated image analysis is reproducible and is associated with prognosis in breast cancer. Hum Pathol 2009;40:156-65. [Crossref] [PubMed]
- Sato M, Nakai Y, Nakata W, et al. Microvessel area of immature vessels is a prognostic factor in renal cell carcinoma. Int J Urol 2014;21:130-4. [Crossref] [PubMed]
- Sharma SG, Aggarwal N, Gupta SD, et al. Angiogenesis in renal cell carcinoma: correlation of microvessel density and microvessel area with other prognostic factors. Int Urol Nephrol 2011;43:125-9. [Crossref] [PubMed]
- Li W, Liang C, Liu P, et al. Predictive value of microvessel features for the clinical response to neoadjuvant chemotherapy in cervical squamous carcinoma and the associations with prognosis. Transl Cancer Res 2021;10:162-73. [Crossref] [PubMed]
- Hasan J, Byers R, Jayson GC. Intra-tumoural microvessel density in human solid tumours. Br J Cancer 2002;86:1566-77. [Crossref] [PubMed]
- Goel S, Wong AH, Jain RK. Vascular normalization as a therapeutic strategy for malignant and nonmalignant disease. Cold Spring Harb Perspect Med 2012;2:a006486. [Crossref] [PubMed]
- Jain RK. Normalizing tumor microenvironment to treat cancer: bench to bedside to biomarkers. J Clin Oncol 2013;31:2205-18. [Crossref] [PubMed]
- Jain RK, Martin JD, Stylianopoulos T. The role of mechanical forces in tumor growth and therapy. Annu Rev Biomed Eng 2014;16:321-46. [Crossref] [PubMed]
- Jain RK. Barriers to drug delivery in solid tumors. Sci Am 1994;271:58-65. [Crossref] [PubMed]
- Tamura M, Ohta Y, Kajita T, et al. Plasma VEGF concentration can predict the tumor angiogenic capacity in non-small cell lung cancer. Oncol Rep 2001;8:1097-102. [Crossref] [PubMed]
- Du JR, Jiang Y, Zhang YM, et al. Vascular endothelial growth factor and microvascular density in esophageal and gastric carcinomas. World J Gastroenterol 2003;9:1604-6. [Crossref] [PubMed]
- Saito H, Fukuhara T, Furuya N, et al. Erlotinib plus bevacizumab versus erlotinib alone in patients with EGFR-positive advanced non-squamous non-small-cell lung cancer (NEJ026): interim analysis of an open-label, randomised, multicentre, phase 3 trial. Lancet Oncol 2019;20:625-35. [Crossref] [PubMed]
- Nakagawa K, Garon EB, Seto T, et al. Ramucirumab plus erlotinib in patients with untreated, EGFR-mutated, advanced non-small-cell lung cancer (RELAY): a randomised, double-blind, placebo-controlled, phase 3 trial. Lancet Oncol 2019;20:1655-69. [Crossref] [PubMed]
- Bais C, Mueller B, Brady MF, et al. Tumor Microvessel Density as a Potential Predictive Marker for Bevacizumab Benefit: GOG-0218 Biomarker Analyses. J Natl Cancer Inst 2017;109:djx066. [Crossref] [PubMed]
- Bianconi D, Herac M, Posch F, et al. Microvascular density assessed by CD31 predicts clinical benefit upon bevacizumab treatment in metastatic colorectal cancer: results of the PassionATE study, a translational prospective Phase II study of capecitabine and irinotecan plus bevacizumab followed by capecitabine and oxaliplatin plus bevacizumab or the reverse sequence in patients in mCRC. Ther Adv Med Oncol 2020;12:1758835920928635. [Crossref] [PubMed]
- Park K, Tan EH, O'Byrne K, et al. Afatinib versus gefitinib as first-line treatment of patients with EGFR mutation-positive non-small-cell lung cancer (LUX-Lung 7): a phase 2B, open-label, randomised controlled trial. Lancet Oncol 2016;17:577-89. [Crossref] [PubMed]
- Ge G, Zhou Q, Zhang S, et al. Detection of micro-vascular density(MVD) and its clinical significance in lung cancer. Zhongguo Fei Ai Za Zhi 2000;3:284-7. [Crossref] [PubMed]