Phenotypes of esophageal pressure response to the change of positive end-expiratory pressure in patients with moderate acute respiratory distress syndrome
Highlight box
Key findings
• Esophageal pressure (Pes) changed with end-expiratory pressure (PEEP) adjustment, and two types of Pes phenotypes were identified.
What is known, and what is new?
• Pes has been used as a surrogate of pleural pressure to titrate PEEP in acute respiratory distress syndrome patients. The relationship between Pes and PEEP remains undetermined.
• We proposed a concept of Pes phenotypes with PEEP change. Two types of Pes phenotypes were identified according to the Pes response to the PEEP changes. The PEEP-independent type patients had constant Pes during the PEEP adjustment, while the Pes of the PEEP-dependent patients changed significantly as PEEP changed.
What is the implication, and what should change now?
• Caution should be exercised in the use of Pes measurements to titrate PEEP in both types of patients, and PEEP adjustment can be used to differentiate between the two types of patients.
Introduction
The measurement of esophageal pressure (Pes) has been used for decades, and Pes has been considered an excellent surrogate for pleural pressure (Ppl) (1-4). The EPVent trial showed that the Pes-guided positive end-expiratory pressure (PEEP) setting was associated with better oxygenation and respiratory system compliance (5). However, the LUNG SAFE study showed that less than 1% of patients with acute respiratory distress syndrome (ARDS) received Pes monitoring (1,6). Previously, it was thought that the Pes measurement was complicated and affected by malpositioning, mediastinal or heart artifacts, balloon filling volume, and esophageal muscle stress. However, as its application has increased, these problems have been addressed; the occlusion test and heart artifact can easily confirm the balloon position; the filling test can identify the best filling volume; and the pressure-volume curve of the balloon can be used to exclude the pressure of the esophageal wall (7-9).
Pes measurements were used to titrate PEEP, and by taking a slightly positive end-expiratory transpulmonary pressure (PL) as a reasonable target, repeated airway closure and alveolar collapse might be prevented, which is particularly useful in patients with morbid obesity and ARDS patients with elevated pleural or intra-abdominal pressure (1,10-13). However, research has shown that Pes and chest wall elastance-based methods for estimating Ppl do not yield similar results (14). A subsequent EPVent-2 trial failed to find any significant difference in the survival benefits of Pes monitoring and the other secondary end points for ARDS patients (14,15). The purpose of esophageal manometry is to serve as a surrogate for Ppl to apply the adequate PEEP setting to avoid further lung collapse. However, airway pressure can transmit to the pleural space in varying degrees, and Pes is not simply equivalent to Ppl in titrating PEEP (16).
In a previous study (14) and in our clinical practice, we found that the Pes response (ΔPes) to the change of PEEP in ARDS patients is diverse. Pes is significantly affected by PEEP levels in certain patients. Additionally, when the initial PEEP was set too high empirically, obtaining Pes values to achieve a positive end-expiratory PL can cause barotrauma or hemodynamic instability. Thus, this study sought to examine the ΔPes to the changes in PEEP settings to identify PEEP-dependent and independent Pes phenotypes, and to further summarize the patients’ clinical and respiratory mechanical characteristics, and if possible, to offer some experience for the clinical use of esophageal manometry. We present this article in accordance with the STROBE reporting checklist (available at https://jtd.amegroups.com/article/view/10.21037/jtd-23-771/rc).
Methods
Study population
The study was approved by the Peking Union Medical College Hospital Institutional Review Board (No. ZS-2458) and conducted in accordance with the Declaration of Helsinki (as revised in 2013). Written informed consent was obtained from all the patients or their legal guardians. The study was retrospectively registered at the Clinical Trial Registry (ClinicalTrials.gov; identifier: NCT05442073). Moderate to severe ARDS patients admitted to the intensive care unit from 1 March 2022 to 31 May 2022 were consecutively screened. ARDS was diagnosed in accordance with the Berlin definition that proposes three categories of ARDS based on the severity of hypoxemia, along with explicit criteria related to the timing of the syndrome’s onset, origin of edema, and chest imaging. Moderate and severe ARDS were defined as a partial pressure of arterial oxygen to fraction of inspiratory oxygen ratio (PaO2/FiO2) 101–200 and ≤100 mmHg, respectively (17,18). Patients were excluded from the study if they met any of the following exclusion criteria: (I) were aged <18 years; (II) did not provide informed consent; (III) had contraindications to the insertion of an esophageal catheter, or an esophageal catheter failed to be inserted; (IV) died immediately after admission; (V) were “do-not-resuscitate” or end-stage patients who were admitted for palliative care only.
Research methods
After admission, all the patients were empirically ventilated in the supine position under lung-protected strategies, including small tidal volume (Vt ≈6 mL/kg predicted bodyweight, which was calculated using the World Health Organization’s standards as follows: predicted bodyweight = height minus 105 centimeters (cm) for women, and height minus 100 cm for men, an adequate PEEP, and FiO2 according to the ARDSnet PEEP-FiO2 table and clinical experience to achieve an arterial oxygen saturation of 90–95%. Subsequently, after recording the vital signs, arterial blood gas, and other baseline characteristics, including age, sex, height, weight, body mass index (BMI), which was computed as weight divided by height2 (in m2), Acute Physiology and Chronic Health Evaluation II (APACHE II) score, the reasons for respiratory failure, and ventilator parameters, a gastric tube with an esophageal balloon (SDY-1, AMK Medical, Guangzhou, China) was inserted. The typical depth of insertion was about 30–45 cm. The depth was incrementally adjusted to ensure the correct position, which was confirmed by the visualization of radiopaque markers on chest X-ray, the presence of cardiac oscillation, and the occlusion test simultaneously (5) (Figures S1,S2). The balloon filling test was performed to obtain the pressure-volume curve of the balloon to achieve the optimal inflation volume, which was the filling volume associated with the largest tidal increase of Pes. To further increase the accuracy of the results, the elastance of the esophagus wall (Pew) was extracted from the measured value, with Pew being considered equivalent to the slope of the end-expiratory PV curve of the balloon (19,20).
Respiratory mechanics measurements
To measure the respiratory mechanics, the volume-controlled mode was adopted with 6 ml/ kg predicted body weight of Vt, and no changes in other parameters. The end-expiratory and end-inspiratory Pes at the initial PEEP level were recorded. The plateau pressure was measured during end-inspiratory occlusion (zero-flow). Driving pressure was calculated as: airway plateau pressure − total PEEP. Elastance of the respiratory system (ERS) was calculated as: (airway plateau pressure-total PEEP)/Vt. Elastance of the chest wall (ECW) was calculated as: (end-inspiratory Pes − end-expiratory Pes)/Vt. Elastance of the lung (EL) was calculated as: (end-inspiratory PL − end-expiratory PL)/Vt. PL at end-expiration and end-inspiration were calculated as: airway pressure − Pes at end-expiration occlusion and end-inspiration occlusion, respectively.
In accordance with the aim of the study, the ΔPes to the PEEP changes (ΔPEEP) was evaluated. Previously, it has been reported that 24–37% of airway pressure is transmitted to the pleural space (16). Additionally, hemodynamics can be severely affected when high airway pressure is applied, and the ARDS status of the lung, such as the compliance, resistance, consolidation, and heterogeneity, is not intended to disrupt, thus recruitment maneuvers and extremely low PEEP that might cause the reinflation or the collapse of the lung were not chosen. As a 30% transmission of 3 cmH2O PEEP can cause a 1 cmH2O change of Pes, the PEEP level was designed to change with an average of 3 cmH2O. The increase (PEEP+3 cmH2O) and decrease (PEEP−3 cmH2O) were significant enough to induce Ppl change but not too high to cause lung injury and hemodynamics. After stabilizing for more than 60 seconds (21), the Pes values and other parameters at those PEEP levels were collected again. Depending on the ΔPes to ΔPEEP during PEEP adjustment, the patients were classified into two types: (I) the PEEP-dependent type, which was defined as Δ Pes ≥30% ΔPEEP during both of the series of PEEP adjustment [(i) from PEEP−3 cmH2O to PEEPbaseline; (ii) from PEEPbaseline to PEEP+3 cmH2O]; and (II) the PEEP-independent type, which was defined as Δ Pes <30% ΔPEEP in any series (Figure S3). The baseline characteristics of the two types of patients were compared. The changes of end-expiratory Pes and PL were also compared between the two types.
Statistical analysis
The categorical variables are reported as numbers and percentages and were compared using Chi-squared test. Because of the small sample size, non-normal distribution was assumed. The continuous data are presented as the median and 25th to 75th percentiles. Friedman’s non-parametric test for repeated measures was used to analyze the differences between the three PEEP levels. If there were significant differences, conditions were compared using a Wilcoxon test with Bonferroni’s correction. A two-tailed test was performed, and a P value <0.05 was considered statistically significant. The statistical analyses were conducted using SPSS V.20.0 (SPSS Inc., Chicago, IL, USA).
Results
During the study period, a total of 33 consecutive moderate to severe ARDS patients were admitted to our intensive care unit, and 18 patients were enrolled in our study (Figure S4). The study population had a median age of 64 [53, 75] years, and 55.6% were male. The patients had a median BMI of 25.4 (21.5, 28.0) kg/m2, and an APACHE II scored of 19.0 (15.5, 27.0). The median PaO2/FiO2 ratio at admission was 175.5 (152.8, 188.3), and sepsis was the most common cause of ARDS (eight cases, 44.4%). The median partial pressure of arterial carbon dioxide (PaCO2) and Vt per predicted body weight were 41.0 (39.0, 42.3) mmHg and 6.4 (6.1, 6.6) mL/kg, respectively. The baseline PEEP and plateau pressure levels were 12.0 [10.0, 14.0] and 25.5 (22.8, 28.0) cmH2O respectively, with a calculated driving pressure of 13.0 (11.8, 16.0) cmH2O (Table 1).
Table 1
Variables | Total patients (N=18) | PEEP-dependent type (N=12) | PEEP-independent type (N=6) |
P* |
---|---|---|---|---|
Baseline characteristics | ||||
Male sex | 10 (55.6) | 7 (58.3) | 3 (50.0) | 0.737 |
Age (years) | 64 [53, 75] | 62 [50, 76] | 69 [60, 73] | 0.437 |
Height (cm) | 166.5 [160, 173] | 168.5 [165.3, 173] | 160 [158, 163.8] | 0.067 |
Actual body weight (kg) | 70.0 [59.5, 77.0] | 67.5 [55.8, 74.5] | 65.0 [56.5, 74.8] | 0.102 |
BMI (kg/m2) | 25.4 [21.5, 28.0] | 23.5 [19.7, 25.5] | 28.7 [26.9, 30.6] | 0.003 |
APACHE II | 19.0 [15.5, 27.0] | 20.5 [14.5, 27] | 18.5 [15.5, 28] | 1.000 |
Reasons for respiratory failure | 0.853 | |||
Pneumonia | 5 (27.8) | 3 (25.0) | 2 (33.3) | |
Sepsis (non-pneumonia) | 8 (44.4) | 6 (50.0) | 2 (33.3) | |
Pancreatitis | 2 (11.1) | 1 (8.3) | 1 (16.7) | |
Cardiogenic pulmonary edema | 1 (5.6) | 1 (8.3) | 0 | |
Trauma | 2 (11.1) | 1 (8.3) | 1 (16.7) | |
Respiratory parameters | ||||
PaO2 (mmHg) | 73.5 [65.0, 79.3] | 75.5 [66.0, 79.8] | 71.0 [63.5, 80.8] | 0.616 |
FiO2 (%) | 45.0 [40.0, 46.3] | 45.0 [41.2, 48.8] | 40.0 [40.0, 46.3] | 0.213 |
PaO2/FiO2 | 175.5 [152.8, 188.3] | 174.5 [136.3, 186] | 177.5 [148.3, 195.8] | 0.553 |
PaCO2 (mmHg) | 41.0 [39.0, 42.3] | 39.9 [38.1, 41.2] | 42.4 [40.9, 46.0] | 0.024 |
Respiratory rate (beats per minute) | 20 [18, 21] | 19 [18, 22] | 21 [19, 21] | 0.385 |
Tidal volume (mL) | 390 [348, 428] | 410 [380, 443] | 360 [328, 428] | 0.250 |
Tidal volume (mL/kg predicted body weight) | 6.4 [6.1, 6.6] | 6.4 [6.1, 6.6] | 6.3 [6.0, 7.8] | 0.820 |
PEEP at baseline (cmH2O) | 12.0 [10.0, 14.0] | 12.0 [10.5,14.8] | 12.0 [10.0, 14.3] | 0.750 |
End-expiratory Pes (cmH2O) | 12.9 [11.6, 15.8] | 12.5 [10.5, 14.9] | 15.5 [11.6, 17.8] | 0.180 |
Plateau pressure (cmH2O) | 25.5 [22.8, 28.0] | 23.5 [22.0, 25.8] | 28.0 [27.8, 29.5] | 0.001 |
Driving pressure (cmH2O) | 13.0 [11.8, 16.0] | 12.0 [11.0, 13.0] | 16.0 [15.8, 17.3] | <0.0001 |
End-inspiratory Pes (cmH2O) | 17.3 [14.6, 20.3] | 17.0 [15.2, 18.8] | 18.5 [13.5, 22.5] | 0.616 |
ECW (cmH2O/L) | 9.6 [5.8, 13.9] | 9.6 [6.7, 14.0] | 7.8 [4.9, 11.8] | 0.385 |
EL (cmH2O/L) | 22 [14.2, 33.4] | 18.3 [13.1, 23.3] | 35.8 [31.2, 40.5] | <0.0001 |
ERS (cmH2O/L) | 31.8 [27.9, 41.2] | 28.6 [24.6, 32.1] | 45.9 [38.9, 48.9] | <0.0001 |
EL/ERS | 0.68 [0.60, 0.81] | 0.64 [0.51, 0.71] | 0.81 [0.71, 0.90] | 0.007 |
Data are expressed as median [interquartile range] or n (%). *, P values for comparisons between PEEP-dependent type and PEEP-independent type patients. Elastance of the respiratory system (ERS) was calculated as (airway plateau pressure − total PEEP)/Vt. Elastance of chest wall (ECW) was calculated as (end-inspiratory esophageal pressure − end-expiratory esophageal pressure)/Vt. Elastance of the lung (EL) was calculated as (end-inspiratory transpulmonary pressure − end-expiratory transpulmonary pressure)/Vt. Plateau pressure was obtained during end-inspiratory occlusion (zero-flow). Driving pressure was calculated as airway plateau pressure − total PEEP. Transpulmonary pressure (PL) at end-expiration and end-inspiration were calculated as airway pressure − esophageal pressure at end-expiration occlusion and end-inspiration occlusion, respectively. ARDS, acute respiratory distress syndrome; BMI, body mass index, which was computed as weight divided by height2 (in m2); APACHE II, acute physiology and chronic health evaluation II; PaO2, arterial partial pressure of oxygen; FiO2, fraction of inspired oxygen; PaCO2, partial pressure of carbon dioxide; PEEP, positive end-expiratory pressure; Pes, esophageal pressure.
The PEEP level was designed to change at an average of 3 cmH2O; however, the hemodynamics of certain patients was severely affected when high airway pressure was applied clinically, and as a result the PEEP level was changed from 2 to 5 cmH2O, with a median of 3 cmH2O (Table S1). Of the 18 patients, 12 were classified as the PEEP-dependent type, while the other six patients were the PEEP-independent type. The BMI of the dependent type patients was significantly lower than that of the independent type patients [23.5 (19.7, 25.5) vs. 28.7 (26.9, 30.6) kg/m2, P=0.003]. Compared with the PEEP-independent type patients, the PEEP-dependent type patients had lower levels of PaCO2 and plateau pressure [39.9 (38.1, 41.2) vs. 42.4 (40.9, 46.0) mmHg, P=0.024, 23.5 (22.0, 25.8) vs. 28.0 (27.8, 29.5) cmH2O, P=0.001, respectively]. The PEEP-dependent type patients had significantly lower driving pressure (12.0 vs. 16.0 cmH2O, P<0.0001). The median ERS and EL of all these patients were 31.8 and 22 cmH2O/L respectively, with the PEEP-dependent patients having significantly lower values (28.6 vs. 45.9 cmH2O/L, P<0.0001; and 18.3 vs. 35.8 cmH2O, P<0.0001, respectively). The ECW of the PEEP-dependent patients was slightly higher than that of the PEEP-independent type patients (9.6 vs. 7.8 cmH2O/L, P=0.385). There were no significant differences in the other baseline characters between the two types of patients (Table 1).
The median PEEP value at the baseline of the PEEP-dependent type patients was 12 (10.5, 14.8) cmH2O, and the corresponding Pes was 12.5 (10.5, 14.9) cmH2O. When the PEEP level was decreased to 9 (7.5, 11.8) cmH2O, the value of the Pes decreased significantly [9.8 (6.9, 11.5) for PEEP−3 cmH2O, P<0.0001). When the PEEP level increased to 15 [14, 18] cmH2O, the value of the Pes also increased to 14.5 (13.1, 18.3) cmH2O significantly [P<0.0001). During the PEEP adjustment, the end-expiratory PL continued to be maintained in a tight range of –2 to +2 cmH2O [Table 2 and Figure 1A,1B). In the PEEP-independent type patients, when the PEEP level decreased to 9.0 (7, 11.3) cmH2O from the baseline [12.0 (10.0, 14.3)], the Pes did not significantly change [15.5 (11.6, 17.8) vs. 15.4 (11.4, 17.8), P=0.509]. When the PEEP increased to 15 (12, 16.5) cmH2O, the Pes values also remained constant. However, with PEEP increment, the calculated end-expiratory PL statistically improved [–5.5 (–7.8, –4.4) cmH2O at PEEP−3 cmH2O, –2.5 (–5.0, –1.6) cmH2O at PEEPbaseline, and –0.5 (–1.8, 0.3) cmH2O at PEEP+3 cmH2O; P<0.0001 and P=0.001, respectively] (Table 2 and Figure 1C,1D). The Pes and end-expiratory PL changes of each patient with PEEP adjustment are listed in Table S1.
Table 2
Variables | PEEP-dependent type (n=12) | PEEP-independent type (n=6) |
---|---|---|
PEEPbaseline (cmH2O) | ||
PEEP level | 12 (10.5,14.8) | 12.0 (10.0, 14.3) |
Pes | 12.5 (10.5, 14.9) | 15.5 (11.6, 17.8) |
End-expiratory transpulmonary pressure | −0.2 (−0.7, 0.4) | −2.5 (−5.0, −1.6) |
PEEP−3 cmH2O (cmH2O) | ||
PEEP level | 9 (7.5, 11.8) | 9 (7, 11.3) |
Pes | 9.8 (6.9, 11.5) | 15.4 (11.4, 17.8) |
End-expiratory transpulmonary pressure | 0.1 (−0.6, 0.5) | −5.5 (−7.8, −4.4) |
PEEP+3 cmH2O (cmH2O) | ||
PEEP level | 15 (14, 18) | 15 (12, 16.5) |
Pes | 14.5 (13.1, 18.3) | 15.4 (11.7, 18.3) |
End-expiratory transpulmonary pressure | 0.3 (−0.3, 0.7) | −0.5 (−1.8, 0.3) |
Data are expressed as the median (interquartile range). Pes, esophageal pressure; PEEP, positive end-expiratory pressure; PEEP−3 cmH2O, decrease 3 cmH2O from the baseline PEEP level; PEEP+3 cmH2O, increase 3 cmH2O from the baseline PEEP level.
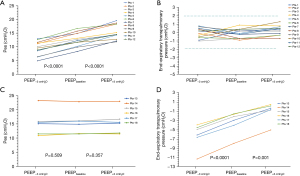
Discussion
For the first time, we demonstrated the effect of PEEP on the Pes measurement. Patients were divided into PEEP-dependent and PEEP-independent Pes phenotypes according to the ΔPes to the change of PEEP. The PEEP-independent type patients had constant Ppl levels with PEEP adjustment, while the PEEP-dependent type patients had various Ppl levels during ΔPEEP. The PEEP-dependent type patients had a lower BMI and higher respiratory system compliance than the PEEP-independent type patients.
The different Pes phenotypes might be associated with complete airway closure. Airway closure occurs when pressure outside the airway, which is more or less equal to the Ppl, is higher than pressure inside the airway. Complete airway closure might occur in most PEEP-independent type patients, and the set PEEP is still lower than Ppl. Coudroy et al. (22) found that, 41% of ARDS patients experienced complete airway closure. Due to the widespread prevalence of airway closure, when the total PEEP was lower than the airway opening pressure, the calculation of transpulmonary was biased, and subsequently led to a higher PEEP setting. The incidence of complete airway closure was associated with BMI; the higher the BMI, the higher the incidence rate of complete airway closure. When the BMI was greater than 40 kg/m2, the incidence of airway closure was as high as 65%. Meanwhile end-expiratory Pes was found to be positively associated with BMI, but not with ECW. The results of our study were consistent with this finding. We found that PEEP-independent type patients had a higher BMI, and higher end-expiratory Pes values than PEEP-dependent type patients, which could be attributed to airway closure. Unfortunately, complete airway closure was not assessed in our study, but the initial PEEP setting at the baseline for our patients was 12 cmH2O, which was higher than the median airway opening pressure. The relatively high PEEP setting in our study effectively avoided the effect of complete airway closure on the results. However, the association between complete airway closure and Pes phenotypes requires further assessment.
This phenomenon might also be explained by the relationship between the Pes and the Ppl, and the Ppl gradient due to the heterogeneity of ARDS lungs. The Pes has been found to correlate well with the Ppl in dependent lung regions (23). We found that the PEEP-dependent type patients had lower EL and ERS, and also a lower EL/ERS ratio than the PEEP-independent type patients, but there was no significant difference in ECW between the two groups (Table 1). The PEEP-dependent type patients showed better lung compliance, which could exhibit better conductive transmission. Endotracheal pressure could transmit to the pleura in dependent regions, which was reflected by the Pes changing with the PEEP adjustment. Decades ago, Jardin et al. (16) evaluated airway pressure transmission using the relationship between tracheal pressure and Pes and found that lung stiffness influenced the transmission because of the damping effect, with higher transmission in patients with higher respiratory system compliance (16). Conversely, the transmission of PEEP might have been much lower to the pleura in the dependent regions in the PEEP-independent patients who showed little or no changes in Pes with PEEP titration, as the pressure was more distributed to the pleura in the non-dependent regions. Our study further confirmed these transmission differences and obtained more accurate Pes measurements and focused on the end-expiratory phase. Further, taking into account the change of PL simultaneously, the results are more conducive to the clinical application of PEEP titration guided by Pes.
We found that the PEEP-independent type patients had higher end-expiratory Ppl, but lower Ecw based on the Pes. In patients with a higher BMI, higher abdominal pressure compressed the lung through the diaphragm. In the spontaneously breathing patients, end-expiratory Ppl changed little, as the equilibrium of the lung was re-established by decompressing the lung volume through the airway opening to the atmosphere. As for the patients who received invasive controlled ventilation with a closed airway, the unreleased pressure acted on the pleura. Just as in the PEEP-independent type patients who had a high BMI and ERS, when the patients had a Ppl that was too high, the inspiratory airway pressure had to overcome the Ppl before opening the lung. Thus, PEEP-independent type patients needed increased PEEP to overcome the external pressure applied to the pleura (e.g., the higher abdominal pressure) to maintain functional residual capacity for the best lung compliance (13,24). Esophageal manometry with PEEP adjustment could be used to identify the different types of patients and thus to apply higher but more optimal PEEP to provide better treatment.
Measuring Pes might be of great importance in identifying the optimal PEEP required to prevent alveolar collapse. The aim of positive end-expiratory PL was achieved by an incremental PEEP titration; however, patients were at high risk of hemodynamic instability and lung overdistension, especially in the non-dependent regions of the lung (25,26). Yoshida et al. (23) examined the Ppl gradient between the non-dependent and dependent regions in lung-injured pigs and human cadavers, and they found that Pes correlated well with the Ppl in the dependent lung regions. In the PEEP-independent patients, Pes did not change with PEEP adjustment, as the pressure was more distributed to the pleura in non-dependent regions. Thus, the PEEP increment in these patients significantly increased the risk of overdistension.
Due to the heterogeneity of ARDS lungs, it was impossible to achieve full lung recruitment without overdistension simply through airway pressure titration. This could also be the reasons why the EPVent 2 trial failed to demonstrate the superiority of the Pes-guided PEEP strategy. The differences in PL among lung regions may introduce pendelluft, which can be monitored at the bedside with electrical impedance tomography (EIT) (27). A previous study showed that the PEEP can decrease the level of pendelluft in spontaneously breathing subjects; however, it was unclear whether PEEP could alter the regional PL (28). Further research should combine the Pes and EIT to explore the correlation between the presented Pes phenotypes and pendelluft. The prone position reduces the dorsal-ventral Ppl gradient, and homogenization was much less dependent on the PEEP levels in the prone than the supine position (29). Therefore, the different ΔPes to PEEP change could be overcome by placing patients in the prone position, and Pes could be much more “PEEP-dependent” in the prone position.
As one of the few PEEP titration methods available in clinical practice, esophageal manometry has a unique value, but the results should be cautiously interpreted. The barotrauma, hemodynamic effect of high PEEP is always an issue. Our study identified two types of Pes responses to ΔPEEP. For PEEP-independent patients especially, a relatively high PEEP should be cautiously used to re-open a collapsed lung, as the Pes value does not reflect the pressure transmission accurately. The relationship between Pes and PEEP is very complicated and deserves further exploration using additional relevant physiologic data. Airway closure and lower transmission to the pleura where esophageal manometry was located are two different reasons explaining the two Pes phenotypes and represent two different titration strategies. This study might have provided a potential method for solving the problems of applying esophageal manometry to guide the PEEP setting (15,30). Through the ΔPes to PEEP increase or decrease of 3–5 cmH2O, two Pes phenotypes were identified without sophisticated calculation and analysis. Regrettably, this study was not able to examine the underlying mechanisms of the Pes responses to PEEP changes, but it was the first attempt to explore the sophisticated physiology.
Limitations
The sample size of the current study was relatively small. More ARDS patients are needed to validate the findings. Additionally, the median baseline PEEP in our study was 12 cmH2O, which was increased to 15 cmH2O during PEEP adjustment. It would be challenging to determine the phenotypes of patients with higher initial PEEP levels. Third, the proposed conceptual Pes phenotypes might be useful for the clinical application of using Pes to guide PEEP titration, and the subsequent effect of phenotype identification needs to be tested on clinical outcomes (e.g., mechanical ventilation days and survival rate) in randomized-controlled settings.
Conclusions
We proposed a concept of Pes phenotypes with PEEP change. Two types of Pes phenotypes were recognized according to the ΔPes to ΔPEEP. The PEEP-independent type patients had constant Pes during the PEEP adjustment, while the Pes of the PEEP-dependent patients changed significantly as PEEP changed. The underlying mechanisms of the different Pes responses require further exploration, and caution should be exercised in the use of Pes measurements to titrate PEEP in both types of patients.
Acknowledgments
Funding: This study was supported by
Footnote
Reporting Checklist: The authors have completed the STROBE reporting checklist. Available at https://jtd.amegroups.com/article/view/10.21037/jtd-23-771/rc
Data Sharing Statement: Available at https://jtd.amegroups.com/article/view/10.21037/jtd-23-771/dss
Peer Review File: Available at https://jtd.amegroups.com/article/view/10.21037/jtd-23-771/prf
Conflicts of Interest: All authors have completed the ICMJE uniform disclosure form (available at https://jtd.amegroups.com/article/view/10.21037/jtd-23-771/coif). All authors report that this study was supported by the National High-Level Hospital Clinical Research Funding (Nos. 2022-PUMCH-D-005 and 2022-PUMCH-B-115) and Special Fund for Youth Medical Research of China International Medical Foundation (CIMF) (No. Z-2018-35-1902). The authors have no other conflicts of interest to declare.
Ethical Statement: The authors are accountable for all aspects of the work, in ensuring that questions related to the accuracy or integrity of any part of the work appropriately investigated and resolved. The study was approved by the Peking Union Medical College Hospital Institutional Review Board (No. ZS-2458) and conducted in accordance with the Declaration of Helsinki (as revised in 2013). Written informed consent was obtained from all the patients or their legal guardians.
Open Access Statement: This is an Open Access article distributed in accordance with the Creative Commons Attribution-NonCommercial-NoDerivs 4.0 International License (CC BY-NC-ND 4.0), which permits the non-commercial replication and distribution of the article with the strict proviso that no changes or edits are made and the original work is properly cited (including links to both the formal publication through the relevant DOI and the license). See: https://creativecommons.org/licenses/by-nc-nd/4.0/.
References
- Pham T, Telias I, Beitler JR. Esophageal Manometry. Respir Care 2020;65:772-92. [Crossref] [PubMed]
- Yoshida T, Brochard L. Esophageal pressure monitoring: why, when and how? Curr Opin Crit Care 2018;24:216-22. [Crossref] [PubMed]
- Akoumianaki E, Maggiore SM, Valenza F, et al. The application of esophageal pressure measurement in patients with respiratory failure. Am J Respir Crit Care Med 2014;189:520-31. [Crossref] [PubMed]
- Chiumello D, Cressoni M, Colombo A, et al. The assessment of transpulmonary pressure in mechanically ventilated ARDS patients. Intensive Care Med 2014;40:1670-8. [Crossref] [PubMed]
- Talmor D, Sarge T, Malhotra A, et al. Mechanical ventilation guided by esophageal pressure in acute lung injury. N Engl J Med 2008;359:2095-104. [Crossref] [PubMed]
- Bellani G, Laffey JG, Pham T, et al. Epidemiology, Patterns of Care, and Mortality for Patients With Acute Respiratory Distress Syndrome in Intensive Care Units in 50 Countries. JAMA 2016;315:788-800. [Crossref] [PubMed]
- Yoshida T, Brochard L. Ten tips to facilitate understanding and clinical use of esophageal pressure manometry. Intensive Care Med 2018;44:220-2. [Crossref] [PubMed]
- Baedorf Kassis E, Talmor D. Clinical application of esophageal manometry: how I do it. Crit Care 2021;25:6. [Crossref] [PubMed]
- Chiumello D, Consonni D, Coppola S, et al. The occlusion tests and end-expiratory esophageal pressure: measurements and comparison in controlled and assisted ventilation. Ann Intensive Care 2016;6:13. [Crossref] [PubMed]
- Chen L, Del Sorbo L, Grieco DL, et al. Airway Closure in Acute Respiratory Distress Syndrome: An Underestimated and Misinterpreted Phenomenon. Am J Respir Crit Care Med 2018;197:132-6. [Crossref] [PubMed]
- Fumagalli J, Berra L, Zhang C, et al. Transpulmonary Pressure Describes Lung Morphology During Decremental Positive End-Expiratory Pressure Trials in Obesity. Crit Care Med 2017;45:1374-81. [Crossref] [PubMed]
- Krebs J, Pelosi P, Tsagogiorgas C, et al. Effects of positive end-expiratory pressure on respiratory function and hemodynamics in patients with acute respiratory failure with and without intra-abdominal hypertension: a pilot study. Crit Care 2009;13:R160. [Crossref] [PubMed]
- Pérez J, Dorado JH, Navarro E, et al. Mechanisms of lung and diaphragmatic protection by high PEEP in obese COVID-19 ARDS: role of the body mass index. Crit Care 2022;26:182. [Crossref] [PubMed]
- Gulati G, Novero A, Loring SH, et al. Pleural pressure and optimal positive end-expiratory pressure based on esophageal pressure versus chest wall elastance: incompatible results*. Crit Care Med 2013;41:1951-7. [Crossref] [PubMed]
- Beitler JR, Sarge T, Banner-Goodspeed VM, et al. Effect of Titrating Positive End-Expiratory Pressure (PEEP) With an Esophageal Pressure-Guided Strategy vs an Empirical High PEEP-Fio2 Strategy on Death and Days Free From Mechanical Ventilation Among Patients With Acute Respiratory Distress Syndrome: A Randomized Clinical Trial. JAMA 2019;321:846-57. [Crossref] [PubMed]
- Jardin F, Genevray B, Brun-Ney D, Bourdarias JP. Influence of lung and chest wall compliances on transmission of airway pressure to the pleural space in critically ill patients. Chest 1985;88:653-8. [Crossref] [PubMed]
- Fan E, Brodie D, Slutsky AS. Acute Respiratory Distress Syndrome: Advances in Diagnosis and Treatment. JAMA 2018;319:698-710. [Crossref] [PubMed]
- Thompson BT, Chambers RC, Liu KD. Acute Respiratory Distress Syndrome. N Engl J Med 2017;377:562-72. [Crossref] [PubMed]
- Sun XM, Chen GQ, Huang HW, et al. Use of esophageal balloon pressure-volume curve analysis to determine esophageal wall elastance and calibrate raw esophageal pressure: a bench experiment and clinical study. BMC Anesthesiol 2018;18:21. [Crossref] [PubMed]
- Mojoli F, Iotti GA, Torriglia F, et al. In vivo calibration of esophageal pressure in the mechanically ventilated patient makes measurements reliable. Crit Care 2016;20:98. [Crossref] [PubMed]
- Chiumello D, Coppola S, Froio S, et al. Time to reach a new steady state after changes of positive end expiratory pressure. Intensive Care Med 2013;39:1377-85. [Crossref] [PubMed]
- Coudroy R, Vimpere D, Aissaoui N, et al. Prevalence of Complete Airway Closure According to Body Mass Index in Acute Respiratory Distress Syndrome. Anesthesiology 2020;133:867-78. [Crossref] [PubMed]
- Yoshida T, Amato MBP, Grieco DL, et al. Esophageal Manometry and Regional Transpulmonary Pressure in Lung Injury. Am J Respir Crit Care Med 2018;197:1018-26. [Crossref] [PubMed]
- Marini JJ, Gattinoni L. Improving lung compliance by external compression of the chest wall. Crit Care 2021;25:264. [Crossref] [PubMed]
- Bachmann MC, Morais C, Bugedo G, et al. Electrical impedance tomography in acute respiratory distress syndrome. Crit Care 2018;22:263. [Crossref] [PubMed]
- He H, Chi Y, Long Y, et al. Influence of overdistension/recruitment induced by high positive end-expiratory pressure on ventilation-perfusion matching assessed by electrical impedance tomography with saline bolus. Crit Care 2020;24:586. [Crossref] [PubMed]
- Chi Y, Zhao Z, Frerichs I, et al. Prevalence and prognosis of respiratory pendelluft phenomenon in mechanically ventilated ICU patients with acute respiratory failure: a retrospective cohort study. Ann Intensive Care 2022;12:22. [Crossref] [PubMed]
- Yoshida T, Roldan R, Beraldo MA, et al. Spontaneous Effort During Mechanical Ventilation: Maximal Injury With Less Positive End-Expiratory Pressure. Crit Care Med 2016;44:e678-88. [Crossref] [PubMed]
- Katira BH, Osada K, Engelberts D, et al. Positive End-Expiratory Pressure, Pleural Pressure, and Regional Compliance during Pronation: An Experimental Study. Am J Respir Crit Care Med 2021;203:1266-74. [Crossref] [PubMed]
- Writing Group for the Alveolar Recruitment for Acute Respiratory Distress Syn-drome Trial (ART) Investigators. Effect of Lung Recruitment and Titrated Positive End-Expiratory Pressure (PEEP) vs Low PEEP on Mortality in Patients With Acute Respiratory Distress Syndrome: A Randomized Clinical Trial. JAMA 2017;318:1335-45. [Crossref] [PubMed]
(English Language Editor: L. Huleatt)