Comparison of early postoperative pulmonary complications between two-lung ventilation with artificial pneumothorax and one-lung ventilation with bronchial blockade in patients undergoing minimally invasive esophagectomy: a retrospective propensity score-matched cohort study
Highlight box
Key findings
• Compared with one-lung ventilation (OLV) with bronchial blockade, two-lung ventilation (TLV) with CO2 pneumothorax did not reduce the incidence of early postoperative pulmonary complications (PPCs) after minimally invasive esophagectomy (MIE).
What is known and what is new?
• TLV with artificial CO2 pneumothorax is used in patients undergoing MIE in the prone position. TLV is associated with shorter operating time, less blood loss, and shorter hospital stay than OLV. Research on the impact of TLV with artificial CO2 pneumothorax on PPCs after MIE is limited and inconclusive.
• This study aimed to compare early PPCs between TLV with CO2 pneumothorax and OLV in patients who underwent MIE. In previous studies comparing TLV and OLV for esophagectomy, nearly all OLV patients were intubated using a DLET. In the present study, bronchial blocker was used in the OLV group, and we focused on PPCs.
What is the implication, and what should change now?
• Additional research is warranted to better comprehend the impact of TLV on PPCs after MIE, particularly large-scale randomized controlled trials.
Introduction
Esophageal cancer is one of the most common cancers in China and the sixth most common cause of cancer-related death worldwide (1). Esophagectomy is the definitive treatment. Esophagectomy is associated with a high incidence of postoperative complications and high rates of procedure-related morbidity and mortality (2). Pulmonary complications are most frequent. Minimally invasive esophagectomy (MIE) is superior to open esophagectomy in terms of recovery time, incidence of perioperative complications, and short-term outcomes (3-5). Nevertheless, postoperative pulmonary complications (PPCs) remain a primary concern in patients undergoing MIE.
During most thoracic surgeries, one-lung ventilation (OLV) is typically used. With patients undergoing MIE in the prone position, two-lung ventilation (TLV) with artificial carbon dioxide (CO2) pneumothorax is used during the thoracoscopic phase of the operation (6,7). TLV is associated with higher oxygenation during the thoracoscopic phase, shorter operating time, less blood loss, and shorter hospital stay than OLV (8-10). Additionally, because a single-lumen endotracheal tube (SLET) is employed with TLV, TLV is expected to be associated with fewer airway injuries, shorter intubation time, and reduced cost (8,11). Better lung protection is also expected with MIE performed in the prone position since OLV, a known risk factor for lung damage, can be avoided (12). However, research on the impact of TLV with artificial CO2 pneumothorax on PPCs after MIE is limited and inconclusive (9,13).
OLV during MIE can be performed using either a double-lumen endotracheal tube (DLET) or a bronchial blocker (12,14,15). Use of a DLET has been associated with higher risk of airway injury and PPCs; moreover, it might increase the difficulty of lymph node dissection along the left recurrent laryngeal nerve (RLN) (8,11,16-18). Use of a bronchial blocker reduces the risk of those drawbacks (17-21). Accordingly, some institutions have begun to routinely use bronchial blockade for OLV during MIE (22). In previous studies comparing OLV and TLV for esophagectomy, however, nearly all OLV patients were intubated using a DLET (13,16,23). Only one previous study compared TLV and OLV with bronchial blockade in patients undergoing MIE, and pulmonary complications were not their main focus (9). Therefore, this study aimed to compare early PPCs between TLV with CO2 pneumothorax and OLV with a bronchial blocker in patients who underwent MIE. We present this article in accordance with the STROBE reporting checklist (available at https://jtd.amegroups.com/article/view/10.21037/jtd-23-1667/rc).
Methods
Study population
This study was performed at the Peking University Cancer Hospital and registered in the Chinese Clinical Trial Registry (No. ChiCTR2300071571). The study was conducted in accordance with the Declaration of Helsinki (as revised in 2013). Ethics committee approval was obtained from the Institutional Review Board at Peking University Cancer Hospital (No. 2023YJZ36). The requirement for written informed consent was waived because of the study’s retrospective design. Patients diagnosed with esophageal cancer and scheduled for elective MIE with two-field lymph node dissection via thoracoscopy and laparoscopy between August 2018 and February 2023 were reviewed. Those with cervical esophageal carcinoma were excluded from the study because of their distinct oncological characteristics and therapeutic regimens. We also excluded patients with the following characteristics: recurrent esophageal carcinoma, American Society of Anesthesiologists class IV or higher, DLET intubation, coronavirus disease 2019 diagnosis within 1 month prior to surgery or during hospitalization, change in operation method during surgery (e.g., conversion to open procedure or unplanned additional excision of other organs), and incomplete data. Each operation was performed by one of three experienced thoracic surgeons. Postoperative care was provided in the general ward or intensive care unit (ICU) as appropriate. Patient characteristics and surgical and postoperative data were retrieved from the electronic medical records. Chronic obstructive pulmonary disease, asthma, bronchiectasis, pulmonary bulla, lung infection within 1 month, and pulmonary tuberculosis were considered preoperative pulmonary comorbidities.
Anesthesia and surgical procedures
General anesthesia was managed by the same group of thoracic anesthesiologists. Standard monitoring was employed using electrocardiography, pulse oximetry, arterial pressure transduction, and a bispectral index monitor. Arterial blood gases were routinely measured before induction and after extubation; intraoperative measurements were determined by the attending anesthesiologist. Intravenous sufentanil/oxycodone, propofol/etomidate, and cisatracurium/rocuronium were used for induction. Patients in the TLV group were intubated using a SLET. The thoracic procedure was completed under TLV with artificial pneumothorax (CO2 was insufflated to a pressure of 8 to 10 mmHg to facilitate partial collapse of the right lung). Patients in the OLV group were first intubated using a SLET; then a bronchial blocker was placed in the right bronchus under fiberoptic guidance. Use of a bronchial blocker is at the attending anesthesiologists’ discretion. Tidal volume during the thoracic phase of surgery was set at 4 to 6 mL/kg (calculated based on predicted body weight) in both groups; it was set at 6 mL/kg throughout the remainder of the procedure. Respiratory rate was set at 14 to 20 breaths per minute and modified to maintain an end-tidal CO2 of 35 to 45 mmHg. General anesthesia was maintained using inhaled sevoflurane and continuous infusions of propofol and remifentanil; muscle relaxation was achieved using intermittent cisatracurium/rocuronium. Postoperative analgesia was administered using patient-controlled intravenous analgesia.
The surgical procedure comprised a right transthoracic subtotal esophagectomy, mediastinal and abdominal lymph node dissection, and anastomosis in the neck. The thoracic procedures were performed in the left lateral-prone position using a thoracoscope (four trocars were placed in the fourth and seventh intercostal spaces in the mid-axillary line and the sixth and ninth intercostal spaces in the subscapular line). The abdominal procedures were carried out laparoscopically. Gastric tube reconstruction was performed with a cervical anastomosis. After surgery, patients were transported to the post-anesthesia care unit. Neostigmine was routinely used for the reversal of neuromuscular blockade, and the recovery of neuromuscular blockade was monitored before extubation. Patients were then transferred to the ward after recovery from anesthesia. Patients who required continued mechanical ventilation or had significant comorbidities were transferred to the ICU.
Measurements
PPC data were gathered while the patient was in the hospital. Given the assumption that PPCs associated with intraoperative ventilation would appear early, the primary outcome was incidence of pneumonia in the first 3 days after surgery (24). Pneumonia was defined as a new or progressive radiological infiltrate and at least two of the following: body temperature >38 ℃, leukocytosis or leukopenia, and purulent secretions (25). The secondary outcome was incidence of other major PPCs in the first 3 days, including pleural effusion requiring drainage, pneumothorax requiring treatment, atelectasis mucous plugging requiring bronchoscopy, respiratory failure requiring invasive or noninvasive ventilation, acute respiratory distress syndrome, acute aspiration, and tracheobronchial injury (adapted from a proposal by the Esophagectomy Complications Consensus Group) (26). The number of re-intubations and tracheotomies in the first 3 days, occurrence of major non-pulmonary postoperative complications including RLN injury, anastomotic leak, wound infection, and chylothorax, and length of hospital and ICU stays were also recorded.
Statistical analysis
Statistical analyses were performed using SPSS software version 26.0 (IBM Corp., Armonk, NY, USA). Categorical data were presented as numbers with percentage and were compared using the Pearson chi-square test or Fisher’s exact test. Continuous data with a normal distribution were presented as means with standard deviation and were compared using the independent-samples t-test. Data with a non-normal distribution were presented as medians with interquartile range (IQR) and were compared using the Mann-Whitney U test. Propensity score matching (PSM) was performed to reduce confounding bias by balancing group differences in patient characteristics and PPC risk factors. Baseline characteristics with P<0.1 in univariate comparisons were included for propensity score calculations, as were known PPC risk factors [age, body mass index (BMI), pulmonary comorbidities, smoking status, intraoperative fluid intake, operative blood loss, and duration of surgery] (27-33). Propensity scores were created for each patient using a multivariate logistic regression model. Patients were matched at a 1:3 ratio between the OLV group and the TLV group using the nearest neighbor method without replacement and a caliper width of 0.2 of the standard deviation of the logit of the estimated propensity score. A standardized difference in means <0.1 between the groups was considered to indicate an adequate balance of matching. P<0.05 was considered significant.
Results
Among the 635 eligible patients, 593 met criteria and were included for analysis (513 in the TLV group and 80 in the OLV group). The PSM algorithm selected 197 individuals from the TLV group and 73 from the OLV group for analysis (Figure 1). Table 1 shows patient characteristics in the groups before and after PSM. Patient characteristics did not significantly differ between the groups. In terms of intraoperative data, the groups significantly differed in terms of intraoperative fluid intake, operative blood loss volume, surgery duration, and anesthesia duration before PSM. After matching, intraoperative data were comparable between the groups except for anesthesia duration (P<0.01), which was defined as the time between entering and leaving the operating room (Table 2). The standardized disparities before and after matching significantly decreased, which suggested that the matched groups were homogenous.
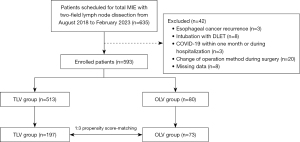
Table 1
Characteristics | Before matching (n=593) | After matching (n=270) | |||||
---|---|---|---|---|---|---|---|
TLV group (n=513) | OLV group (n=80) | P value | TLV group (n=197) | OLV group (n=73) | P value | ||
Age, years | 64.0 (58.0–68.0) | 65.5 (60.0–68.8) | 0.12 | 64.0 (59.0–68.0) | 65.0 (59.0–68.0) | 0.61 | |
Sex | 0.60 | 0.91 | |||||
Male | 424 (82.7) | 68 (85.0) | 169 (85.8) | 63 (86.3) | |||
Female | 89 (17.3) | 12 (15.0) | 28 (14.2) | 10 (13.7) | |||
Height, cm | 166.0 (162.0–171.0) | 167.8 (163.3–172.8) | 0.13 | 166.0 (162.0–171.5) | 167.5 (163.5–172.5) | 0.29 | |
Weight, kg | 65.0 (57.5–71.0) | 67.0 (59.3–73.8) | 0.13 | 64.0 (57.0–72.0) | 67.0 (58.0–73.0) | 0.33 | |
BMI, kg/m2 | 23.5 (21.5–25.7) | 23.7 (21.4–26.3) | 0.67 | 23.5 (21.1–25.5) | 23.1 (21.3–26.3) | 0.82 | |
Pulmonary comorbidities | 58 (11.3) | 8 (10.0) | 0.73 | 16 (8.1) | 7 (9.6) | 0.70 | |
Diabetes mellitus | 65 (12.7) | 9 (11.3) | 0.72 | 32 (16.2) | 7 (9.6) | 0.17 | |
Anemia | 116 (22.6) | 13 (16.3) | 0.20 | 47 (23.9) | 12 (16.4) | 0.19 | |
Current smoker | 28 (5.5) | 3 (3.8) | 0.71 | 7 (3.6) | 3 (4.1) | >0.99 | |
Creatine, μmol/L | 66.0 (58.0–75.0) | 67.5 (60.0–77.0) | 0.31 | 67.0 (58.0–77.0) | 68.0 (60.0–77.0) | 0.71 | |
Albumin, g/L | 44.2 (41.7–46.1) | 44.1 (41.8–45.9) | 0.99 | 44.2 (41.4–46.2) | 44.2 (41.9–46.0) | 0.70 | |
ASA classification | 0.65 | 0.74 | |||||
I | 31 (6.0) | 3 (3.8) | 13 (6.6) | 3 (4.1) | |||
II | 445 (86.7) | 73 (91.3) | 170 (86.3) | 66 (90.4) | |||
III | 37 (7.2) | 4 (5.0) | 14 (7.1) | 4 (5.5) | |||
Pathology | >0.99 | 0.63 | |||||
SCC | 485 (94.5) | 77 (96.3) | 182 (92.4) | 70 (95.9) | |||
Adenocarcinoma | 23 (4.5) | 3 (3.8) | 12 (6.1) | 3 (4.1) | |||
Other malignancy | 5 (1.0) | 0 (0.0) | 3 (1.5) | 0 (0.0) | |||
Tumor location | 0.83 | 0.47 | |||||
Upper thoracic | 65 (12.7) | 12 (15.0) | 28 (14.2) | 11 (15.1) | |||
Middle thoracic | 237 (46.2) | 35 (43.8) | 97 (49.2) | 30 (41.1) | |||
Lower thoracic† | 211 (41.1) | 33 (41.3) | 72 (36.5) | 32 (43.8) |
Data shown are medians (interquartile range) or numbers (%). †, included gastroesophageal junction. TLV, two-lung ventilation; OLV, one-lung ventilation; BMI, body mass index; ASA, American Society of Anesthesiologists; SCC, squamous cell carcinoma.
Table 2
Variables | Before matching (n=593) | After matching (n=270) | |||||
---|---|---|---|---|---|---|---|
TLV group (n=513) | OLV group (n=80) | P value | TLV group (n=197) | OLV group (n=73) | P value | ||
Fluid intake, mL | 2,250.0 (2,000.0–2,500.0) |
2,350.0 (2,000.0–2,600.0) |
0.04* | 2,300.0 (2,000.0–2,625.0) |
2,300.0 (2,000.0–2,600.0) |
0.30 | |
Blood loss, mL | 100.0 (50.0–100.0) | 100.0 (62.5–100.0) | 0.04* | 100.0 (50.0–100.0) | 100.0 (100.0–100.0) | 0.24 | |
Urinary output, mL | 300.0 (200.0–500.0) | 400.0 (200.0–600.0) | 0.10 | 350.0 (200.0–500.0) | 400.0 (275.0–600.0) | 0.15 | |
Duration, min | |||||||
Surgery | 201.0 (185.0–227.0) | 229.5 (195.0–259.5) | <0.01* | 225.4±44.8 | 233.0±45.9 | 0.22 | |
Anesthesia | 250.0 (232.0–280.0) | 300.0 (250.0–327.0) | <0.01* | 278.1±50.1 | 297.0±50.2 | <0.01* |
Data shown are medians (interquartile range) or means ± standard deviation. *, significant. TLV, two-lung ventilation; OLV, one-lung ventilation.
Incidence of pneumonia within the first 3 days of surgery was higher in the TLV group (11.7% vs. 4.1%) but the difference was not significant (P=0.06; Figure 2). The incidence of radiographic infiltrate was 36.0% in the TLV group and 28.8% in the OLV group (P=0.26). Incidence of other major PPCs and major non-pulmonary postoperative complications did not differ significantly between the groups (Tables 3,4). Incidence of prolonged ICU stay (more than 2 days), was 5.6% in the TLV group and 2.7% in the OLV group (P=0.52). Length of hospital stay was significantly longer in the TLV group [13.0 days (IQR, 10.0–15.0 days) vs. 11.0 days (IQR, 9.0–15.0 days); P=0.03].
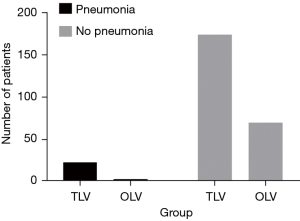
Table 3
PPCs | TLV group (n=197) | OLV group (n=73) | χ2 | P value |
---|---|---|---|---|
Pneumonia | 23 (11.7) | 3 (4.1) | 3.503 | 0.06 |
Pleural effusion | 13 (6.6) | 0 (0.0) | 3.723 | 0.05 |
Pneumothorax | 4 (2.0) | 0 (0.0) | – | 0.58 |
Atelectasis | 5 (2.5) | 0 (0.0) | – | 0.33 |
Respiratory failure | 7 (3.6) | 2 (2.7) | 0.000 | >0.99 |
ARDS | 1 (0.5) | 0 (0.0) | – | >0.99 |
Acute aspiration | 0 (0.0) | 1 (1.4) | – | 0.27 |
Tracheobronchial injury | 0 (0.0) | 0 (0.0) | – | – |
Re-intubation | 3 (1.5) | 1 (1.4) | – | >0.99 |
Tracheotomy | 3 (1.5) | 0 (0.0) | – | 0.57 |
Data shown are numbers (%). PPCs, postoperative pulmonary complications; TLV, two-lung ventilation; OLV, one-lung ventilation; ARDS, acute respiratory distress syndrome.
Table 4
Postoperative complication | TLV group (n=197) | OLV group (n=73) | χ2 | P value |
---|---|---|---|---|
Recurrent laryngeal nerve injury | 5 (2.5) | 1 (1.4) | – | >0.99 |
Anastomotic leak | 25 (12.7) | 7 (9.6) | 0.490 | 0.49 |
Chylothorax | 1 (0.5) | 1 (1.4) | – | 0.47 |
Wound infection | 3 (1.5) | 2 (2.7) | – | 0.62 |
Data shown are numbers (%). TLV, two-lung ventilation; OLV, one-lung ventilation.
Discussion
The main finding of this study was that TLV with CO2 pneumothorax did not reduce the incidence of early PPCs after MIE compared with OLV with bronchial blockade. Among the 270 matched patients, 26 patients overall developed pneumonia within the first 3 days of surgery (9.6%), which is comparable to the rate reported in previous studies (34-36). Although the incidence of pneumonia in the TLV and OLV groups was 11.7% and 4.1%, respectively, the difference was not significant. Additionally, the incidence of other PPCs requiring treatment did not significantly differ between the groups. These results are in line with several earlier studies, which also found no significant distinctions in terms of PPCs between individuals who underwent TLV or OLV for esophagectomy (8,9,23,37). Contrary to our results, in a recent meta-analysis, TLV was associated with decreased incidence of PPCs (13); however, the TLV group underwent thoracoscopy, whereas the OLV group underwent either thoracoscopy or thoracotomy, which might have contributed to the difference. In their subgroup analysis of patients who only underwent thoracoscopy, incidence of PPCs did not differ. Compared with DLET intubation, bronchial blocker use in thoracic surgery patients is associated with lower risks of respiratory infections and PPCs (18). However, in contrast to our study, most previous ones used a DLET for OLV. Only one retrospective study compared TLV and OLV with bronchial blockade for thoracoscopic esophagectomy (9); although PPCs were not its main focus, it reported no significant difference in incidence of PPCs between the different ventilation strategies.
The incidence rates of early postoperative pneumonia and pleural effusion requiring drainage in our study were higher in the TLV group, although the differences were not significant. However, the length of hospital stay was significantly longer in the TLV group (13.0 vs. 11.0 days), even though the two groups did not differ significantly in other major non-pulmonary complications. We speculate this may be related to the non-significant differences in PPCs between the TLV and OLV groups. Our results contrast with the theory that OLV acts as a risk factor for lung damage and may cause more lung damage because of higher lung volume and ventilation pressure in the ventilated lung and atelectasis and ischemia–reperfusion injury in the collapsed lung (12,38). We suppose that there are several reasons for the unexpected results. Although a low insufflation rate and a maximum pneumothorax pressure of 8 to 10 mmHg have been considered safe for TLV with artificial pneumothorax, a recent study has shown that intrathoracic pressure overshoot frequently occurs when TLV with pneumothorax is used for thoracoscopic surgery (39). Peak airway pressure increases during this overshoot and may result in stress injury to the pulmonary alveoli. Another retrospective study similarly showed that peak inspiratory pressure increases after artificial pneumothorax is induced (40). High airway pressure during mechanical ventilation damages endothelial cells, disrupts the pulmonary surfactant system, increases pulmonary permeability, and may raise the danger of ventilator-associated lung damage and PPCs (41,42). This could in part explain the higher incidence of infiltrates on chest radiography in the TLV group in our study (36.0% vs. 28.8%; P=0.26). Therefore, we speculate that pulmonary damage is related to increased airway pressure during TLV with artificial pneumothorax. The fact that tidal volume is typically set at a minimum tolerable value in the TLV group to achieve sufficient lung collapse to enable a workable surgical field is another potential explanation. At low lung volumes, repeated lung unit opening and closing may be harmful, especially if lung inflation is not uniform. Therefore, low lung volumes can cause lung damage, which has systemic, physiologic, structural, and biologic repercussions (43). Unfortunately, no studies have specifically examined these differences between TLV with artificial pneumothorax and OLV with bronchial blockade during mechanical ventilation. To better comprehend this issue, additional research is required, particularly prospective randomized controlled trials.
Researchers have demonstrated that TLV is advantageous for MIE because SLET intubation causes less airway injury than OLV. However, DLET intubation was employed in the OLV group in every pertinent previous study (11,13). Airway injury was not examined in the one study that compared perioperative outcomes between TLV and OLV with bronchial blockade (9). In our study, neither group had a patient with tracheobronchial damage, perhaps because all intubations were handled by a team of experienced thoracic anesthesiologists who were familiar with using a bronchial blocker. Studies have also shown that using a bronchial blocker causes significantly less airway injury than using a DLET (17-19). Therefore, we argue that airway injury is less of an issue in patients intubated using a bronchial blocker for OLV, especially in the hands of skilled anesthesiologists. However, we did notice a shorter anesthesia time in the TLV group, which is consistent with a prior study (11). This was not unexpected because all preparation was performed in the operating room and use of a bronchial blocker has been associated with longer intubation and tube localization times. After anesthesia induction, bronchial blocker positioning was aided using a bronchofiberscope in the supine position and re-checked after the patient was moved to the left lateral-prone position. Obviously, more time is required for intubation preparation and fiberoptic confirmation of a bronchial blocker. In addition, prolonged anesthesia duration may also be related to a higher malposition rate of bronchial blocker (44). Because of the study’s retrospective design, we could not collect data on this issue. Nevertheless, prolonged anesthesia time was not associated with a worse clinical outcome.
This study has several limitations. First, it was retrospective in design. Although we performed PSM analysis to account for confounding factors, bias still may have been present because of variations in clinical practice. Second, potentially crucial intraoperative data such as ventilator parameters (tidal volume, positive end-expiratory pressure, and peak airway pressure) were not included for analysis because of the retrospective design. These data may have an impact on PPCs. Nevertheless, the same team of thoracic anesthesiologists directed all anesthetic management, which ensured a certain level of uniformity and consistency. Third, certain technical details of the procedure (e.g., the number of the harvest lymph nodes along the RLN and details of the drainage procedure) were not collected. However, the incidence of RLN injury, which is more directly related to the occurrence of PPCs, was analyzed. Finally, even though the study included 593 patients, only 270 of them were included in the PSM analysis; this sample size may not be sufficient to detect certain trends. Large-scale randomized controlled trials are warranted.
Conclusions
Compared with OLV with bronchial blockade, TLV with CO2 pneumothorax did not reduce the incidence of early PPCs after MIE. Further investigation is needed to understand the underlying mechanism, particularly prospective randomized controlled trials.
Acknowledgments
We thank Liwen Bianji (Edanz) (http://www.liwenbianji.cn) for editing the language of a draft of this manuscript.
Funding: None.
Footnote
Reporting Checklist: The authors have completed the STROBE reporting checklist. Available at https://jtd.amegroups.com/article/view/10.21037/jtd-23-1667/rc
Data Sharing Statement: Available at https://jtd.amegroups.com/article/view/10.21037/jtd-23-1667/dss
Peer Review File: Available at https://jtd.amegroups.com/article/view/10.21037/jtd-23-1667/prf
Conflicts of Interest: All authors have completed the ICMJE uniform disclosure form (available at https://jtd.amegroups.com/article/view/10.21037/jtd-23-1667/coif). The authors have no conflicts of interest to declare.
Ethical Statement: The authors are accountable for all aspects of the work in ensuring that questions related to the accuracy or integrity of any part of the work are appropriately investigated and resolved. The study was conducted in accordance with the Declaration of Helsinki (as revised in 2013). The study was approved by the Institutional Review Board at Peking University Cancer Hospital (No. 2023YJZ36). The requirement for written informed consent was waived because of the study’s retrospective design.
Open Access Statement: This is an Open Access article distributed in accordance with the Creative Commons Attribution-NonCommercial-NoDerivs 4.0 International License (CC BY-NC-ND 4.0), which permits the non-commercial replication and distribution of the article with the strict proviso that no changes or edits are made and the original work is properly cited (including links to both the formal publication through the relevant DOI and the license). See: https://creativecommons.org/licenses/by-nc-nd/4.0/.
References
- Murphy G, McCormack V, Abedi-Ardekani B, et al. International cancer seminars: a focus on esophageal squamous cell carcinoma. Ann Oncol 2017;28:2086-93. [Crossref] [PubMed]
- Deana C, Vetrugno L, Stefani F, et al. Postoperative complications after minimally invasive esophagectomy in the prone position: any anesthesia-related factor? Tumori 2021;107:525-35. [Crossref] [PubMed]
- Ising MS, Smith SA, Trivedi JR, et al. Minimally Invasive Esophagectomy Is Associated with Superior Survival Compared to Open Surgery. Am Surg 2023;89:1833-43. [Crossref] [PubMed]
- Dyas AR, Stuart CM, Bronsert MR, et al. Minimally invasive surgery is associated with decreased postoperative complications after esophagectomy. J Thorac Cardiovasc Surg 2023;166:268-78. [Crossref] [PubMed]
- Yu WQ, Zhai LX, Shi GD, et al. Short-term outcome of totally minimally invasive versus hybrid minimally invasive Ivor-Lewis esophagectomy. Asian J Surg 2023;46:3727-33. [Crossref] [PubMed]
- Haagh TJ, Buise MP, Luyer MDP, et al. Two-lung ventilation during prone minimally invasive thorascopic oesophagectomy: A case series. Eur J Anaesthesiol 2019;36:307-9. [Crossref] [PubMed]
- Singh M, Uppal R, Chaudhary K, et al. Use of single-lumen tube for minimally invasive and hybrid esophagectomies with prone thoracoscopic dissection: case series. J Clin Anesth 2016;33:450-5. [Crossref] [PubMed]
- Lin M, Shen Y, Wang H, et al. A comparison between two lung ventilation with CO(2) artificial pneumothorax and one lung ventilation during thoracic phase of minimally invasive esophagectomy. J Thorac Dis 2018;10:1912-8. [Crossref] [PubMed]
- Cai L, Li Y, Sun L, et al. Better perioperative outcomes in thoracoscopic-esophagectomy with two-lung ventilation in semi-prone position. J Thorac Dis 2017;9:117-22. [Crossref] [PubMed]
- Daghmouri MA, Chaouch MA, Depret F, et al. Two-lung ventilation in video-assisted thoracoscopic esophagectomy in prone position: a systematic review. Anaesth Crit Care Pain Med 2022;41:101134. [Crossref] [PubMed]
- Cheng YL, Huang TW, Lee SC, et al. Video-assisted thoracoscopic surgery using single-lumen endotracheal tube anaesthesia in primary spontaneous pneumothorax. Respirology 2010;15:855-9. [Crossref] [PubMed]
- Deana C, Vetrugno L, Bignami E, et al. Peri-operative approach to esophagectomy: a narrative review from the anesthesiological standpoint. J Thorac Dis 2021;13:6037-51. [Crossref] [PubMed]
- Deng HY, Zhang Y, Ren Y, et al. Two-lung ventilation or one-lung ventilation for esophagectomy: maybe the more is better from the evidence of meta-analysis. Updates Surg 2022;74:1199-207. [Crossref] [PubMed]
- Morris BN, Fernando RJ, Garner CR, et al. A Randomized Comparison of Positional Stability: The EZ-Blocker Versus Left-Sided Double-Lumen Endobronchial Tubes in Adult Patients Undergoing Thoracic Surgery. J Cardiothorac Vasc Anesth 2021;35:2319-25. [Crossref] [PubMed]
- Zhang TH, Liu XQ, Cao LH, et al. A randomised comparison of the efficacy of a Coopdech bronchial blocker and a double-lumen endotracheal tube for minimally invasive esophagectomy. Transl Cancer Res 2020;9:4686-92. [Crossref] [PubMed]
- Lin M, Shen Y, Feng M, et al. Is two lung ventilation with artificial pneumothorax a better choice than one lung ventilation in minimally invasive esophagectomy? J Thorac Dis 2019;11:S707-12. [Crossref] [PubMed]
- Knoll H, Ziegeler S, Schreiber JU, et al. Airway injuries after one-lung ventilation: a comparison between double-lumen tube and endobronchial blocker: a randomized, prospective, controlled trial. Anesthesiology 2006;105:471-7. [Crossref] [PubMed]
- Liu W, Jin F, Wang HM, et al. The association between double-lumen tube versus bronchial blocker and postoperative pulmonary complications in patients after lung cancer surgery. Front Oncol 2022;12:1011849. [Crossref] [PubMed]
- Mourisse J, Liesveld J, Verhagen A, et al. Efficiency, efficacy, and safety of EZ-blocker compared with left-sided double-lumen tube for one-lung ventilation. Anesthesiology 2013;118:550-61. [Crossref] [PubMed]
- Tu DH, Qu R, Ping W, et al. Double-lumen tube versus bronchial blocker in lymphadenectomy along the left recurrent laryngeal nerve for esophageal cancer: a propensity-matched analysis. Langenbecks Arch Surg 2022;407:1891-900. [Crossref] [PubMed]
- Clayton-Smith A, Bennett K, Alston RP, et al. A Comparison of the Efficacy and Adverse Effects of Double-Lumen Endobronchial Tubes and Bronchial Blockers in Thoracic Surgery: A Systematic Review and Meta-analysis of Randomized Controlled Trials. J Cardiothorac Vasc Anesth 2015;29:955-66. [Crossref] [PubMed]
- Vanner R. Arndt Endobronchial Blocker during oesophagectomy. Anaesthesia 2005;60:295-6. [Crossref] [PubMed]
- Zhang R, Liu S, Sun H, et al. The application of single-lumen endotracheal tube anaesthesia with artificial pneumothorax in thoracolaparoscopic oesophagectomy. Interact Cardiovasc Thorac Surg 2014;19:308-10. [Crossref] [PubMed]
- Howells P, Thickett D, Knox C, et al. The impact of the acute respiratory distress syndrome on outcome after oesophagectomy. Br J Anaesth 2016;117:375-81. [Crossref] [PubMed]
- American Thoracic Society. Guidelines for the management of adults with hospital-acquired, ventilator-associated, and healthcare-associated pneumonia. Am J Respir Crit Care Med 2005;171:388-416. [Crossref] [PubMed]
- Low DE, Alderson D, Cecconello I, et al. International Consensus on Standardization of Data Collection for Complications Associated With Esophagectomy: Esophagectomy Complications Consensus Group (ECCG). Ann Surg 2015;262:286-94. [Crossref] [PubMed]
- Ohi M, Toiyama Y, Omura Y, et al. Risk factors and measures of pulmonary complications after thoracoscopic esophagectomy for esophageal cancer. Surg Today 2019;49:176-86. [Crossref] [PubMed]
- Griffin SM, Shaw IH, Dresner SM. Early complications after Ivor Lewis subtotal esophagectomy with two-field lymphadenectomy: risk factors and management. J Am Coll Surg 2002;194:285-97. [Crossref] [PubMed]
- Law S, Wong KH, Kwok KF, et al. Predictive factors for postoperative pulmonary complications and mortality after esophagectomy for cancer. Ann Surg 2004;240:791-800. [Crossref] [PubMed]
- Healy LA, Ryan AM, Gopinath B, et al. Impact of obesity on outcomes in the management of localized adenocarcinoma of the esophagus and esophagogastric junction. J Thorac Cardiovasc Surg 2007;134:1284-91. [Crossref] [PubMed]
- Uchihara T, Yoshida N, Baba Y, et al. Risk factors for pulmonary morbidities after minimally invasive esophagectomy for esophageal cancer. Surg Endosc 2018;32:2852-8. [Crossref] [PubMed]
- Nakamura M, Iwahashi M, Nakamori M, et al. An analysis of the factors contributing to a reduction in the incidence of pulmonary complications following an esophagectomy for esophageal cancer. Langenbecks Arch Surg 2008;393:127-33. [Crossref] [PubMed]
- Hikasa Y, Suzuki S, Mihara Y, et al. Intraoperative fluid therapy and postoperative complications during minimally invasive esophagectomy for esophageal cancer: a single-center retrospective study. J Anesth 2020;34:404-12. [Crossref] [PubMed]
- Low DE, Kuppusamy MK, Alderson D, et al. Benchmarking Complications Associated with Esophagectomy. Ann Surg 2019;269:291-8. [Crossref] [PubMed]
- Biere SS, van Berge Henegouwen MI, Maas KW, et al. Minimally invasive versus open oesophagectomy for patients with oesophageal cancer: a multicentre, open-label, randomised controlled trial. Lancet 2012;379:1887-92. [Crossref] [PubMed]
- Kuppusamy MK, Low DEInternational Esodata Study Group (IESG). Evaluation of International Contemporary Operative Outcomes and Management Trends Associated With Esophagectomy: A 4-Year Study of >6000 Patients Using ECCG Definitions and the Online Esodata Database. Ann Surg 2022;275:515-25. [Crossref] [PubMed]
- Nomura S, Tsujimoto H, Ishibashi Y, et al. Efficacy of artificial pneumothorax under two-lung ventilation in video-assisted thoracoscopic surgery for esophageal cancer. Surg Endosc 2020;34:5501-7. [Crossref] [PubMed]
- Shinozaki H, Matsuoka T, Ozawa S. Pharmacological treatment to reduce pulmonary morbidity after esophagectomy. Ann Gastroenterol Surg 2021;5:614-22. [Crossref] [PubMed]
- Ren Y, Zhu X, Yan H, et al. Cardiorespiratory impact of intrathoracic pressure overshoot during artificial carbon dioxide pneumothorax: a randomized controlled study. BMC Anesthesiol 2022;22:76. [Crossref] [PubMed]
- Tanigawa Y, Nakamura K, Yamashita T, et al. Changes in respiratory mechanics of artificial pneumothorax two-lung ventilation in video-assisted thoracoscopic esophagectomy in prone position. Sci Rep 2021;11:6978. [Crossref] [PubMed]
- Serpa Neto A, Juffermans NP, Hemmes SNT, et al. Interaction between peri-operative blood transfusion, tidal volume, airway pressure and postoperative ARDS: an individual patient data meta-analysis. Ann Transl Med 2018;6:23. [Crossref] [PubMed]
- Seeley E, McAuley DF, Eisner M, et al. Predictors of mortality in acute lung injury during the era of lung protective ventilation. Thorax 2008;63:994-8. [Crossref] [PubMed]
- Lohser J, Slinger P. Lung Injury After One-Lung Ventilation: A Review of the Pathophysiologic Mechanisms Affecting the Ventilated and the Collapsed Lung. Anesth Analg 2015;121:302-18. [Crossref] [PubMed]
- Langiano N, Fiorelli S, Deana C, et al. Airway management in anesthesia for thoracic surgery: a "real life" observational study. J Thorac Dis 2019;11:3257-69. [Crossref] [PubMed]