Gram-negative bacteria facilitate tumor outgrowth and metastasis by promoting lipid synthesis in lung cancer patients
Introduction
Lung cancer is the leading cause of cancer-related death worldwide, with an increasing incidence every year (1,2). Non-small cell lung cancer (NSCLC) is the most common type and accounts for about 85% of all cases in patients with lung cancer (3). In NSCLC patients, general outcomes of standard therapy including surgery, radiation therapy and chemotherapy are poor (3,4). Recently, personalized treatment of NSCLC patients using targeted therapeutics, which are dominantly based on patients’ and tumors’ precise genetic defects, served as a promising direction for NSCLC clinical management (5-7). However, patients who respond to targeted therapeutics first are frequently and quickly encountered with drug resistance. Thus, current clinical therapeutic options for NSCLC patients are still limited and their prognoses remain far from acceptable (8-10). Further studies on critical influential factors involved in outgrowth and metastasis of NSCLC cells, especially those focused on cancer cells from clinical patients, are urgently needed.
Pulmonary infections are very common and predict poor prognosis of NSCLC patients (11). Further studies pinpoint Gram-negative bacteria as the most frequent pathogen that led to increased mortality in NSCLC patients (12,13). Further, postoperative pneumonias are a common complication of thoracic surgery and predominantly caused by Gram-negative bacteria (14-16). These observations form the basis of an important subject of investigation, regarding the effects of Gram-negative bacteria on cancer development. Of interest, a recent study revealed a positive contributing effect of Gram-negative bacteria on metastasis of H59 and A549 lung cancer cell lines (17), which could partly explain the adverse effect of infectious complications on prognosis of NSCLC patients. However, potential roles of Gram-negative bacteria in NSCLC development are still not fully understood.
In this study, we explored the effect of Gram-negative bacteria on outgrowth and metastasis of NSCLC cells from clinical patients. NSCLC cells were isolated from the surgical tissues of clinical patients, cultured with Gram-negative bacteria and observed for their growth and invasive potentials in vitro and in vivo. Our findings demonstrated an efficient ability of Gram-negative bacteria to enhance outgrowth and metastasis of human lung cancer cells by promoting lipid synthesis through TLR4 and TLR9 signaling. These findings enlarge our knowledgebase on the effects of infectious complications on NSCLC pathogenesis and thus provide clues for developing therapeutic strategies in future clinical practice.
Methods
Patients
Fifty patients with NSCLC were enrolled in this study. Diagnosis of NSCLC was confirmed by pathology. Subjects with autoimmune inflammatory diseases were excluded. The median age of NSCLC patients was 65.8 years old and 72% were males. All subjects were given written informed consent before collecting surgical tissues and clinical parameters. All procedures performed in this study, which involved human participants, were in accordance with the ethical standards of our institutional research committee and with the 1964 Helsinki declaration and its later amendments with an approval from Tongji Institutional Ethics Committee.
Mice
Female BALB/c nude mice of 6 weeks old were obtained from the Shanghai Laboratory Animal Center (CAS) and housed under specific pathogen-free conditions. All procedures performed in studies involving animals were in accordance with the ethical standards of Tongji Institutional Ethics Committee.
Cell culture and reagents
NSCLC cells were isolated from surgical tissues with Clonogenic Tumor Cell Isolation Kit (Cell Biolabs) according to the manual’s instructions. Escherichia coli (E. coli, ATCC® 11775™) were inactivated by boiling at 95 °C for 15min and used as previously described (17). NSCLC cells were cultured in complete RPMI 1640 medium containing 10% heat-inactivated fetal bovine serum (Gibco) supplemented with 2 mM glutamine, 100 IU/mL penicillin and 100 mg/mL streptomycin sulfate at 37 °C under 5% CO2. Human TLR4 and TLR9 shRNAs, LPS-RS, ODN TTAGGG and control were purchased from Invivogen. Nucleofector™ Kit was obtained from Lonza. Anti-Fatty Acid Synthase (FAS) antibody was from Abcam. BODIPY Fluorophores, human ACC1 siRNA and control were from Thermo Fisher Scientific. C75, a synthetic inhibitor of FAS, and lipopolysaccharide (LPS) were from Sigma. Reagents were used according to manufactures’ instructions.
Real-time polymerase chain reaction (PCR)
Total RNA was extracted using Ambion® Isolation Kit (Thermo Fisher). cDNA was synthesized with First-Strand cDNA Synthesis Kit (Thermo Fisher). qPCR was performed using SYBR Green Master Mixes (Thermo Fisher). Primers were obtained from Applied Biosystems and 18S rRNA was used as an internal control.
MTT assay
Tumor growth in vitro was detected by analyzing proliferative expansion of human NSCLC cells with MTT assay. Briefly, NSCLC cells were seeded at 5×103 cells each well and incubated with or without inactivated E. coli in 96-well plates and measured with MTT cell proliferation kit (Cayman Chemical) according to the manual’s instructions.
Invasive assay
Tumor invasive assay in vitro was performed with Biocoat Matrigel Invasion Chamber (8 µm, BD Bioscience) (18). Briefly, 5×104 NSCLC cells were suspended in 0.5 mL of serum-free media and seeded onto the upper chamber of Matrigel-coated filters. In lower chambers, 0.75 mL of complete medium was added as a chemoattractant. After 24 h, cells on the upper side of the chamber were scraped, and the ones on the lower side of the chamber were fixed, stained and invaded cells were counted under the microscope. Five predetermined fields were counted for each membrane and the mean values were used. Invasive potentials were expressed as the percentage of invasion through the Matrigel Matrix and membrane relative to the migration through the control membrane.
Tumor growth in vivo
Tumor growth in vivo was assessed as previously described (19). Briefly, groups of nude mice (n=5 per group) were subcutaneously injected with 5×106 human NSCLC cells suspended in 200 µL of PBS. For some experiments, NSCLC cells were treated with inactivated E. coli for 24 h before injection. The size of the tumor was measured at days 3, 6 and 9 after implantation by digital calipers.
Tumor metastasis in vivo
Tumor metastasis in vivo was determined as previously described (18,20). Briefly, nude mice (n=5 per group) were intratracheally challenged with LPS (10 µg/mouse), and injected with 5×105 NSCLC cells via tail vein 6 h later. For some experiments, NSCLC cells were pretreated with inactivated E. coli for 24 h before injection. Two weeks later, nude mice were detected for their lung tumor metastases/burdens that were reflected by total lung weights.
Flow cytometry
For FAS and ACC1 analysis, 0.5 million NSCLC cells were fixed with BD fix buffer I for 10 min at 37 °C and permeabilized with BD Perm Buffer III for 30 min on ice, followed by staining with rabbit anti-human FAS antibody (Abcam) or rabbit anti-human ACC1 antibody (Abcam) respectively for 45 min at 4 °C and Alexa Fluor® 488 goat anti-rabbit IgG (Abcam) for 30 min at 4 °C. Rabbit monoclonal IgG was used as isotype control. For detection of lipids, 0.5 million NSCLC cells were stained with BODIPY Fluorophores according to manufactures’ instructions. Cells were analyzed on a FACSCalibur flow cytometer (BD). Collected data were analyzed with FlowJo software (Tree Star).
Statistical analyses
Data were expressed as mean ± SD. T tests and Pearson correlation were used for statistical analyses using the program PRISM 6.0 (GraphPad Software). A value of P<0.05 was considered statistically significant.
Results
Gram-negative bacteria promote outgrowth and metastasis of NSCLC cells
To evaluate the effect of Gram-negative bacteria on outgrowth of NSCLC cells in clinical patients, tumor cells were isolated from surgical tissues in NSCLC patients, incubated with inactivated E. coli and analyzed for their proliferative growth using MTT assay. Results showed that E. coli efficiently promoted the growth capacity of NSCLC cells in dose and time dependent manner (Figure 1A,B). Further, invasive assay revealed that E. coli was able to enhance the invasive potential of NSCLC cells in a dose dependent manner (Figure 1C).
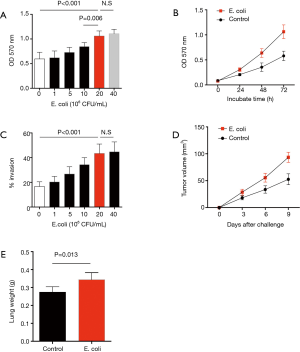
To confirm these phenomena in vivo, NSCLC cells were pretreated with inactivated E. coli and then injected into immune-deficient mice. Compared with untreated NSCLC cells, pretreatment with inactivated E. coli resulted in increased tumor size and lung weight in tumor-bearing mice, reflecting an robust outgrowth and metastasis of NSCLC cells in vivo (Figure 1D,E).
Gram-negative bacteria induce aberrant lipid synthesis in NSCLC cells
Aberrant lipid synthesis is believed to be an important metabolic feature for tumor cells and crucial for cancer development (21,22). To explore the possible mechanisms underling the effect of Gram-negative bacteria on NSCLC development, potential roles of Gram-negative bacteria in lipid synthesis were analyzed in NSCLC cells. Surprisingly, E. coli efficiently up-regulated the expression of FASN gene, which encodes FAS as a multifunctional and key enzyme to catalyze lipid synthesis. The FASN mRNA expression and FAS protein level were both substantially increased in NSCLC cells in response to E. coli (Figure 2A,B,C). In consistent, overexpression of ACC1, the first committed step of fatty acid synthesis, was also observed in NSCLC cells in response to E. coli (Figure 2D,E,F).
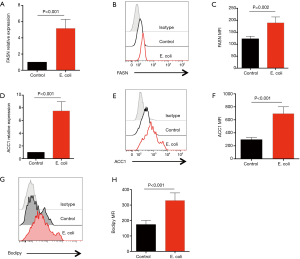
To directly detect lipids levels, NSCLC cells were stimulated with or without Gram-negative bacteria and stained with BODIPY Fluorophores. We found that lipids in NSCLC cells were significantly increased in response to E. coli (Figure 2G,H).
Inhibition of lipid synthesis abrogates Gram-negative bacteria-mediated NSCLC development
To determine whether increased lipid synthesis was required for Gram-negative bacteria to promote NSCLC outgrowth and metastasis, NSCLC cells were incubated with inactivated E. coli in the presence or absence of C75, an inhibitor of FAS, and detected for their growth and metastasis. Results showed that administration of C75 efficiently reduced the lipids in NSCLC cells (Figure 3A,B). Of note, C75 dramatically inhibited E. coli mediated outgrowth and metastasis of NSCLC cells in vitro (Figure 3C,D). Further, treatment with C75 abrogated E. coli enhanced growth and metastasis of NSCLC cells in vivo (Figure 3E,F).
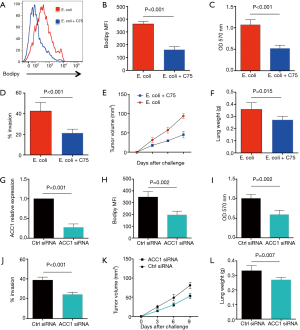
To confirm above findings, NSCLC were transfected with ACC1 siRNA, stimulated with Gram-negative bacteria and detected for outgrowth and metastasis. We found that transfection with ACC1 siRNA effectively reduced ACC1 mRNA and protein expressions in NSCLC cells (Figure 3G, Figure S1). Further, knockdown of ACC1 resulted in limited lipids in NSCLC cells (Figure 3H), and significantly abrogated NSCLC outgrowth and metastasis in vitro and in vivo (Figure 3I,J,K,L).
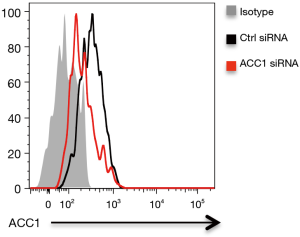
Gram-negative bacteria promotes lipid synthesis through TLR4 and TLR9 signaling in NSCLC cells
Recent evidence implicated TLR4 and TLR9 as functional pattern-recognition receptors in recognizing Gram-negative bacteria (17,23). Of interest, TLR4 and TLR9 were readily detectable in NSCLC cells and involved in NSCLC pathogenesis (24-26). Thus, we characterized the possible role of TLR4 and TLR9 in Gram-negative bacteria enhanced lipid synthesis. Knockdown of TLR4 expression in NSCLC cells by transfection with TLR4 shRNA effectively reduced mRNA and protein expressions of TLR4 (Figure 4A, Figure S2A), and essentially abrogated aberrant lipid synthesis in response to E. coli (Figure 4B,C). Interference with TLR9 expression by TLR9 shRNA also significantly decreased TLR9 expression and limited E. coli enhanced lipid synthesis in NSCLC cells (Figure 4D,E,F, Figure S2B). Lastly, double knockdown of TLR4 and TLR9 had synergistic effect and decreased the lipid synthesis further in NSCLC cells (Figure 4G).
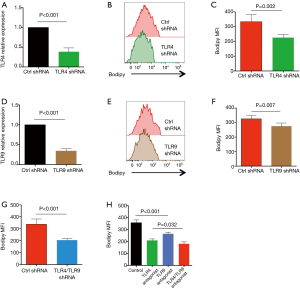
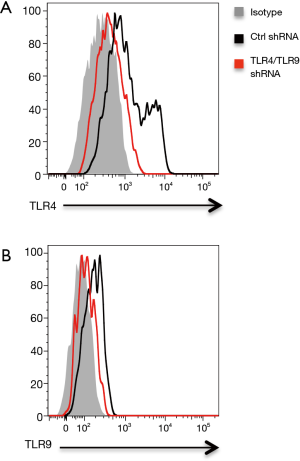
To confirm above phenomena, NSCLC cells were treated with LPS from rhodobacter sphaeroides (LPS-RS) as a TLR4 antagonist and ODN TTAGGG as a TLR9 antagonist, followed by stimulation with Gram-negative bacteria and detection of lipids level. Similar results were observed, that inhibition of TLR4 and TLR9 efficiently impeded aberrant lipid synthesis within NSCLC cells in response to Gram-negative bacteria (Figure 4H). In consistent, administration of inhibitory peptide against MyD88, a critical adaptor protein in TLR4/TLR9 signaling, efficiently alleviated the increased lipogenesis in NSCLC cells (Figure S3).
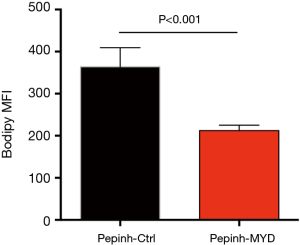
Interference with TLR4 and TLR9 signaling limits Gram-negative bacteria mediated NSCLC development
Given the crucial role of TLR4 and TLR9 in Gram-negative bacteria enhanced lipid synthesis, it is reasonable to hypothesize that TLR4 and TLR9 were responsible for Gram-negative bacteria mediated NSCLC development. Thus, NSCLC cells were transfected with TLR4 shRNA and TLR9 shRNA, stimulated with Gram-negative bacteria and analyzed for outgrowth and metastasis. Results showed that knockdown of TLR4 and TLR9 significantly limited E. coli-mediated outgrowth and metastasis of NSCLC cells in vitro and in vivo (Figure 5A,B,C,D). TLR4 and TLR9 appeared to have synergistic effect, as double knockdown of both of these receptors reduced NSCLC outgrowth and metastasis even further (Figure 5A,B,C,D). We confirmed these results by using LPS-RS and ODN TTAGGG as TLR4 and TLR9 antagonist respectively, and consistent results were obtained (Figure 5E,F,G,H).
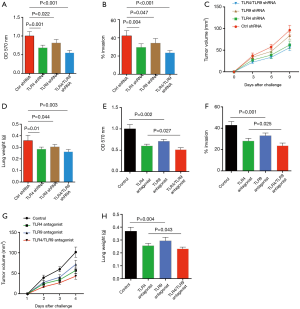
Higher expressions of TLR4 and TLR9 are associated with aberrant lipid synthesis in NSCLC patients with Gram-negative infection
To investigate the in vivo relevance of above findings in clinical patients, 20 NSCLC patients with Gram-negative infection and 16 NSCLC patients without Gram-negative infection were assayed for TLR4 and TLR9 expressions. Results showed that expressions of TLR4 and TLR9 were higher in patients with Gram-negative infection (Figure 6A,B). Further analysis revealed that both TLR4 and TLR9 expressions were closely correlated with FASN expression and lipids level in NSCLC patients with Gram-negative infection (Figure 6C,D,E,F). In addition, aberrant lipid synthesis in NSCLC cells was associated with their differentiation state (Figure 6G,H). Specifically, lipid synthesis was elevated in NSCLC cells with poor differentiation state and remained at lower levels in well-differentiated ones.
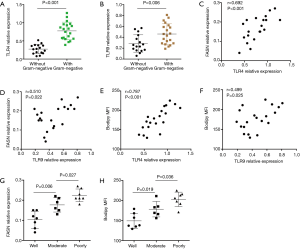
Discussion
Herein, we demonstrated that Gram-negative bacteria promoted outgrowth and metastasis of human lung cancer cells. Mechanistically, Gram-negative bacteria activated TLR4 and TLR9 signaling within NSCLC cells, which in turn led to increased lipid synthesis. In NSCLC patients, higher expressions of TLR4 and TLR9 were observed in those with Gram-negative infections and associated with their aberrant lipid synthesis. Collectively, NSCLC cells appeared competent and actively in sensing Gram-negative bacteria through TLR4 and TLR9 receptors, which fuel their aberrant metabolic features to promote tumor outgrowth and metastasis.
To our knowledge, this is the first study that investigates direct effect of Gram-negative bacteria on NSCLC outgrowth and metastasis in clinical patients. TLR4 and TLR9 are functionally expressed in NSCLC cells and crucial for responsiveness to Gram-negative bacteria in clinical patients. Consistent with our findings, a previous study showed that Gram-negative bacteria could increase the metastatic potential of lung cancer cell lines H59 and A549 via TLR4 activation (17). In fact, Toll like receptors have long been an active subject of investigation in tumor immunity and are well documented in lung cancer development (27-30). These results provide strong evidence and reveal a pro-proliferative and pro-metastatic effect of pulmonary infection on NSCLC development in patients. Thus, pulmonary infections, in particular with Gram-negative bacteria, require early diagnosis and prompt treatment for favorable outcomes in NSCLC patients.
Enhanced glucose uptake and use of aerobic glycolysis are conventionally considered as the most prominent metabolic alterations in cancer (22,31-33). However, increasing attention has been given to aberrant lipid synthesis and their regulation in cancer patients (22). Increased lipid synthesis is a prerequisite for rapid proliferation and metastasis of cancer cells (22,34-36). Herein, increased lipid synthesis in response to Gram-negative bacteria, to our knowledge, was a newly discovered mechanism in pulmonary-infection-mediated NSCLC development. Compared with well-differentiated uninfected NSCLC cells, lipogenensis was increased in well-differentiated Gram-negative-infected NSCLC cells (Figure S4). Stimulation of uninfected NSCLC cells with Gram-negative bacteria resulted in increased expressions of TLR4/TLR9 and aberrant lipogenesis (Figure S5), reinforcing a contributing effect of Gram-negative bacteria on aberrant lipid synthesis. However, we did not observe a higher lipogenesis in well-differentiated infected NSCLC cells than poorly differentiated uninfected NSCLC cells (Figure S4), suggesting aberrant lipogenesis as an intrinsic mechanism for cancer cells. In contrast, pulmonary infections with Gram-negative bacteria functions by fuelling the cancer metabolism for NSCLC cell to proliferate and metastasize. Accordingly, inhibition of lipid synthesis lead to limited proliferative and metastatic capacity of NSCLC cells. Aberrant lipid synthesis in cancer cells of lung cancer patients might be, at least partly, a consequence of pulmonary infections with Gram-negative bacteria. Thus, targeting aberrant lipid synthesis is a bio-functional therapeutic strategy to restrict both pro-cancer effect of Gram-negative bacteria and NSCLC development for clinical patients with pulmonary infections.
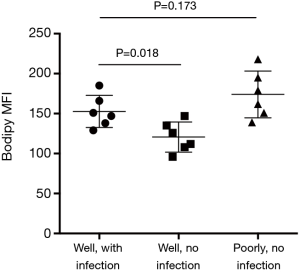
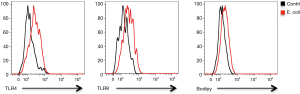
In essence, pulmonary infections with Gram-negative bacteria lead to aberrant lipid synthesis through TLR4 and TLR9 signaling in NSCLC patients and result in rapid proliferation and metastasis of NSCLC cells. These findings are obtained from NSCLC cells isolated from surgical tissues and closely reflect an in vivo relevance. Targeting these key molecules and pathways could greatly aid to treatment for lung cancer patients with pulmonary infections in clinical practice.
Acknowledgements
We would like to thank Professor Chunxue Bai from Zhongshan Hospital of Fudan University for valuable discussions and assistance.
Funding: This work was supported by grants from National Natural Science Foundation of China (81372347, 81402555) and Key Disciplines in Pudong New District of Shanghai City (PWZx2014-10).
Footnote
Conflicts of Interest: The authors have no conflicts of interest to declare.
Ethical Statement: The study was approved by our institutional research committee and in accordance with the 1964 Helsinki declaration and its later amendments with an approval from Tongji Institutional Ethics Committee. All subjects were given written informed consent before collecting surgical tissues and clinical parameters.
References
- Torre LA, Siegel RL, Jemal A. Lung Cancer Statistics. Adv Exp Med Biol 2016;893:1-19. [Crossref] [PubMed]
- Islami F, Torre LA, Jemal A. Global trends of lung cancer mortality and smoking prevalence. Transl Lung Cancer Res 2015;4:327-38. [PubMed]
- Molina JR, Yang P, Cassivi SD, et al. Non-small cell lung cancer: epidemiology, risk factors, treatment, and survivorship. Mayo Clin Proc 2008;83:584-94. [Crossref] [PubMed]
- Liu J, Dong M, Sun X, et al. Prognostic Value of 18F-FDG PET/CT in Surgical Non-Small Cell Lung Cancer: A Meta-Analysis. PLoS One 2016;11:e0146195. [Crossref] [PubMed]
- Ulivi P, Zoli W, Capelli L, et al. Target therapy in NSCLC patients: Relevant clinical agents and tumour molecular characterisation. Mol Clin Oncol 2013;1:575-81. [PubMed]
- Gridelli C, Peters S, Sgambato A, et al. ALK inhibitors in the treatment of advanced NSCLC. Cancer Treat Rev 2014;40:300-6. [Crossref] [PubMed]
- Pirker R. Third-generation epidermal growth factor receptor tyrosine kinase inhibitors in advanced nonsmall cell lung cancer. Curr Opin Oncol 2016;28:115-21. [Crossref] [PubMed]
- Xu M, Xie Y, Ni S, et al. The latest therapeutic strategies after resistance to first generation epidermal growth factor receptor tyrosine kinase inhibitors (EGFR TKIs) in patients with non-small cell lung cancer (NSCLC). Ann Transl Med 2015;3:96. [PubMed]
- Planchard D, Loriot Y, André F, et al. EGFR-independent mechanisms of acquired resistance to AZD9291 in EGFR T790M-positive NSCLC patients. Ann Oncol 2015;26:2073-8. [Crossref] [PubMed]
- Isozaki H, Ichihara E, Takigawa N, et al. Non-Small Cell Lung Cancer Cells Acquire Resistance to the ALK Inhibitor Alectinib by Activating Alternative Receptor Tyrosine Kinases. Cancer Res 2016;76:1506-16. [Crossref] [PubMed]
- Akinosoglou KS, Karkoulias K, Marangos M. Infectious complications in patients with lung cancer. Eur Rev Med Pharmacol Sci 2013;17:8-18. [PubMed]
- Sarihan S, Ercan I, Saran A, et al. Evaluation of infections in non-small cell lung cancer patients treated with radiotherapy. Cancer Detect Prev 2005;29:181-8. [Crossref] [PubMed]
- Qiao D, Wang Z, Lu Y, et al. A retrospective study of risk and prognostic factors in relation to lower respiratory tract infection in elderly lung cancer patients. Am J Cancer Res 2014;5:423-32. [PubMed]
- Seok Y, Lee E, Cho S. Respiratory complications during mid- and long-term follow-up periods in patients who underwent pneumonectomy for non-small cell lung cancer. Ann Thorac Cardiovasc Surg 2013;19:335-40. [Crossref] [PubMed]
- Mei J, Liu L, Tang M, et al. Airway bacterial colonization in patients with non-small cell lung cancer and the alterations during the perioperative period. J Thorac Dis 2014;6:1200-8. [PubMed]
- Andalib A, Ramana-Kumar AV, Bartlett G, et al. Influence of postoperative infectious complications on long-term survival of lung cancer patients: a population-based cohort study. J Thorac Oncol 2013;8:554-61. [Crossref] [PubMed]
- Chow SC, Gowing SD, Cools-Lartigue JJ, et al. Gram negative bacteria increase non-small cell lung cancer metastasis via Toll-like receptor 4 activation and mitogen-activated protein kinase phosphorylation. Int J Cancer 2015;136:1341-50. [Crossref] [PubMed]
- Li Q, Han Y, Wang C, et al. MicroRNA-125b promotes tumor metastasis through targeting tumor protein 53-induced nuclear protein 1 in patients with non-small-cell lung cancer. Cancer Cell Int 2015;15:84. [Crossref] [PubMed]
- Hattar K, Savai R, Subtil FS, et al. Endotoxin induces proliferation of NSCLC in vitro and in vivo: role of COX-2 and EGFR activation. Cancer Immunol Immunother 2013;62:309-20. [Crossref] [PubMed]
- Yan L, Cai Q, Xu Y. The ubiquitin-CXCR4 axis plays an important role in acute lung infection-enhanced lung tumor metastasis. Clin Cancer Res 2013;19:4706-16. [Crossref] [PubMed]
- Daniëls VW, Smans K, Royaux I, et al. Cancer cells differentially activate and thrive on de novo lipid synthesis pathways in a low-lipid environment. PLoS One 2014;9:e106913. [Crossref] [PubMed]
- Baenke F, Peck B, Miess H, et al. Hooked on fat: the role of lipid synthesis in cancer metabolism and tumour development. Dis Model Mech 2013;6:1353-63. [Crossref] [PubMed]
- Bhan U, Ballinger MN, Zeng X, et al. Cooperative interactions between TLR4 and TLR9 regulate interleukin 23 and 17 production in a murine model of gram negative bacterial pneumonia. PLoS One 2010;5:e9896. [Crossref] [PubMed]
- Liu X, Pei C, Yan S, et al. NADPH oxidase 1-dependent ROS is crucial for TLR4 signaling to promote tumor metastasis of non-small cell lung cancer. Tumour Biol 2015;36:1493-502. [Crossref] [PubMed]
- Li D, Jin Y, Sun Y, et al. Knockdown of toll-like receptor 4 inhibits human NSCLC cancer cell growth and inflammatory cytokine secretion in vitro and in vivo. Int J Oncol 2014;45:813-21. [PubMed]
- Xu L, Wen Z, Zhou Y, et al. MicroRNA-7-regulated TLR9 signaling-enhanced growth and metastatic potential of human lung cancer cells by altering the phosphoinositide-3-kinase, regulatory subunit 3/Akt pathway. Mol Biol Cell 2013;24:42-55. [Crossref] [PubMed]
- Pinto A, Morello S, Sorrentino R. Lung cancer and Toll-like receptors. Cancer Immunol Immunother 2011;60:1211-20. [Crossref] [PubMed]
- Zhan Z, Xie X, Cao H, et al. Autophagy facilitates TLR4- and TLR3-triggered migration and invasion of lung cancer cells through the promotion of TRAF6 ubiquitination. Autophagy 2014;10:257-68. [Crossref] [PubMed]
- Basith S, Manavalan B, Yoo TH, et al. Roles of toll-like receptors in cancer: a double-edged sword for defense and offense. Arch Pharm Res 2012;35:1297-316. [Crossref] [PubMed]
- Yang LS, Wu WS, Zhang F, et al. Role of toll-like receptors in lung cancer. J Recept Signal Transduct Res 2014;34:342-4. [Crossref] [PubMed]
- Lu J, Tan M, Cai Q. The Warburg effect in tumor progression: mitochondrial oxidative metabolism as an anti-metastasis mechanism. Cancer Lett 2015;356:156-64. [Crossref] [PubMed]
- Hammoudi N, Ahmed KB, Garcia-Prieto C, et al. Metabolic alterations in cancer cells and therapeutic implications. Chin J Cancer 2011;30:508-25. [Crossref] [PubMed]
- Zhang Y, Yang JM. Altered energy metabolism in cancer: a unique opportunity for therapeutic intervention. Cancer Biol Ther 2013;14:81-9. [Crossref] [PubMed]
- Santos CR, Schulze A. Lipid metabolism in cancer. FEBS J 2012;279:2610-23. [Crossref] [PubMed]
- Huang C, Freter C. Lipid metabolism, apoptosis and cancer therapy. Int J Mol Sci 2015;16:924-49. [Crossref] [PubMed]
- Phan LM, Yeung SC, Lee MH. Cancer metabolic reprogramming: importance, main features, and potentials for precise targeted anti-cancer therapies. Cancer Biol Med 2014;11:1-19. [PubMed]